Open Access
ARTICLE
Inhibition of H2O2-induced apoptosis of GC2-spg cells by functionalized selenium nanoparticles with lentinan through ROS-mediated ERK/p53 signaling pathways
1 Department of Andrology, Guangzhou Women and Children’s Medical Center, Guangzhou Medical University, Guangzhou, 510120, China
2 Center Laboratory, Guangzhou Women and Children’s Medical Center, Guangzhou Medical University, Guangzhou, 510120, China
* Corresponding Authors: YINGHUA LI. Email: ; LIANDONG ZUO. Email:
# These authors contributed equally to this work
BIOCELL 2023, 47(2), 401-408. https://doi.org/10.32604/biocell.2023.025154
Received 24 June 2022; Accepted 15 August 2022; Issue published 18 November 2022
Abstract
A H2O2-induced oxidative stress injury cell model was established to investigate the antioxidant effect of nano-selenium on mouse spermatocyte lines and the regulation mechanism of the expression level and activity of selenium-containing antioxidant enzymes induced by oxidative stress. A safe and effective nano-drug system of functionalized selenium-containing nanoparticles (SeNPs) was developed with lentinan (LNT) (SeNPs@LNT). Mice spermatocyte line GC2-spg cells were treated with SeNPs@LNT (1, 2, 4, 8, 16, 32 μM) for 24–72 h to evaluate the cytotoxicity of selenium. GC2-spg cells were randomly divided into the following groups: control, hydrogen peroxide (H2O2), SeNPs@LNT, and H2O2+SeNPs@LNT groups. H2O2+SeNPs@LNT group was pretreated with SeNPs@LNT 4 μM for 12 h, followed by H2O2 600 μM for 8 h. The cell viability decreased in the H2O2 group and increased significantly in the SeNPs@LNT group. Compared with the H2O2 group, the SeNPs@LNT+H2O2 group exhibited obvious red fluorescence, indicating a higher level of mitochondrial membrane potential. The content of intracellular reactive oxygen species (ROS) in the SeNPs@LNT group reduced significantly, and the intensity of green fluorescence in the SeNPs@LNT+H2O2 group decreased significantly compared with the H2O2 group, indicating the inhibitory effect of SeNPs@LNT on the generation of ROS-induced oxidative stress. The activity of GPx and SOD increased significantly in the SeNPs@LNT group. The expression of p53 decreased significantly under the intervention of nano-selenium, and GPx1 expression increased. In the oxidative stress group, the expressions of DNA damage-related proteins and apoptosis-related proteins were higher than those in other groups. Thus, SeNPs@LNT can promote GC2-spg cell proliferation, improve GPx and SOD activities, remove intracellular ROS, and reduce mitochondrial damage and functional abnormalities caused by oxidative stress by regulating the ERK and p53 protein levels. SeNPs@LNT has good biological activity and antioxidant effect, which can be used to protect the male reproductive system from oxidative stress.Keywords
Selenium (Se) is an important trace mineral having several essential roles at the cellular and organismal levels and affecting animal and human health (Qazi et al., 2019). In nature, selenium exists in three forms, organic selenium, inorganic selenium, and nano-selenium; among these, nano-selenium has the least physiological toxicity, and its application has been widely investigated (Falchi et al., 2018; Hosnedlova et al., 2018; Khurana et al., 2019). Selenium is added as selenocysteine (SEC) to various antioxidant enzymes such as glutathione peroxidase (GPx), thioredoxin reductase, and selenophosphorin P (SeP) (Labunskyy et al., 2014; Rayman, 2020). Selenium is the redox center for all these enzymes and is essential for their biochemical activity (Avery and Hoffmann, 2018; Burk and Hill, 2015). The key role of selenium in male reproduction is mediated by two selenoproteins, phospholipid hydrogen peroxide (H2O2) glutathione peroxidase (PHGPx/GPx4) and selenoprotein P (Boitani and Puglisi, 2008; Hariharan and Dharmaraj, 2020). GPx4 is an oxidative inactivation protein that constitutes more than 50% of the mitochondrial envelope in the midsection of mature sperm (Foresta et al., 2002). GPx4 is thought to protect developing sperm from oxidative stress-induced DNA damage early in the sperm formation process (Ingold et al., 2015). GPx1 mainly acts as an antioxidant enzyme, scavenging free radicals in cells and protecting sperm from oxidative stress. SeP, as a selenium transporter, is expressed in the vesicle-like structure of the basal region of Sertoli cells for selenium uptake (Maiorino et al., 2018).
Lentinan is a β-glucan extracted from Lentinus edodes, which has been found to be safe but with low bioefficiency in the treatment of malignant ascites in studies conducted in China (Liu et al., 2019). Nano-selenium has better biocompatibility, and the functional nano-selenium is more stable than pure nano-selenium dispersed in water (Chen et al., 2019). Previous studies have found that L. edodes polysaccharide can enhance the targeting of SeNPs to tumor cells, and its -OH group interacts with nano-selenium to obtain surface-modified nano-selenium, which has stronger anti-inflammatory and mitochondrial effects (Liu et al., 2020).
Oxidative stress injury is one of the main factors leading to the decline of male fertility (Chen et al., 2012; Gong et al., 2012). Under normal circumstances, reactive oxygen species (ROS) production and clearance in testicular tissue are in dynamic balance and thus maintain the integrity and normal function of testicular tissue (Aitken et al., 2010; Zhang et al., 2016; Zorov et al., 2014). The reproductive system produces a small amount of ROS, which is important for physiological functions, such as sperm tolerance, hyperactive motility, energy acquisition, acrosome reaction, and sperm-egg fusion (Aitken, 2017; Dutta et al., 2019; Zini et al., 2000). However, too much ROS can cause toxic effects, namely peroxidation, leading to cell membrane damage. Its peroxidation products and the interaction of proteins and nucleic acids in cells cause chromosome structure damage and enzyme function damage, resulting in testicular tissue damage (Gong et al., 2012; Takeshima et al., 2021). Selenoproteins, as described above, act as antioxidant enzymes that protect cells against oxidative damage. This study aimed to explore the protective mechanism of nano-selenium on oxidative stress injury of hydrogen peroxide-induced mice spermatocyte GC2-spg cells. The oxidative damage model was established by inducing using H2O2. The oxidative stress model was then treated with different concentrations of nano-selenium. The cell viability was determined using the Cell Counting Kit-8 (CCK-8). Mitochondrial function and ROS were also measured. The levels of superoxide dismutase (SOD) and total glutathione peroxidase were measured by spectrophotometer, and the changes in apoptosis and DNA damage-related proteins were studied. We found that nano-selenium plays an antioxidant role through the p53 signaling pathway during hydrogen peroxide-induced spermatogastoma injury, which improves the intracellular antioxidant level and reduces mitochondrial damage and DNA damage.
CCK8 kit (C0038) and BCA protein assay kit (P0009) were purchased from Beyotime (China). Anti-caspase 8 antibodies, extracellular-signal-regulated kinase (ERK) antibodies, anti-signal transducer and activator of transcription 3 (STAT3) antibodies, anti-ß-actin, anti-caspase 8 antibodies, and anti-mouse IgG secondary antibodies for western blotting were obtained from Cell Signaling Technology (USA). Fetal bovine serum (FBS) (10099) and Dulbecco’s modified Eagle’s medium (DMEM) (11965092) were procured from Gibco (USA). Mitochondrial membrane potential detection kit (JC-1) (MAK160) were purchased from Sigma (USA). ROS detection kit (S0033), glutathione peroxidase detection kit (S0059S), and total SOD activity detection kit (S0109) were purchased from Beyotime (China).
Preparation and characterization of selenium-containing nanoparticles
SeNPs@LNT was prepared by the team of Professor Chen Tianfeng, Jinan University, Jinan, China. The synthesis and characterization data of nano-selenium are described earlier (Liu et al., 2020).
GC2-spg cells were obtained from the American Type Culture Collection (ATCC, USA). Cells were maintained in DMEM supplemented with 10% FBS, streptomycin (100 μg/mL), and penicillin (100 U/mL). When the cell growth was confluent to the density of 70%–80%, FBS concentration was changed. Cells were maintained in DMEM supplemented with 5% FBS, streptomycin (100 μg/mL), and penicillin (100 U/mL) at 37°C in an incubator with a humidified atmosphere of 5% CO2. GC2-spg cells were treated with SeNPs@LNT at 1, 2, 4, 8, 16, and 32 μM concentrations for 24 h to evaluate the cytotoxicity of selenium. GC2-spg cells at the logarithmic growth stage were cultured for 12 h, and after adherence, the solution was changed. GC2-spg cells were randomly divided into the control group, H2O2 group, SeNPs group, and H2O2+SeNPs group (SeNPs@LNT 4 μM pretreatment for 12 h, then H2O2 600 μM treatment for 8 h).
As mentioned above, cell viability was determined by the CCK-8 method. Untreated GC2-spg cells were seeded in a 96-well plate at a density of 5 × 104/mL and incubated overnight at 37°C. GC2-spg cells were then divided into four groups, as mentioned above. Cells in each group were treated accordingly, then 100 μL CCK-8 solution was added to the 96-well plate and incubated for 1 h. The absorbance at 570 nm was read by a microplate reader (Varioskan Flash, Thermo, USA).
Detection of mitochondrial membrane potential (∆Ψm) and intracellular reactive oxygen species levels
After the above treatment, the transformation of mitochondrial membrane potential, which is closely relative to the cell apoptosis, was assessed by JC-1, described as follows: GC2-spg cells were cultured in a 6-well microtiter plate at a density of 5 × 104/mL for 24 h. Then, after digestion and resuspension in PBS with 10 μg/mL of JC-1, the GC2-spg cells were incubated at 37°C for 30 min. Finally, mitochondrial membrane potential was observed under a fluorescence microscope (Leica, Germany). ROS detection method was as follows: GC2-spg cells were cultured on a 96-well droplet plate at a density of 5 × 104/mL for 24 h. In the nano-selenium and H2O2 treatment group, GC2-spg cells were pretreated with 4 μM nano-selenium for 12 h and 600 μM H2O2 for 8 h. The cells were stained with 10 μM DCFH-DA for 30 min. Two techniques, microscopy and fluorometry, were used to measure intracellular ROS levels. Images of each group were acquired with use of a fluorescence microscope (Leica) at the same exposure time. Fluorescence intensity of 488 nm excitation and 525 nm emission was measured with a fluorescence plate reader (Thermo Fisher Scientific, USA).
Glutathione peroxidase activity
For the total glutathione peroxidase assay, cells were treated above according to different groups. Cell lysates were prepared as per the manufacturer’s instructions. Lysis was performed at a rate of 100–200 µL of lysate per million cells. The lysate was homogenized in a glass homogenizer at 4°C or ice bath and centrifuged at 12000×g at 4°C for 10 min. The supernatant was used to determine the enzyme activity. NADPH and GSH solutions were prepared, and except for the part to be used immediately, the remaining GSH solution was properly aliquoted and stored at −20°C. The GPx detection working fluid was prepared. The 96-well plate was taken, and the detection buffer, the sample to be tested, and the GPx detection working solution were successively added and then mixed. Then 40 µL GPx detection working solution was added and incubated at room temperature for 15 min, followed by the addition of 10 µL of 30 mM peroxide solution to each well and mixed. A microplate reader (Thermo Fisher Scientific, USA) was immediately used to determine A340, with a continuous determination of A340 once for 5 min. The glutathione peroxidase activity in the samples was calculated by recording the data of six points for at least 5 min.
Cell lysates were prepared in the same way. The cell culture medium was aspirated, and the cells were washed with PBS or normal saline pre-cooled at 4°C or ice bath. The SOD sample preparation solution provided with this kit was added at a ratio of 100–200 µL/1 × 106 cells. Wst-8/enzyme working solution and reaction starter was prepared, and samples to be tested and other solutions were successively added. The reaction starting working solution was added and mixed thoroughly. The absorbance was measured at 450 nm, and the SOD activity was calculated.
GC2-spg cells were cultured in a 12 cm cell culture dish at a density of 8 × 104/mL for 24 h and treated as specified. The cells were placed in the lysis buffer to obtain cellular proteins. Protein concentration was determined by the bicinchoninic acid method. Sample loading was 20 μg and transferred to nitrocellulose membrane at the appropriate voltage. After that, TBSE buffer (Tween-20, Tris-Base, and 10 × TBS, pH 7.4) was added with skim milk for 1 h for blocking. The primary antibody was diluted at 1:1000, and an appropriate amount of primary antibody was added to submerge the PVDF membrane, and the reaction was conducted overnight at 4°C. After that, it was incubated with a secondary antibody for 1–2 h. Enhanced chemiluminescence reagents (Clinx, Shanghai, China) were used for X-ray film development.
All data were obtained from three repeated independent tests. SPSS software was used for statistical analysis. Continuous variables were expressed as mean ± SD. Data were tested for normality by performing one-way ANOVA; if data were not distributed normally, Kruskal-Wallis analysis method was used. The differences between groups were analyzed, followed by the Bonferroni post hoc test on the SPSS software package (version 19.0, SPSS Inc., Chicago, IL, USA) and GraphPad software (version 7, GraphPad Software, Inc., La Jolla, CA, USA). P-value less than 0.05 (*) indicated statistical significance.
Establishment of oxidative stress model
The results of treatment of GC2-spg cells were treated with different concentrations of H2O2 (0, 125, 250, 500, 600, 700, 800, and 1000 μM) for 8 h are shown in Fig. 1A. With the increase in H2O2 concentration, cell viability decreased gradually, and when the concentration was 600 μM, cell viability decreased to 76.07% (p < 0.001), which was statistically significant. To ensure oxidative damage and avoid the decline in cell viability to irreversible damage, a suitable H2O2 concentration of 600 μM and treatment time of 8 h were selected as the oxidative stress model for subsequent experiments.
Figure 1: Effect of SeNPs@LNT on hydrogen peroxide (H2O2)-induced Mice spermatocyte line GC2-spg cells. (A) GC2-spg cells were exposed to increased concentrations of H2O2 (from 0 to 1000 μM) for 8 h; (B) GC2-spg cells were treated with SeNPs@LNT 1, 2, 4, 8, 16, and 32 μM for 24 h to evaluate the cytotoxicity of selenium. (C) GC2-spg cells were treated with 4 μM SeNPs@LNT for 12 h before being challenged by 600 μM H2O2 for 8 h. Cell viability was determined by CCK-8 assay. (D) Microscopic observation results of each treatment group. All data were presented as mean ± SD of three separate experiments. Compared with the control: *p < 0.05, ***p < 0.001, ****p < 0.0001.
Antioxidant and cell proliferation effects of nano-selenium in vitro
We used 4 µM nano-selenium to pretreat the normal control group and the oxidative stress model group for 12 h. The results after treatment are shown in Figs. 1B and 1C. The cell viability of the H2O2 group decreased to 72.09%, and the difference was statistically significant (p < 0.001), the cell viability of the SeNPs@LNT+H2O2 group was 88.02% and compared with that of H2O2 group, the difference was statistically significant (p < 0.05). The cell viability of the SeNPs@LNT treatment group was 40%, and the difference was statistically significant compared to those of the H2O2 and SeNPs@LNT+H2O2 groups. Fig. 1D shows that the cell density of the group pretreated with nano-selenium increased, and the number of apoptotic cells decreased. Thus, nano-selenium promoted cell proliferation and protected cells in the oxidative stress environment.
Nano-selenium has been widely used in the development of anti-tumor therapy and targeted transport carrier due to its low biotoxicity and low molecular weight (Ferro et al., 2021). It is also involved in the maintenance of intracellular oxidative stress levels and is also applied in the field of oxidative stress. Ibrahim et al. (2012) found significant differences in sperm index, penis length, testicular weight, and histopathological features in mice on a high-fat diet with probiotics, inorganic selenium, selenium-enriched probiotics (organic selenium form), and lower total cholesterol. Liu et al. (2017a) found that nano-selenium supertrophic dose promoted sperm motility and GPx1 and GPx4 gene expressions in male SD rats exposed to the nano-selenium suspension. They reported that the intake of nano-selenium, compared to organic and inorganic selenium, could improve the male reproductive system and fertility of healthy animals or even make it better than the normal level. This is consistent with the results obtained in this study and reflects the characteristics of low toxicity and high activity of nano-selenium.
SeNPs@LNT could prevent the reduction of mitochondrial membrane potential induced by oxidative stress
In high mitochondrial membrane potential, JC-1 aggregates in the mitochondrial matrix and forms polymer (J-aggregates), which produces red fluorescence; under low mitochondrial membrane potential, JC-1 cannot aggregate in the matrix. At this time, JC-1 is a monomer and can produce green fluorescence. The decrease in mitochondrial membrane potential is a landmark event in the early stage of apoptosis. The decrease in cell membrane potential can be easily detected by the transformation of JC-1 from red fluorescence to green fluorescence, and this transformation can also be used as an indicator of early apoptosis. The ratio of mitochondrial depolarization was measured by observing the relative ratio of red and green fluorescence of the mitochondria in the JC-1 staining solution. The decrease in mitochondrial membrane potential can be regarded as a landmark event in the early stage of apoptosis. As shown in Fig. 2, compared with the H2O2 group, the SeNPs@LNT+H2O2 group had obvious red fluorescence, that is, a higher level of mitochondrial membrane potential. The fusion fluorescence revealed the red-green fluorescence ratio was higher in the SeNPs@LNT treatment group than that in the oxidative stress group.
Figure 2: The mitochondrial membrane potential of GC2-spg cells was detected with JC-1 fluorescent probe under a fluorescent microscope. JC-1 aggregates emit red fluorescence, and JC-1 monomers emit green fluorescence. Changes in mitochondrial membrane potential were observed by the change from red fluorescence to green fluorescence.
The roxburgh rose polysaccharide functionalized nano-selenium RP3-SenPs has an anti-apoptotic effect. Wang et al. (2019) found that the mitochondrial membrane potential of INS-1 cells treated with H2O2 decreased, and selenium pretreatment significantly changed this situation. Liu et al. (2017b) found that pretreatment with functionalized nano-selenium can prevent mitochondrial fragmentation caused by oxidative stress and reduce the production of ROS and caspase 3. Cui et al. (2018) reported that biosynthesized functionalized nano-selenium He-SeNPs, and pretreatment can change the mitochondrial membrane potential of hepatocellular carcinoma cells and the cellular apoptotic status, thus reflecting the anticancer effect of nano-selenium.
Inhibition of reactive oxygen species generation by SeNPs@LNT
The level of intracellular ROS production is shown in Fig. 3. DCFH-DA can freely cross the cell membrane, and after entering the cell, it is hydrolyzed by intracellular esterase to generate DCFH. However, DCFH cannot penetrate the cell membrane, which makes it easy for the probe to be loaded into the cell. Reactive oxygen species in the cell can oxidize non-fluorescent DCFH to generate fluorescent DCF. The fluorescence intensity of DCF reflected the generation of ROS in cells, which was observed by a fluorescence microscope. The content of intracellular ROS in the SeNPs@LNT group was significantly reduced, and the intensity of green fluorescence in the SeNPs@LNT+H2O2 group was significantly decreased compared with that in the H2O2 group, indicating that SeNPs@LNT had an inhibitory effect on the generation of ROS caused by oxidative stress.
Figure 3: Effect of SeNPs@LNT on H2O2-induced GC2-spg cells on reactive oxygen species (ROS) production. (A) ROS production of GC2-spg cells was detected by 2′7′-dichlorofluorescin-diacetate (DCFA-DH). (B) GC2-spg cells were treated with 4 μM SeNPs@LNT for 12 h before being challenged by 600 μM H2O2 for 8 h.
Xu et al. (2019a) demonstrated that the synthesized SeNPs of Lactobacillus casei ATCC 393 can alleviate oxidative damage induced by H2O2 in human and pig intestinal epithelial cells; they also detected increased production of ROS in the oxidative stress group. This finding was consistent with the results of this study, suggesting that the production of intracellular ROS induced by H2O2 may be undifferentiated, while selenium can inhibit the occurrence of this phenomenon. Meanwhile, the preparation of new functionalized SeNPs using Lactobacillus NZ9000 can also reduce the increase of intracellular ROS induced by H2O2 (Xu et al., 2019b).
SeNPs@LNT could increase the activity of intracellular antioxidant enzymes
The results of detecting intracellular glutathione peroxidase activity and SOD activity are shown in Fig. 4A. The average GPx activity was 32.76 mU/mg protein in the control group. The average GPx activity was 12.70 mU/mg protein in the oxidative stress group and 247.80 mU/mg protein (p < 0.001) in the SeNPs@LNT+H2O2 group; SeNPs@LNT group had the highest activity (362.05 mU/mg protein) (p < 0.001). Compared with the H2O2 alone group, SeNPs@LNT+H2O2 and SeNPs@LNT groups significantly increased the activity of glutathione peroxidase, p < 0.001 in both groups. As shown in Fig. 4B, the mean SOD activity of the control group was 1.32 U/mg protein, and that of the H2O2 group was 0.60 (p < 0.01); the mean value of SeNPs@LNT+H2O2 group was 1.68, and the mean value of SeNPs@LNT group was 3.25 (p < 0.001). We observed an experimental phenomenon in the detection results of SOD level after different intracellular treatments similar to that observed with GPx. Nano-selenium intervention can be observed to increase the activity of antioxidant enzymes, indicating that nano-selenium treatment can inhibit H2O2-mediated oxidative stress damage, enhance the antioxidant capacity of cells, and increase the activity of antioxidant enzymes to promote apoptosis.
Figure 4: Glutathione peroxidase (GPx) activity (A) and T-SOD (B) on H2O2-induced GC2-spg cells. GC2-spg cells were treated with 4 μM SeNPs@LNT for 12 h before being challenged by 600 μM H2O2 for 8 h.
This study was the first to investigate the antioxidant activity of nano-selenium on male spermatogamous cells. The male reproductive system has a high proportion of polyunsaturated acids in testicles and sperm, and is thus vulnerable to oxidative stress. Selenium-containing antioxidant enzymes play a role in scavenging oxidative stress products, especially ROS (Wadhwani et al., 2016). Changes in the content and activity of these enzymes may lead to sensitivity of cellular defense function (Ingold et al., 2015). Studies on selenium-containing supplements as antioxidant supplements have been reported to reduce testicular damage from heavy metal exposure, increase antioxidant enzyme levels in high-fat diet animals, and combine with metformin to treat diabetes in mice (Ebokaiwe et al., 2020). In 64 infertility patients diagnosed with varicocele, Ardestani found significantly improved male fertility in those who received vitamin E-selenium-folic acid antioxidant treatment (Ardestani et al., 2019). Lombardo et al. (2012) also found statistically significant improvements in inflammatory indices and sperm motility, and sperm morphology with the new supplementation containing selenium and zin. The antioxidant capacity was reflected in the decrease of lipid oxidation (malondialdehyde) and the increase of antioxidant enzyme activity. Shalini and Bansal found that an increase in lipid peroxidation and expression was observed in both selenium deficient group and the selenium excess group (Shalini and Bansal, 2007).
SeNPs@LNT activated the expression of reactive oxygen species-related proteins
On observing the intracellular signaling pathway proteins, we found a decreased expression of p53 significantly under the intervention of nano-selenium, and the level of GPx1 increased. In the oxidative stress group, the expressions of DNA damage-related proteins and apoptosis-related proteins were higher than those of the other groups. P53 protein is mainly distributed in the cytoplasm and can specifically bind to DNA, and its activity is regulated by post-translational modifications such as phosphorylation, acetylation, methylation, and ubiquitination. Normal p53 functions as a “guardian of the genome,” checking for DNA damage at G1 and monitoring genome integrity. If there is damage, the p53 protein blocks DNA replication to provide enough time for the damaged DNA to repair. If the repair fails, the p53 protein triggers apoptosis. ERKs phosphorylate not only cytoplasmic proteins but also some nuclear transcription factors such as C-FOS, C-Jun, ETS transcription factor ELK-1, C-MyC, and ATF2, thus participating in the regulation of cell proliferation and differentiation. Caspase 3 expression decreases after nano-selenium treatment (Huang et al., 2018). The expression level was found to be correlated with cell death, suggesting that nano-selenium treatment improved cell viability and alleviated oxidative stress injury (Zhang et al., 2019).
The expression level of p53 was significantly increased after H2O2 treatment and significantly decreased after nano-selenium treatment, and its expression was related to DNA replication and damage repair. The change in the expression of p53 indicated that nano-selenium had a protective effect on DNA damage due to oxidative stress. In addition, the expression level of ERK was higher than that of the other groups. Changes in the expression of other proteins, such as Caspase 3, and STAT-3, are shown in Fig. 5. The signal pathways triggered by nano-selenium treatment are shown in Fig. 6. In this study, exposure to acute or chronic oxidative stress potentially led to cellular damage that impairing normal physiological functions of organs. Cells protect themselves from severe damage by activating scavenging free radicals, and increasing the expression of p53 protein which could reflect DNA damage and repair. At the same time, more experiments are needed to verify this. This study did not extend to animal experiments, and the pathological characteristics of male testis after oxidative stress exposure, as well as the effects of nano-selenium on male reproductive hormone levels, were not explored.
Figure 5: The signaling pathways potentially activated by SeNPs@LNT treatment. The GC2-spg cells exposed to different treatments expressed the pro-apoptotic and anti-apoptotic protein tracked by western blotting. β-actin was used as the loading control.
Figure 6: Proposed signaling pathway triggered by SeNPs@LNT and H2O2 in GC2-spg cells.
To conclude, SeNPs@LNT could alleviate oxidative stress damage caused by H2O2 on GC2-spg cells and exert antioxidant capacity by increasing the activities of GPx1 and SOD. The mechanism may be related to ROS activation of signaling pathways, increased expression of GPx1, decreased expression of ERK, caspase 3, and p53, and regulation of apoptosis, DNA replication, and other functions. The results showed that nano-selenium, as an antioxidant supplement, could play an antioxidant role in male reproduction, improve semen quality, and enhance male fertility.
Availability of Data and Material: Data sharing is not applicable to this article as no datasets were generated or analyzed during the current study.
Author Contribution: Study conception and design: Miaomiao Li; data collection: Miaomiao Li, Junyi Ke, Danyang Chen; analysis and interpretation of results: Miaomiao Li, Ruilin Zheng, Jingyao Su, Zilin Zheng, Jieyi Luo; draft manuscript preparation: Miaomiao Li, Danyang Chen, Hanran Mai, Fan Jiang, Yanxia Qu, Xiaoqiong Gu. Bing Zhu, Yinghua Li and Liandong Zuo reviewed the results and approved the final version of the manuscript.
Ethics Approval: Not applicable.
Funding Statement: This study was funded by the open Project of Guangdong Key Laboratory of Marine Materia (LMM2020-7), the science and technology projects in Guangzhou (202201020632, 202201020638, 202206010100, 202201020655 and 202102010202), the Guangdong Natural Science Foundation (2020A1515110648), the Open Fund of Guangdong Provincial Key Laboratory of Functional Supramolecular Coordination Materials and Applications (2020A03). The Guangzhou Medical University Students’ Science and Technology Innovation Project (02-408-2203-2079, 2021AEK119).
Conflicts of Interest: The authors declare that they have no conflicts of interest to report regarding the present study.
References
Aitken RJ (2017). Reactive oxygen species as mediators of sperm capacitation and pathological damage. Molecular Reproduction and Development 84: 1039–1052. DOI 10.1002/mrd.22871. [Google Scholar] [CrossRef]
Aitken RJ, De Iuliis GN, Finnie JM, Hedges A, Mclachlan RI (2010). Analysis of the relationships between oxidative stress, DNA damage and sperm vitality in a patient population: Development of diagnostic criteria. Human Reproduction 25: 2415–2426. DOI 10.1093/humrep/deq214. [Google Scholar] [CrossRef]
Ardestani ZA, Arab D, Kia NS, Heshmati S, Amirkhalili SN (2019). The role of Vitamin E-selenium-folic acid supplementation in improving sperm parameters after varicocelectomy: A randomized clinical trial. Urology Journal 16: 495–500. DOI 10.22037/uj.v0i0.4653. [Google Scholar] [CrossRef]
Avery JC, Hoffmann PR (2018). Selenium, selenoproteins, and immunity. Nutrients 10: 1–20. DOI 10.3390/nu10091203. [Google Scholar] [CrossRef]
Boitani C, Puglisi R (2008). Selenium, a key element in spermatogenesis and male fertility. Advances in Experimental Medicine and Biology 636: 65–73. DOI 10.1007/978-0-387-09597-4. [Google Scholar] [CrossRef]
Burk RF, Hill KE (2015). Regulation of selenium metabolism and transport. Annual Review of Nutrition 35: 109–134. DOI 10.1146/annurev-nutr-071714-034250. [Google Scholar] [CrossRef]
Chen H, Zhao HX, Huang XF, Chen GW, Yang ZX et al. (2012). Does high load of oxidants in human semen contribute to male factor infertility? Antioxid Redox Signal 16: 754–759. DOI 10.1089/ars.2011.4461. [Google Scholar] [CrossRef]
Chen Q, Liu T, Chen S, Luo Y, Ma M, Xue F, Zhang L, Bao W, Chen H (2019). Targeted therapeutic-immunomodulatory nanoplatform based on noncrystalline selenium. ACS Applied Materials & Interfaces 11: 45404–45415. DOI 10.1021/acsami.9b15774. [Google Scholar] [CrossRef]
Cui D, Liang T, Sun L, Meng L, Yang C, Wang L, Liang T, Li Q (2018). Green synthesis of selenium nanoparticles with extract of hawthorn fruit induced HepG2 cells apoptosis. Pharmaceutical Biology 56: 528–534. DOI 10.1080/13880209.2018.1510974. [Google Scholar] [CrossRef]
Dutta S, Majzoub A, Agarwal A (2019). Oxidative stress and sperm function: A systematic review on evaluation and management. Arab Journal of Urology 17: 87–97. DOI 10.1080/2090598X.2019.1599624. [Google Scholar] [CrossRef]
Ebokaiwe AP, Obeten KE, Okori SO, David EE, Olusanya O, Chukwu CJ, Okoro N, Ehiri RC (2020). Co-administration of selenium nanoparticles and metformin abrogate testicular oxidative injury by suppressing redox imbalance, augmenting sperm quality and nrf2 protein expression in Streptozotocin-Induced diabetic rats. Biological Trace Element Research 198: 544–556. DOI 10.1007/s12011-020-02082-2. [Google Scholar] [CrossRef]
Falchi L, Khalil WA, Hassan M, Marei W (2018). Perspectives of nanotechnology in male fertility and sperm function. International Journal of Veterinary Science and Medicine 6: 265–269. DOI 10.1016/j.ijvsm.2018.09.001. [Google Scholar] [CrossRef]
Ferro C, Florindo HF, Santos HA (2021). Selenium nanoparticles for biomedical applications: From development and characterization to therapeutics. Advanced Healthcare Materials 10: e2100598. DOI 10.1002/adhm.202100598. [Google Scholar] [CrossRef]
Foresta C, Flohe L, Garolla A, Roveri A, Ursini F, Maiorino M (2002). Male fertility is linked to the selenoprotein phospholipid hydroperoxide glutathione peroxidase. Biology of Reproduction 67: 967–971. DOI 10.1095/biolreprod.102.003822. [Google Scholar] [CrossRef]
Gong S, San GM, Zini A, Chan P, O’Flaherty C (2012). Low amounts and high thiol oxidation of peroxiredoxins in spermatozoa from infertile men. Journal of Andrology 33: 1342–1351. DOI 10.2164/jandrol.111.016162. [Google Scholar] [CrossRef]
Hariharan S, Dharmaraj S (2020). Selenium and selenoproteins: It is role in regulation of inflammation. Inflammopharmacology 28: 667–695. DOI 10.1007/s10787-020-00690-x. [Google Scholar] [CrossRef]
Hosnedlova B, Kepinska M, Skalickova S, Fernandez C, Ruttkay-Nedecky B et al. (2018). Nano-selenium and its nanomedicine applications: A critical review. International Journal of Nanomedicine 13: 2107–2128. DOI 10.2147/IJN. [Google Scholar] [CrossRef]
Huang H, An Y, Jiao W, Wang J, Li S, Teng X (2018). CHOP/caspase-3 signal pathway involves in mitigative effect of selenium on lead-induced apoptosis via endoplasmic reticulum pathway in chicken testes. Environmental Science and Pollution Research 25: 18838–18845. DOI 10.1007/s11356-018-1950-1. [Google Scholar] [CrossRef]
Ibrahim HA, Zhu Y, Wu C, Lu C, Ezekwe MO, Liao SF, Huang K (2012). Selenium-enriched probiotics improves murine male fertility compromised by high fat diet. Biological Trace Element Research 147: 251–260. DOI 10.1007/s12011-011-9308-2. [Google Scholar] [CrossRef]
Ingold I, Aichler M, Yefremova E, Roveri A, Buday K et al. (2015). Expression of a catalytically inactive mutant form of glutathione peroxidase 4 (Gpx4) confers a dominant-negative effect in male fertility. Journal of Biological Chemistry 290: 14668–14678. DOI 10.1074/jbc.M115.656363. [Google Scholar] [CrossRef]
Khurana A, Tekula S, Saifi MA, Venkatesh P, Godugu C (2019). Therapeutic applications of selenium nanoparticles. Biomedicine & Pharmacotherapy 111: 802–812. DOI 10.1016/j.biopha.2018.12.146. [Google Scholar] [CrossRef]
Labunskyy VM, Hatfield DL, Gladyshev VN (2014). Selenoproteins: Molecular pathways and physiological roles. Physiological Reviews 94: 739–777. DOI 10.1152/physrev.00039.2013. [Google Scholar] [CrossRef]
Liu C, Fu Y, Li CE, Chen T, Li X (2017a). Phycocyanin-Functionalized selenium nanoparticles reverse palmitic Acid-Induced pancreatic beta cell apoptosis by enhancing cellular uptake and blocking reactive oxygen species (ROS)-Mediated mitochondria dysfunction. Journal of Agricultural and Food Chemistry 65: 4405–4413. DOI 10.1021/acs.jafc.7b00896. [Google Scholar] [CrossRef]
Liu HJ, Qin Y, Zhao ZH, Zhang Y, Yang JH et al. (2020). Lentinan-functionalized selenium nanoparticles target tumor cell mitochondria via TLR4/TRAF3/MFN1 pathway. Theranostics 10: 9083–9099. DOI 10.7150/thno.46467. [Google Scholar] [CrossRef]
Liu L, He Y, Xiao Z, Tao W, Zhu J, Wang B, Liu Z, Wang M (2017b). Effects of selenium nanoparticles on reproductive performance of male Sprague-Dawley rats at supranutritional and nonlethal levels. Biological Trace Element Research 180: 81–89. DOI 10.1007/s12011-017-0980-8. [Google Scholar] [CrossRef]
Liu Y, Zhao J, Zhao Y, Zong S, Tian Y et al. (2019). Therapeutic effects of lentinan on inflammatory bowel disease and colitis-associated cancer. Journal of Cellular and Molecular Medicine 23: 750–760. DOI 10.1111/jcmm.13897. [Google Scholar] [CrossRef]
Lombardo F, Fiducia M, Lunghi R, Marchetti L, Palumbo A, Rizzo F, Koverech A, Lenzi A, Gandini L (2012). Effects of a dietary supplement on chronic pelvic pain syndrome (Category IIIAleucocytospermia and semen parameters. Andrologia 44: 672–678. DOI 10.1111/j.1439-0272.2011.01248.x. [Google Scholar] [CrossRef]
Maiorino M, Conrad M, Ursini F (2018). GPx4, lipid peroxidation, and cell death: Discoveries, rediscoveries, and open issues. Antioxid Redox Signal 29: 61–74. DOI 10.1089/ars.2017.7115. [Google Scholar] [CrossRef]
Qazi IH, Angel C, Yang H, Zoidis E, Pan B et al. (2019). Role of selenium and selenoproteins in male reproductive function: A review of past and present evidences. Antioxidants 8: 1–36. DOI 10.3390/antiox8080268. [Google Scholar] [CrossRef]
Rayman MP (2020). Selenium intake, status, and health: A complex relationship. Hormones 19: 9–14. DOI 10.1007/s42000-019-00125-5. [Google Scholar] [CrossRef]
Shalini S, Bansal MP (2007). Alterations in selenium status influences reproductive potential of male mice by modulation of transcription factor NFkappaB. Biometals 20: 49–59. DOI 10.1007/s10534-006-9014-2. [Google Scholar] [CrossRef]
Takeshima T, Usui K, Mori K, Asai T, Yasuda K, Kuroda S, Yumura Y (2021). Oxidative stress and male infertility. Nature Reviews Urology 20: 41–52. DOI 10.1002/rmb2.12353. [Google Scholar] [CrossRef]
Wadhwani SA, Shedbalkar UU, Singh R, Chopade BA (2016). Biogenic selenium nanoparticles: Current status and future prospects. Applied Microbiology and Biotechnology 100: 2555–2566. DOI 10.1007/s00253-016-7300-7. [Google Scholar] [CrossRef]
Wang L, Li C, Huang Q, Fu X (2019). Biofunctionalization of selenium nanoparticles with a polysaccharide from Rosa roxburghii fruit and their protective effect against H2O2-induced apoptosis in INS-1 cells. Food & Function 10: 539–553. DOI 10.1039/C8FO01958D. [Google Scholar] [CrossRef]
Xu C, Qiao L, Ma L, Guo Y, Dou X, Yan S, Zhang B, Roman A (2019a). Biogenic selenium nanoparticles synthesized by Lactobacillus casei ATCC 393 alleviate intestinal epithelial barrier dysfunction caused by oxidative stress via Nrf2 signaling-mediated mitochondrial pathway. International Journal of Nanomedicine 14: 4491–4502. DOI 10.2147/IJN.S199193. [Google Scholar] [CrossRef]
Xu C, Qiao L, Ma L, Yan S, Guo Y, Dou X, Zhang B, Roman A (2019b). Biosynthesis of Polysaccharides-Capped selenium nanoparticles using lactococcus lactis NZ9000 and their antioxidant and anti-inflammatory activities. Frontiers in Microbiology 10: 1–12. DOI 10.3389/fmicb.2019.01632. [Google Scholar] [CrossRef]
Zhang J, Wang X, Vikash V, Ye Q, Wu D, Liu Y, Dong W (2016). ROS and ROS-Mediated cellular signaling. Oxidative Medicine and Cellular Longevity 2016: 1–18. DOI 10.1155/2016/4350965. [Google Scholar] [CrossRef]
Zhang X, Gan X, Q. E, Zhang Q, Ye Y et al. (2019). Ameliorative effects of nano-selenium against NiSO4-induced apoptosis in rat testes. Toxicology Mechanisms and Methods 29: 467–477. DOI 10.1080/15376516.2019.1611979. [Google Scholar] [CrossRef]
Zini A, Garrels K, Phang D (2000). Antioxidant activity in the semen of fertile and infertile men. Urology 55: 922–926. DOI 10.1016/S0090-4295(00)00453-2. [Google Scholar] [CrossRef]
Zorov DB, Juhaszova M, Sollott SJ (2014). Mitochondrial reactive oxygen species (ROS) and ROS-induced ROS release. Physiological Reviews 94: 909–950. DOI 10.1152/physrev.00026.2013. [Google Scholar] [CrossRef]
Cite This Article
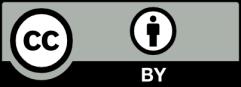