Open Access
ARTICLE
The antioxidant trolox inhibits aging and enhances prostaglandin E-2 secretion in mesenchymal stem cells
1 Laboratory of Molecular Diagnosis and Regenerative Medicine, Medical Research Center, The Affiliated Hospital of Qingdao University, Qingdao, 266000, China
2 Department of Hematology, The Affiliated Hospital of Qingdao University, Qingdao, 266000, China
3 School of Nursing, Jilin University, Changchun, 130000, China
4 Yanda Medical Research Institute, Hebei Yanda Hospital, Sanhe, 065201, China
* Corresponding Authors: WEI WANG. Email: ; LISHENG WANG. Email:
(This article belongs to the Special Issue: Expert Opinions and Future Trends on Stem Cells, Biomaterials and Growth Factors)
BIOCELL 2023, 47(2), 385-392. https://doi.org/10.32604/biocell.2023.025203
Received 27 June 2022; Accepted 30 August 2022; Issue published 18 November 2022
Abstract
Mesenchymal stem cells (MSCs) have been widely used in regenerative medicine and clinical therapy due to their capabilities of proliferation, differentiation, and immune regulation. However, during in vitro expansion, MSCs are prone to aging, which largely limits their application. Prostaglandin E-2 (PGE-2) is a key effector secreted by MSCs to exert immunomodulatory effects. By screening the compound library for PGE-2 secretion, the antioxidant trolox was verified as a stimulator of MSCs to secrete PGE-2. The effect of antioxidant trolox on biological characteristics of MSCS, including aging, proliferation, and gene expression, was examined. The results demonstrated that trolox can resist aging, promote proliferation, and enhance PGE-2 secretion of MSCs without affecting their surface marker expression. Furthermore, trolox treatment up-regulates miR-17-92 clusters in MSCs and may contribute to its anti-aging effects. Thus, trolox addition might be beneficial for MSCs expansion and their application.Keywords
Mesenchymal stem cells have become a research hot spot in the field of regenerative medicine and clinical therapy due to the characteristics of self-renewal and multi-directional differentiation (Odabas et al., 2014), low immunogenicity (Nekanti et al., 2010), easy separation and acquisition (Krull et al., 2021), etc. However, the regenerative ability of MSCs gradually decreases with the passages in expansion culture (Yueh-Hsun et al., 2018), which limits their therapeutic efficiency in clinical application. Therefore, the strategies to suppress the aging of MSCs and enhance their immunomodulatory ability have attracted much attention for their application. The immunomodulatory ability of MSCs is dependent on their secretion of cytokines and biological substances (Prockop and Oh, 2012). Prostaglandin E-2 (PGE-2) is the major prostaglandin generated by cyclooxygenase enzymes and has been implicated in tissue regeneration such as activation of endogenous stem cells, immune regulation, and angiogenesis (Verma et al., 2022). Previous studies (Aggarwal and Pittenger, 2005) have demonstrated that PGE-2 acts as the major effector in regulating the immune activities of MSCs. The feline adipose tissue-derived MSCs alleviate dextran sodium sulfate-induced colitis mediated by PGE-2 secretion (An et al., 2018). PGE-2 has also been implicated as a key component of the MSC secretome (Yang et al., 2016). Preconditioning of MSCs strengthens the capacity of MSCs to regulate features of immunosuppression and tissue regeneration. Thus, PGE-2 secretion is considered as an immune ability and activated ability of MSCs. However, PGE-2 secretion gradually decreases with the expansion of MSCs generations in vitro culture (Zhang et al., 2019). In this study, we screened the bioactive molecules that can specifically enhance the secretion of a PGE-2 of MSCs and identified trolox (6-hydroxy-2,5,7,8-tetramethylchroman-2-carboxylic acid) as an inhibitor of MSC aging in long-term culture. Trolox is a water-soluble derivative of vitamin E, similar to α-tocopherol. It is a chain-breaking antioxidant that provides hydrogen from the hydroxyl group to peroxy radicals, thus terminating the chain reaction (Rezk et al., 2004). In this study, the effect of trolox on biological characteristics and aging of MSCs is further investigated.
Fetal bovine serum (FBS), α-minimal essential medium (MEM), and 0.25% trypsin-ethylenediaminetetraacetic acid were purchased from Gibco (USA). CCK8 Enhanced Solution was purchased from Meilunbio (Dalian, China), β-Galactase Kit was from Solarbio (Beijing, China), Human PGE-2 ELISA Kit was from Shanghai Guangrui Bio (Shanghai, China), Flow Cytometry Kit was from Biolegend (Beijing, China), Antioxidant Enzyme Typing Colorimetric Kit was from ElabScience (Wuhan, China), and the Compound trolox was from APEXBIO (USA). All primers used for quantitative polymerase chain reaction (qPCR) in this study were synthesized by Sangon Biotech (Shanghai, China).
Mesenchymal stem cells culture
Human umbilical cord-derived MSCs (UC-MSCs) were provided by the Allcare Biomedical Development Co., Ltd. (Qingdao, China). The UC-MSCs were cultured with α-MEM culture medium supplemented with 10% fetal bovine serum and 1 × double antibody (penicillin and streptomycin) on the Petri dish. These cells were cultured at 37°C in 5% CO2. After 2–3 days, when cell adherent fusion reached 80%, they were digested with 0.05% trypsin, subcultured, and expanded.
Enzyme-linked immunoabsorbent assay (ELISA) for PGE-2 detection
The supernatants of MSCs were collected, and concentrations of PGE-2 were measured according to the instructions of the PGE-2 ELISA kit. The OD value of each sample was measured at 450 nm by a microplate reader. A standard curve was established according to the concentration and its corresponding OD value. The concentration of PGE-2 was calculated according to the standard curve.
CCK-8 assay for detection of mesenchymal stem cell proliferation
MSCs were plated into 48-well plates at the density of 1 × 104/well. The concentration of trolox was set to 0, 0.5, 1, 2, 5, and 10 μM, respectively, and three multiple wells were set for each concentration. The CCK-8 reagent was added to the culture medium after MSCs adhered to the wall, incubating for 2 h, and then the OD was measured at 450 nm using a microplate reader. The increase in the rate of MSCs was calculated based on the measured values.
Flow cytometry for detection of mesenchymal stem cell surface markers
The P6 MSCs were divided into an experimental group and a control group. The experimental group was continuously treated with 5 μM trolox and transferred from P6 to P18. The expression of surface markers CD34, CD45, CD73, CD90, and CD105 was detected by flow cytometry.
β-Galactosidase staining for detection of aging of mesenchymal stem cells
MSCs in the control group and the trolox group were stained according to the instructions of the β-Galactosidase kit. These cells were cultured overnight at 37°C and then six fields were randomly selected to be visualized under light microscopy for observation. The positive cells were counted, and the aging cell percentage was calculated according to the number of positive cells.
Real-time quantitative polymerase chain reaction for detection of miR-17-92 clusters, peroxiredoxins (prdx) families, and glutathione peroxidase 4 (gpx4) gene expression
After the MSCs were treated with trolox for different periods, RNA was extracted by the Trizol method. After the concentration was determined, it was reverse-transcribed into cDNA. SYBRGREEN kit was used for quantitative PCR detection. The PCR reaction conditions included 40 cycles of denaturation at 95°C for 20 min, annealing at 95°C for 30 s, and extension at 60°C for 1 min. The final result was analyzed using the 2−∆∆CT method. The primer sequences are shown in Table 1.
The activity assay for superoxide dismutase (SOD), glutathione peroxidase (GSH-PX), and catalase (CAT) in the culture supernatant of mesenchymal stem cells
P6 MSCs were seeded in 6-well plates and divided into the experimental group and control group. The cells in the experimental group were continuously cultured in the absence (control group) or presence of 5 μM trolox (experimental group) until P12 passages. After P12 MSCs were allowed to adhere for 24 h, the culture medium was changed to that without trolox and cultured for another 24 h, the supernatant was collected, and the activity level of antioxidant enzymes in the supernatant of mesenchymal stem cells culture was detected according to the operating procedures of SOD typing colorimetry kit, GSH-PX colorimetry kit, and CAT colorimetry kit instruction.
The data obtained in this study were expressed by the mean ± standard deviation. Each experiment was repeated at least three times. The experimental data were processed by GraphPad Prism 8 software. Two-tailed student t-test was used to analyze the differences between the two groups. Multiple groups were compared using one-way ANOVA. P < 0.05 (*) was judged to be statistically significant.
The effect of trolox on Prostaglandin E2 secretion of UC-MSCs
A compound library was screened for enhancing the PGE-2 secretion by UC-MSCs. The screening data of the compound library showed that trolox exhibited the most apparent promoting effect on PGE-2 secretion (Fig. 1a). The UC-MSCs were treated with different concentrations of trolox for 24 h and exhibited enhancing effect on PGE-2 secretion exceeding 0.25 μM (38.13 to 53.58 ng/L). When UC-MSCs were treated for 48 h, high concentrations of trolox (exceeding 2 μM) showed a persistent promoting effect on PGE-2 section (41.50 to 89.68 ng/L) (Figs. 1b and 1c).
Figure 1: Trolox promotes the Prostaglandin E2 (PGE-2) secretion of umbilical cord mesenchymal stem cells (UC-MSCs). (a) Trolox was screened from the compound library to promote the PGE-2 secretion. (b, c) The UC-MSCs were cultured with trolox at concentrations as indicated for 24 h (b) or 48 h (c), respectively, and the PGE-2 concentrations were determined. ****P < 0.0001.
Effects of trolox on proliferation and surface marker expression of umbilical cord mesenchymal stem cells
The effect of trolox on the surface marker expression of MSCs was detected. Fig. 2a shows that trolox exhibited a significantly stimulating effect on MSCs proliferation when the concentration exceeded 5 μM. In addition, the results in Figs. 2b and 2c demonstrated that the expression of surface markers such as CD34, CD45, CD73, CD90, and CD105 was not affected after continuous treatment with trolox.
Figure 2: Trolox promotes the proliferative capacity of mesenchymal stem cells (MSCs) without altering their surface markers. (a) The fold proliferation of MSCs treated with different concentrations of trolox. (b) The positive rate of the surface markers of MSCs in the experimental group and the control group. (c) Flow cytometry for the Detection of the surface markers of MSCs in the experimental and control groups, Flow Jo processing the results. **P < 0.01.
The anti-aging effect of trolox on mesenchymal stem cells
The morphology of aging MSCs treated with trolox was further observed. Fig. 3a shows that the aging MSCs became larger, tabular, had multi-tentacles, and were β- galactosidase-staining positive. Fig. 3b shows the average positive rate of six random visual fields under the microscope. The results confirmed that the MSCs treated with trolox for a single time did not change the positive rate, but after several passage numbers with trolox, the positive rate decreased (55.5% to 43.75%). Fig. 3c confirms that as passage numbers increased, PGE-2 secretion of MSCs did not decrease after treatment with trolox, while PGE-2 secretion of the control group without trolox decreased after P10. Fig. 3d shows that the proliferation ability of MSCs decreased from P12 without trolox treatment, while in the trolox treatment group, the proliferation ability remained the same as before P16.
Figure 3: The anti-aging effect of trolox on mesenchymal stem cells (MSCs). (a) The morphology of β-galactosidase staining positive MSCs. The red lines represent the scale bar of 200 μm. (b) The rate of β-galactosidase staining positive MSCs after trolox treatment after a short time (left) and long time (right). (c, d) The Prostaglandin E2 (PGE-2) levels (c) and cell numbers (d) of MSCs cultured in the presence or absence of trolox for passages. *P < 0.05, **P < 0.01, ****P < 0.0001.
Trolox up-regulates the expression of miR-17-92 clusters and anti-oxidative enzymes in umbilical cord mesenchymal stem cells
The members of miR-17-92 clusters are considered the pro-proliferation miRNAs in several types of cells. In Fig. 4a, the expression of miR-17-92, including miR-17-3p, miR-17-5p, miR-19b-3p, miR-20a-5p, and miR-92a-3p in trolox treated MSCS are at least 3 folds higher than that of control group. The results in Fig. 4b show up-regulated activities of antioxidant enzymes, including CAT (2.51 U/ML to 4.41 U/ML), T-SOD (17.21 U/ML to 26.09 U/ML), and GSH-PX (651.70 U to 919.30 U) in the culture supernatant of MSCs after the continuous treatment with trolox. The expression of prdx5, prdx6, and gpx4 genes is up-regulated as well, as shown in Fig. 4c.
Figure 4: Mechanisms of proliferation and anti-aging of trolox treating on mesenchymal stem cells (MSCs). (a) Expression of miR-17-92 cluster genes in MSCs after once (1) and multiple (2) Trolox treatments. (b) After trolox treatment on MSCs, the activities of antioxidant enzymes catalase (CAT), superoxide dismutase (SOD), an glutathione peroxidase (GSH-Px) in the supernatant. (c) Expression of peroxiredoxins (prdx) families genes and gpx4 gene after trolox treatment on MSCs. *P < 0.05, **P < 0.01, ****P < 0.0001.
MSCs are currently utilized in regenerative medicine and clinical therapies, but the susceptibility to aging during in vitro culture largely limits their application. Thus, the strategies to suppress the aging of MSCs could facilitate their application. The regenerative ability of MSCs is mainly associated with the secretion of growth factors and immunomodulatory regulators, including (Shi et al., 2012) PGE-2, TGFβ, and IL-10 (Németh et al., 2009). Among MSC-derived immunomodulatory regulators, PGE-2 plays an important role in immune activity (DelaRosa et al., 2009) by regulating the production of various interleukins and tumor necrosis factor-α (TNF-α) production and promotes tissue regeneration as well as endocytosis restoration (Nakanishi and Rosenberg, 2013). Thus, PGE-2 secretion represents the regenerative ability of MSCs. Compound library screening revealed that trolox could significantly promote MSCs to secrete PGE-2. Trolox is a water-soluble vitamin E and could promote proliferation and reduce intracellular ROS level and p53 expression in cell lines (Diao et al., 2016). This study further explored its effects on aging and proliferation of MSCs. The in vitro data demonstrated that trolox treatment promotes MSC proliferation and does not change the surface antigens of MSCs. It might be beneficial for their expansion in culture.
The common opinion is that MSC aging during in vitro culture is caused by oxidative stress, and high levels of reactive oxygen species (ROS) production will cause aging, as well as even apoptosis of MSCs (Wen et al., 2020). Previous studies demonstrated that trolox can scavenge excess ROS and act as an antioxidant therapy in myocardial injury and diabetic retinopathy (Diaz et al., 2005). Trolox also exhibits a preventive effect on oxidative stress-induced cell death (Sarveazad et al., 2016) and apoptosis in thymocytes, cardiomyocytes, and anterior pituitary cells (Poliandri et al., 2003). This study focused on the anti-aging effect of trolox in MSCs culture. Long-term culture of MSCs in the presence of trolox could significantly reduce the number of aging MSCs, and the regenerative ability of MSCs was well retained. The result implies that trolox treatment was able to retard the aging of MSCs.
The regenerative medicine and clinical application of MSCs are attributed to their regenerative capacity (Odabas et al., 2014), which are closely related to secretome and anti-oxidative ability (González-González et al., 2020). The regenerative capacity of MSCs gradually decreases or even stagnates as passage numbers increase and are associated with changes in the secretome. The MSC secretome consists of growth factors, cytokines, and regenerative miRNAs. It was also found that multiple treatments of MSCs with trolox increased the expression of the miR-17-92 clusters. These RNA clusters are located on human chromosome 13 and encode six mature miRs, including miR-17, miR-18a, miR-19a, miR-20a, miR-19b-1, and miR-92a-1 (Németh et al., 2009). Over-expression of miR-17-92 clusters compensates for c-MYC inactivation (Sylvestre et al., 2007), allowing cellular proliferation to be promoted both in vivo and in vitro (Li et al., 2014). Therefore, we speculate that trolox promotes MSCs growth by probably up-regulating the expression of miR-17-92 clusters. Excessive production of ROS in MSCs during in vitro culture might be harmful to cells. MSCs generate antioxidant systems which could avoid damage by resisting excessive ROS. The antioxidant system consists of multiple enzymes, including CAT, SOD, and GSH-Px, which are involved in the direct scavenging of ROS (Gönenç et al., 2006). CAT, SOD, and GSH-PX have been shown to play an important role in cell growth, proliferation, differentiation, and apoptosis through their anti-ROS effects (Maiorino et al., 1990). The activity of these antioxidant enzymes increased in the culture supernatant of MSCs treated with trolox. In addition, the long-term culture of MSCs in the presence of trolox leads to the up-regulation of the antioxidant-related genes prdx families and gpx4, including prdx5, prdx6, and gpx4. Therefore, we hypothesize that trolox resists the aging of MSCs by up-regulating antioxidant-related genes and enzymes. Trolox treatment could maintain the proliferative capacity and the immunomodulatory ability of MSCs might be associated with the enhanced expression of antioxidant enzymes.
In conclusion, this study revealed that the antioxidant compound trolox could enhance the expression of miR-17-92 clusters and antioxidant enzymes in MSCs. Trolox inhibits aging, promotes proliferation, and enhances the secretion of PGE-2 of MSCs when cultured in vitro. Thus, the addition of trolox to in vitro expansion of MSCs will be beneficial.
Availability of Data and Material: All data generated or analyzed during this study are included in this article.
Author Contributions: The authors confirm their contribution to the paper as follows: study conception and design: Wei Wang, Li-Sheng Wang; experimentation: Xiao-Xu Zhang, Lin Zhang, Lin Du, Hui-Yan Sun; analysis and interpretation of results: Xia Zhao, Yang Sun; draft manuscript preparation: Xiao-Xu Zhang. All authors have reviewed the results and approved the final version of the manuscript.
Ethics Approval: This study was approved by the Medical Ethics Committee of the Affiliated Hospital of Qingdao University (Approval No. QYFYWZLL27240).
Funding Statement: This work was supported by the Natural Science Foundation of Shandong Province (ZR2020MH327), Hebei Key Research and Development Program (19272405D), and Jilin Scientific and Technological Development Program (Grant No. 20190304041YY).
Conflicts of Interest: The authors declare that they have no conflicts of interest to report regarding the present study.
References
Aggarwal S, Pittenger MF (2005). Human mesenchymal stem cells modulate allogeneic immune cell responses. Blood 105: 1815–1822. DOI 10.1182/blood-2004-04-1559. [Google Scholar] [CrossRef]
An JH, Song WJ, Li Q, Kim SM, Yang JI, Ryu MO, Nam AR, Bhang DH, Jung YC, Youn HY (2018). Prostaglandin E2 secreted from feline adipose tissue-derived mesenchymal stem cells alleviate DSS-induced colitis by increasing regulatory T cells in mice. BMC Veterinary Research 14: 354. DOI 10.1186/s12917-018-1684-9. [Google Scholar] [CrossRef]
DelaRosa O, Lombardo E, Beraza A, Mancheño-Corvo P, Ramirez C et al. (2009). Requirement of IFN-gamma-mediated indoleamine 2,3-dioxygenase expression in the modulation of lymphocyte proliferation by human adipose-derived stem cells. Tissue Engineering Part A 15: 2795–2806. DOI 10.1089/ten.tea.2008.0630. [Google Scholar] [CrossRef]
Diao QX, Zhang JZ, Zhao T, Xue F, Gao F, Ma SM, Wang Y (2016). Vitamin E promotes breast cancer cell proliferation by reducing ROS production and p53 expression. European Review for Medical and Pharmacological Sciences 20: 2710–2717. [Google Scholar]
Diaz Z, Colombo M, Mann KK, Su H, Smith KN, Bohle DS, Schipper HM, Miller Jr WH (2005). Trolox selectively enhances arsenic-mediated oxidative stress and apoptosis in APL and other malignant cell lines. Blood 105: 1237–1245. DOI 10.1182/blood-2004-05-1772. [Google Scholar] [CrossRef]
Gönenç A, Erten D, Aslan S, Akinci M, Simşek B, Torun M (2006). Lipid peroxidation and antioxidant status in blood and tissue of malignant breast tumor and benign breast disease. Cell Biology International 30: 376–380. DOI 10.1016/j.cellbi.2006.02.005. [Google Scholar] [CrossRef]
González-González A, García-Sánchez D, Dotta M, Rodríguez-Rey JC, Pérez-Campo FM (2020). Mesenchymal stem cells secretome: The cornerstone of cell-free regenerative medicine. World Journal of Stem Cells 12: 1529–1552. DOI 10.4252/wjsc.v12.i12.1529. [Google Scholar] [CrossRef]
Krull AA, Setter DO, Gendron TF, Hrstka SCL, Polzin MJ et al. (2021). Alterations of mesenchymal stromal cells in cerebrospinal fluid: Insights from transcriptomics and an ALS clinical trial. Stem Cell Research & Therapy 12: 187. DOI 10.1186/s13287-021-02241-9. [Google Scholar] [CrossRef]
Li Y, Choi PS, Casey SC, Dill DL, Felsher DW (2014). MYC through miR-17-92 suppresses specific target genes to maintain survival, autonomous proliferation, and a neoplastic state. Cancer Cell 26: 262–272. DOI 10.1016/j.ccr.2014.06.014. [Google Scholar] [CrossRef]
Maiorino M, Gregolin C, Ursini F (1990). Phospholipid hydroperoxide glutathione peroxidase. Methods in Enzymology 186: 448–457. DOI 10.1016/0076-6879(90)86139-M. [Google Scholar] [CrossRef]
Nakanishi M, Rosenberg DW (2013). Multifaceted roles of PGE2 in inflammation and cancer. Seminars in Immunopathology 35: 123–137. DOI 10.1007/s00281-012-0342-8. [Google Scholar] [CrossRef]
Nekanti U, Dastidar S, Venugopal P, Totey S, Ta M (2010). Increased proliferation and analysis of differential gene expression in human wharton’s jelly-derived mesenchymal stromal cells under hypoxia. International Journal of Biological Sciences 6: 499–512. DOI 10.7150/ijbs.6.499. [Google Scholar] [CrossRef]
Németh K, Leelahavanichkul A, Yuen PS, Mayer B, Parmelee A et al. (2009). Bone marrow stromal cells attenuate sepsis via prostaglandin E2-dependent reprogramming of host macrophages to increase their interleukin-10 production. Nature Medicine 15: 42–49 DOI 10.1038/nm.1905. [Google Scholar] [CrossRef]
Odabas S, Elcin AE, Elcin YM (2014). Isolation and characterization of mesenchymal stem cells. Methods in Molecular Biology 1109: 47–63. DOI 10.1007/978-1-4614-9437-9. [Google Scholar] [CrossRef]
Poliandri AH, Cabilla JP, Velardez MO, Bodo CC, Duvilanski BH (2003). Cadmium induces apoptosis in anterior pituitary cells that can be reversed by treatment with antioxidants. Toxicology and Applied Pharmacology 190: 17–24. DOI 10.1016/S0041-008X(03)00191-1. [Google Scholar] [CrossRef]
Prockop DJ, Oh JY (2012). Mesenchymal stem/stromal cells (MSCsRole as guardians of inflammation. Molecular Therapy 20: 14–20. DOI 10.1038/mt.2011.211. [Google Scholar] [CrossRef]
Rezk BM, Haenen GR, van der Vijgh WJ, Bast A (2004). The extraordinary antioxidant activity of vitamin E phosphate. Biochimica et Biophysica Acta 1683: 16–21. DOI 10.1016/j.bbalip.2004.03.005. [Google Scholar] [CrossRef]
Sarveazad A, Babahajian A, Yari A, Goudarzi F, Soleimani M, Nourani M (2016). Neuroprotective role of trolox in hippocampus after ischemia reperfusion injury in mouse. International Journal for Vitamin and Nutrition Research 86: 228–234. DOI 10.1024/0300-9831/a000293. [Google Scholar] [CrossRef]
Shi Y, Su J, Roberts AI, Shou P, Rabson AB, Ren G (2012). How mesenchymal stem cells interact with tissue immune responses. Trends in Immunology 33: 136–143. DOI 10.1016/j.it.2011.11.004. [Google Scholar] [CrossRef]
Sylvestre Y, de Guire V, Querido E, Mukhopadhyay UK, Bourdeau V, Major F, Ferbeyre G, Chartrand P (2007). An E2F/miR-20a autoregulatory feedback loop. The Journal of Biological Chemistry 282: 2135–2143. DOI 10.1074/jbc.M608939200. [Google Scholar] [CrossRef]
Verma AK, Pandey AK, Singh A, Kant S, Mahdi AA, Prakash V, Ansari KM, Dixit RK, Chaudhary SC (2022). Increased serum levels of matrix-metalloproteinase-9, cyclo-oxygenase-2 and prostaglandin E-2 in patients with chronic obstructive pulmonary disease (COPD). Indian Journal of Clinical Biochemistry 37: 169–177. DOI 10.1007/s12291-021-00973-2. [Google Scholar] [CrossRef]
Wen C, Huang C, Yang M, Fan C, Li Q, Zhao J, Gan D, Li A, Zhu L, Lu D (2020). The secretion from bone marrow mesenchymal stem cells pretreated with berberine rescues neurons with oxidative damage through activation of the Keap1-Nrf2-HO-1 signaling pathway. Neurotoxicity Research 38: 59–73. DOI 10.1007/s12640-020-00178-0. [Google Scholar] [CrossRef]
Yang Z, Concannon J, Ng KS, Seyb K, Mortensen LJ et al. (2016). Tetrandrine identified in a small molecule screen to activate mesenchymal stem cells for enhanced immunomodulation. Scientific Reports 6: 30263. DOI 10.1038/srep30263. [Google Scholar] [CrossRef]
Yueh-Hsun Y, Ogando CR, See CW, Chang TY, Barabino GA (2018). Changes in phenotype and differentiation potential of human mesenchymal stem cells aging in vitro. Stem Cell Research & Therapy 9: 131. DOI 10.1186/s13287-018-0876-3. [Google Scholar] [CrossRef]
Zhang Z, Huang S, Wu S, Qi J, Li W et al. (2019). Clearance of apoptotic cells by mesenchymal stem cells contributes to immunosuppression via PGE2. eBioMedicine 45: 341–350. DOI 10.1016/j.ebiom.2019.06.016. [Google Scholar] [CrossRef]
Cite This Article
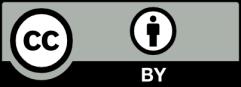
This work is licensed under a Creative Commons Attribution 4.0 International License , which permits unrestricted use, distribution, and reproduction in any medium, provided the original work is properly cited.