Open Access
ARTICLE
Hyperglycemia-induced myocardial fibrosis may be associated with pyroptosis and apoptosis of cardiomyoctes in diabetic mice
1 Department of Anesthesiology, The First Affiliated Hospital of Anhui Medical University, Hefei, 230022, China
2 Ambulatory Surgery Center, The First Affiliated Hospital of Anhui Medical University, Hefei, 230022, China
* Corresponding Author: YAO LU. Email:
BIOCELL 2023, 47(2), 393-400. https://doi.org/10.32604/biocell.2023.024944
Received 15 June 2022; Accepted 25 July 2022; Issue published 18 November 2022
Abstract
Myocardial fibrosis is an important manifestation of diabetic cardiomyopathy. This study investigated the potential mechanism of diabetic myocardial fibrosis. Male C57BL/6J and db/db mice aged 8 weeks were randomly divided into the diabetic (DB) and control groups. At 20 weeks, the mouse heart was harvested and subjected to hematoxylin-eosin staining (HE) and Masson staining to investigate the degree of fibrosis. The expressions of transforming growth factor-beta 1 (TGF-β1), collagen-III, B-cell lymphoma-2 (Bcl2), Bcl2-associated X protein (Bax), cleaved gasdermin D (GSDMD), cysteinyl aspartate specific proteinase-1 (caspase-1), apoptosis-associated speck-like protein containing a CARD (ASC), and nucleotide-binding oligomerization domain (NOD)-like receptor 3 (NLRP3) were measured by western blotting. Immunohistochemistry and TdT-mediated dUTP nick end labeling (TUNEL) staining were performed to analyze the development of apoptosis and pyroptosis. A significant increase in body weight and blood glucose in the DB group was observed. Myocardial pathological injury, fibrosis, apoptosis, and pyroptosis were more obvious and serious in the DB group. The expression of anti-apoptotic Bcl2 significantly decreased, while the expression levels of pro-apoptotic Bax, caspase-3, and pyroptosis-related proteins, such as cleaved GSDMD, and caspase-1 in the DB group were significantly increased. Pyroptosis and apoptosis were probably the main mechanisms that caused myocardial fibrosis in mice with diabetes.Keywords
Diabetes is a disease that mainly characterizes high blood glucose levels, and high prevalence and mortality rates. The overall global incidence of diabetes is predicted to increase from 451 million (age 18–99 years) in 2017 to 693 million by 2035 (Cho et al., 2018). It is usually accompanied by serious complications, especially those associated with the cardiovascular system, such as diabetic cardiomyopathy (DCM) (Ling et al., 2020; Rahbarghazi et al., 2021), which is one of the leading causes of death in individuals with type 2 diabetes (Brown et al., 2010). DCM is characterized by fibrosis, with functional and pathological damage to the myocardium. Cardiac fibrosis may weaken myocardial compliance, trigger arrhythmic events, and induce eventually heart failure (Zhang et al., 2018). The underlying molecular mechanisms related to the development of DCM are complex, involving myocardial fibrosis. Furthermore, the molecular basis contributing to fibrosis induced by diabetes is still not fully understood.
Myocardial fibrosis is an important manifestation of DCM. Extensive studies have suggested the involvement of several overlapping pathways, such as inflammatory cytokines and growth factors, reactive oxygen species (ROS), and transforming growth factor-β (TGF-β) signaling pathways, may be involved in cardiac fibrosis. The activation of the TGF-β signaling pathway accompanied by the deposition of structural extracellular matrix proteins in the cardiac interstitium is likely a more significant pathway (Russo and Frangogiannis, 2016). Studies have shown that inflammatory cytokines tumor necrosis factor-alpha (TNF-α) and interleukin (IL)-1β could exert several effects on the modulation of fibroblast phenotype and proliferation by regulating the TGF-β1/Smad signaling pathway, thereby enhancing collagen synthesis and inducing fibrosis (Guo et al., 2016; Saxena et al., 2013; Venkatachalam et al., 2009). Consequently, both apoptosis and pyroptosis exert several actions that may be crucial in myocardial hypertrophy and fibrosis. Another study has shown that nod-like receptor protein 3 (NLRP3) inflammasome, a vital molecule in pyroptosis, modulates the death of cardiomyocytes and activation of fibroblasts in DCM, including myocardial structure and function disorder in diabetes (Luo et al., 2017). Thus, pyroptosis and apoptosis lead to severe fibrotic damage. This study further reveals the significance of TGF-β in fibrosis and explores the relationship between pyroptosis, apoptosis, and TGF-β.
Apoptosis and pyroptosis are manifestations of programmed cell death. Pyroptosis, a newly discovered pro-inflammatory programmed cell death, is mainly mediated by inflammatory cytokines by activating caspase-1/4/11 and polymerizing gasdermins, such as gasdermin D (GSDMD), resulting in cell membrane swelling and the formation of gaps and perforation; then, the inflammatory response may be enlarged (Ruhl et al., 2018). Pyroptosis is associated with the generation of several pro-inflammatory cytokines and inflammasomes, such as IL-18 and NLRP3, that usually cause severe damage (Tsuchiya, 2020; Wang et al., 2022). Furthermore, myocardial fibrosis is induced by activating immune and pro-inflammatory signaling pathways (Saxena et al., 2015), and attenuated by downregulating the inflammatory response (Bao et al., 2018). The process of apoptosis is programmed cell death and is responsible for the stability of the internal environment (Fuchs and Steller, 2011). Numerous studies have highlighted that apoptosis contributes to the formation of fibrosis in DCM (Ares-Carrasco et al., 2009), and is a major determinant of adverse remodeling in pathological hypertrophies (Diwan et al., 2008).
In this study, we explored and demonstrated the potential mechanism of myocardial injury, consequently resulting in fibrosis, in mice with type 2 diabetes mellitus. Both apoptosis and pyrosis might be involved in mediating cardiomyocyte injury and myocardial fibrosis in mice with type 2 diabetes. This finding provides a new idea for the treatment of diabetic cardiomyopathy.
Animals and experimental protocol
All animal experiments were carried out in line with the guidelines for laboratory animal care and use of the National Institutes of Health, and were approved by the animal care and use ethics committee of Anhui Medical University. All experimental procedures were implemented on the ethical guidelines for the study of experimental pain in conscious animals.
Male C57BL/6J (n = 7) and –db/db mice (n = 8) aged 8 weeks were obtained from the GemPharmatech (Nanjing, China) and categorized into two groups. Male C57BL/6J mice without diabetes matched by age were used as the control group. Animals were acclimated 2 weeks before the study and housed in groups of three mice per cage, with a daily cycle of 12 h of light and darkness each. Food (Provincial Experimental Animal Center, Hefei, China) and water were given, ad libitium except during the fasting period. Each cage contained nesting materials and absorbent bedding. Blood glucose levels and weight were measured once every 2 weeks. Animals exhibiting both obesity (25 grams) and pronounced hyperglycemia (200 mg/dL) were considered as overt obese-diabetics. In the final period of treatment, overnight-fasted mice were euthanized under anesthesia with the abdominal injection of isopentobarbital. After blood samples were collected, the heart was removed for further experiments.
Hematoxylin and eosin (H&E) and immunohistochemistry (IHC) staining
The hearts were excised and subjected to H&E and IHC staining. After being processed with sequential clearing and dehydrating steps, the myocardium was embedded in paraffin blocks. H&E staining was performed on 5-μm slices to evaluate the myocardial injury. IHC staining was performed to measure the expression of caspase-3 (Proteintech, China), NLRP3 (Affinity, China), and caspase-1 (Proteintech, China) in the myocardium. An optical microscope (Olympus, Tokyo, Japan) was used for acquiring images. All primary antibodies for IHC staining were purchased from Wuhan Servicebio Technology (Wuhan, China).
Terminal deoxynucleotidyl transferase dUTP nick end labeling (TUNEL) staining
The hearts were fixed with formaldehyde, embedded in paraffin, and sectioned. Cardiomyocyte apoptosis was assessed in the sections by TUNEL staining. The sections were dewaxed, hydrated, and permeabilized. The TUNEL reaction solution and converter pod were added, followed by reacting with 3,3-′diaminobenzidine substrate of horseradish peroxidase to develop color. Finally, the sections were observed under microscopy.
Cardiac tissues from mice were fixed with 10% formaldehyde, embedded in paraffin, and sectioned. The sections were routinely dewaxed in water and stained with Weigert’s iron hematoxylin staining solution for 5–10 min, Masson blue staining solution for 3–5 min, Ponceau fuchsin staining solution for 5–10 min, and aniline blue staining solution for 1–2 min. During the dyeing process, the prepared weak acid working solution and distilled water were used for washing as needed. Following rapid dehydration with ethanol, the sections were permeabilized with xylene and sealed with neutral gum. Images of the stained sections were captured using a light microscope.
Radio-Immune Precipitation Assay lysate (Beyotime, Shanghai, China) was used to obtain total protein, 100 μg of which was segregated with sodium dodecyl sulfate–polyacrylamide gel electrophoresis and transferred onto polyvinylidene difluoride membranes. Tris-buffered saline with Tween 20 (TBST) containing 5% skim milk was used to incubate the membrane for 1 h. Then, the membranes were incubated with primary antibodies, including anti-TGF-β1 (1/1000, 21898-1-AP, Proteintech, China), anti-collagen-III (1/1000, 22734-1-AP, Proteintech, China), anti-B-cell lymphoma-2 (Bcl2) (ab194583, 1/1000, Abcam, Cambridge, UK), anti-Bcl2 associated X protein (Bax) (ab32503, 1/1000, Abcam, Cambridge, UK), anti-caspase-1 (22915-1-AP, 1/1000, Proteintech, China), anti-NLRP3 (DF7438, 1:1000, Affinity), cleaved GSDMD (AF4012, 1/1000, Affinity), and apoptosis-associated speck-like protein containing a CARD (ASC) at 4°C overnight. The membranes were washed thrice with TBST and incubated with anti-rabbit IgG H&L secondary antibody (ab6721, 1:2000, Abcam, Cambridge, UK) at room temperature for 1.5 h. After washing thrice with TBST, the membranes were subjected to color reaction using ECL Plus Western blotting from Life Technology, and GAPDH was detected as the control.
All data were expressed as mean ± SD. Data analysis was performed using Prism 8.0.1 (GraphPad, San Diego, California). Unpaired Student’s t-test was used for comparisons between two groups, and one-way ANOVA and the Tukey test were used for comparisons among multiple groups. All experiments were repeated at least three times under the same condition. p-values of less than 0.05 were used to denote statistical significance.
Hyperglycemia induces myocardial fibrosis in diabetic mice
One mouse in the DB group was excluded because its body weight did not meet the standard of type-2 diabetes model. During the experimental process, body weight and blood glucose levels in both groups showed a significant increase (Figs. 1A–1C), in which the body weight, blood glucose levels, and food intake of the –db/db mice were significantly higher than those of the control group (p < 0.001). The average body weight and blood glucose concentration were 57.63 g and 24.69 mmol/L, respectively, in the DB group, and 24.69 g and 7.36 mmol/L, respectively, in the control group. Water intake was more in the DB group than that in the control group (Fig. 1D), and the difference was significant between 8 and 12 weeks. According to the data of blood glucose and body weight, a diabetes model was successfully and steadily established. The results are shown in Fig. 2. The myocardium of the control group had a clear texture, organized arrangement of myocardium cells, uniform distribution of nuclei, and no pathological structural changes, such as degeneration and necrosis. Conversely, H&E staining results indicated that diabetic mice had red cytoplasm and nuclear pyknosis; moreover, the arrangement of myocardial fibers was disordered, and the number of normal myocardium cells was decreased. To further observe the situation and degree of myocardial fibrosis caused by diabetes, the heart tissues of 20-week-old diabetic mice were removed for Masson staining (Fig. 2B): compared with the control group, diabetic mice showed significant collagen deposition in the myocardial interstitium and around small blood vessels, and the number and degree of fibrosis were significantly more severe than those in the control group. The expression of TGF-β1 and collagen-III associated with diabetic heart fibrosis was significantly increased, as shown by western blotting of –db/db mice, and the difference was statistically significant (Figs. 2C and 2D). These results suggest that high blood glucose levels in diabetic mice promote the generation of myocardial fibrosis.
Figure 1: Weight, blood glucose, food, and water intake in the diabetic (DB) and control groups. (A–D) Blood glucose level, body weight, food intake, and water intake are different between C57BL/6J and –db/db mice. The DB group represents –db/db mice, whereas the control group represents C57BL/6J mice. Data are presented as mean ± SD (n = 7 per group). **p < 0.01, ***p < 0.001 represent significant differences between the control and DB groups.
Figure 2: Myocardial pathological injury and fibrosis in the diabetic (DB) and control groups. (A, B) Representative images of hematoxylin and eosin, and Masson-stained heart sections. Magnification ×400. The scale bar represents 20 μm. (C, D) The protein expression of transforming growth factor-β1 (TGF-β1) and collagen-3 in the hearts of mice was determined by Western blotting. Quantitative analysis of TGF-β1 and collagen-3 for western blotting (n = 3 per group). Data are presented as mean ± SD. *p < 0.05 represent significant differences between the control and DB groups.
Cardiomyocytes apoptosis occurred in diabetic mice
To further investigate the levels of cardiomyocyte apoptosis in –db/db and c57 mice, TUNEL staining and expression of anti-apoptotic Bcl2, pro-apoptotic Bax, and caspase-3 were examined in this study. TUNEL staining of myocardial tissue showed a significantly elevated number of apoptotic cells, decreased number of normal cells, and more disordered arrangement of cells in the DB group than in the control group (Fig. 3A). IHC staining revealed that a large proportion of cells were caspase-3 positive, which corroborated the staining intensity in the DB group (Fig. 3B). Western blotting showed that the expression of apoptosis-related proteins, such as Bax, and caspase-3, was significantly increased; the expression levels in the DB were higher than those in the control group, with statistically significant differences (Fig. 3C). Bcl2, Bax, and caspase-3 were critical in apoptosis. Studies have shown that apoptosis in –db/db mice was promoted by downregulating the expression of Bcl2/Bax and increasing the generation of caspase-3. These regulations and alterations convincingly demonstrated that apoptosis is crucial in the progression of diabetes mellitus.
Figure 3: Myocardial pathological injury and fibrosis in the diabetic (DB) and control groups. (A, B) Representative images of terminal deoxynucleotidyl transferase dUTP nick end labeling (TUNEL)-stained and immunohistochemistry–stained caspase-3 of heart sections. Magnification ×400. The scale bar represents 100 μm. (C) The protein expression of Bcl2 and Bax in the hearts of mice was determined by Western blotting (n = 3 per group). Data are presented as mean ± SD. *p < 0.05 represent significant differences between the control and DB groups.
Myocardial pyroptosis in mice with diabetes mellitus
Pyroptosis is a programmed cell death, which involves complex inflammatory response and multiple signaling pathways. It plays a pivotal role in cell renewal and repair; the essential significance of pyroptosis was also observed in our study. IHC staining showed that a large proportion of cells were caspase-1 and NLRP3 positive, which corroborated with staining intensity in the DB group (Figs. 4A and 4B). The expression levels of pyroptosis-related genes or proteins detected by western blotting revealed significantly increased expression of ASC, cleaved GSDMD, and caspase-1 in –db/db mice, with statistically significant differences (Fig. 4). GSDMD, as a substrate for caspase-1 is the executor of pyroptosis. The maturation of IL-1β is induced by caspase-1, which also enhances the extracellular release of IL-1β by promoting GSDMD cleavage to form cellular pores. IL-1β subsequently mediates pyroptosis through the GSDMD cell pores. Thus, NLRP3 and ASC are the inflammasomes that mediate cell pyroptosis. All these cardiac cells are seriously damaged by the inflammatory response and cytokines, as indicated by the rapidity of this process. Therefore, the increase in these proteins suggests that pyroptosis is significant in myocardial damage in diabetic mice.
Figure 4: Cardiomyocyte pyroptosis in –db/db and c57 mice. (A, B) Representative immunohistochemistry staining of caspase-1 and NLRP3 of the heart section. Magnification ×400. The scale bar represents 100 μm. (C, D) The expression of NLRP3, cleaved GSDMD, caspase-1, and ASC protein in the hearts of mice was determined by western blotting (n = 4 per group). Data are presented as mean ± SD. **p < 0.01, and *p < 0.05 represent significant differences between the control and DB groups.
DCM is a specific disease that involves myocardium abnormalities that cannot be explained by hypertension, coronary atherosclerotic heart disease, valvular heart disease, and other heart diseases. It is regarded as one of the main causes of death in individuals with diabetes, especially those with type 2 diabetes (Dillmann, 2019; Ling et al., 2020; Paolillo et al., 2019). Considering the metabolic disorders and microvascular lesions, this disease leads to extensive focal necrosis of the myocardium and subclinical cardiac dysfunction, and eventually progresses to heart failure, arrhythmia, and cardiogenic shock (Jia et al., 2018). Myocardial fibrosis is a remarkable manifestation of DCM. Therefore, the research on myocardial fibrosis is necessary and meaningful; the results of our experiment are as follows. The weight and blood glucose levels and water and food intake were significantly higher in the DB group than those in the control group. Likewise, the degree of myocardial fibrosis was more severe in the DB group than that in the control group. In addition, the expression of anti-apoptotic Bcl2 decreased, and that of pro-apoptotic Bax, caspase-3, cleaved GSDMD, and caspase-1 in 20-week-old –db/db mice increased.
The difference between H&E and Masson staining in this study indicated serious fibrosis caused by high blood glucose and myocardial energy metabolic abnormalities. Studies have reported that the increase in fat metabolism and reduced metabolism of sugar and lactate could lead to the accumulation of free fatty acid, oxidative stress, and mitochondrial damage, causing superfluous production of collagen and cardiac fibrosis (Kanamori et al., 2021; Tallquist and Molkentin, 2017; Voulgari et al., 2010). Therefore, fibrosis is a major and severely damaging condition, eventually resulting in disordered cardiac structure and function. The activation of the TGF-β1/Smad pathway and the production of redundant collagen have been often found as the main pathological mechanisms of myocardial fibrosis (Kanamori et al., 2021; Tallquist and Molkentin, 2017; Voulgari et al., 2010). Similarly, this study has also exhibited an increase in TGF-β1 and collagen-III protein expression in –db/db mice with diabetes mellitus. In addition, cardiac myocyte reduction, inflammatory cell infiltration, and myocardial interstitial fibrosis have been found in other studies similar to our current staining outcomes (Hu et al., 2020; Wang et al., 2018).
The molecular basis that contributes to myocardial fibrosis in diabetic mice is complicated and is so far, poorly understood; we hypothesize that apoptosis and pyroptosis are involved in the process. We found apoptosis and pyroptosis as the two major types of programmed cell death and considered the terminal pathways of cardiomyocytes during myocardial fibrosis. Apoptosis is one of the fastest forms of cell death and mainly relies on caspase proteolysis. Signaling molecules, including Bcl2, pro-apoptotic Bax, and TNF-α have been often reported to be involved in apoptosis associated with myocardial fibrosis (Sulistiyowati et al., 2020; Wu et al., 2019). Accordingly, perivascular and interstitial fibrosis in cardiac muscle fibers of rats with diabetes was improved by decreasing and inhibiting inflammatory response and functions of apoptosis-related proteins, such as p53, Bax, cytochrome c, and caspase-3 (Sulistiyowati et al., 2020; Wu et al., 2019). In another study, the reduction of TNF-α serum levels was beneficial in alleviating myocardial fibrosis and the signal genes of apoptosis were significantly upregulated (Ye et al., 2020). Furthermore, the levels of pro-apoptotic Bax protein differed significantly between the control and DB groups, and the ratio of Bcl2 to Bax decreased. The destructive changes in apoptosis might cause the death of myocardial cells and result in cardiac fibrosis.
To further investigate the mechanism of myocardial fibrosis essentially, the genes and proteins associated with pyroptosis were examined and analyzed. Overwhelming evidence has shown that pyroptosis plays a critical role in the development of fibrosis. Xiao et al. (2020) reported that the repression of NLRP3 inflammasome-mediated pyroptosis was expected to be pivotal in myocardial injury. Pyroptosis is a critical immune-inflammatory death in fibrosis and heart failure including mitochondrial dysfunction, lysosomal rupture, and ROS generation. Wang et al. (2019) suggested that fibrosis diabetic cardiomyopathy could be alleviated by inhibiting cardiac fibrosis and NLRP3 inflammasome activation. NLRP3, IL-1β, IL-18, and caspase-1 are fundamental molecules in the activation of pyroptosis (Fig. 5). In our current study, the same results also showed significantly increased expression of pyroptosis-associated proteins, such as GSDMD and caspase-1in DB mice aged 20 weeks.
Figure 5: A schematic showing that diabetic fibrosis involves apoptosis and pyroptosis.
Pyroptosis is a newly found form of programmed necrotic cell death (Robinson et al., 2019); it is responsible for the secretion and activation of pro-inflammatory cytokines and cell lysis via high glucose stimulation and then induces inflammatory responses by releasing IL-1β and IL-18 (Robinson et al., 2019). Canonical and noncanonical are two main pathways involved in the process of pyroptosis (Fig. 5). The classical pathway that depends on caspase-1 includes various inflammasome releases and cascade reactions (Li et al., 2019). The activation of caspase-11/4 induced by bacterial lipopolysaccharide mainly involves the noncanonical pathway (Li et al., 2019). Both pathways eventually lead to activation of GSDMD and caspase-1, forming a membrane pore, thereby advancing to the cell death. Our study reveals that the expression of GSDMD and caspase-1 are significantly increased in db/db mice compared with those in the control. These results indicate the importance and effects of canonical pyroptosis pathway in the progression of myocardial fibrosis. Jeyabal et al. (2016) also showed augmented expression of NLRP3 and caspase-1 in both human diabetic heart and HG-exposed human ventricular cardiomyocytes. Inflammasome, caspase-1, IL-1β, and GSDMD were increased in the myocardium of diabetes mellitus. Consequently, the canonical pyroptosis pathway is crucial in diabetic heart damage, and our study mainly revealed the canonical pyroptosis pathway.
NLRP3 inflammasome, as a molecular marker in myocardial fibrosis, regulates cardiomyocyte death and fibroblast activation, thus leading to the structural and functional disfunction of myocardial fibrosis and the canonical pyroptosis pathway. Inflammatory response connected with pyroptosis caused by hyperglycemia is recognized to play a central role in cardiac and noncardiac diabetes complications (Bornfeldt and Tabas, 2011; Knapp et al., 2019). Apoptosis is mainly involved in the mitochondrial intrinsic and TNF-a-induced extrinsic pathways, and the intrinsic pathway is related to Bax and Bcl2 (Bornfeldt and Tabas, 2011; Knapp et al., 2019). The mice with the highest blood glucose level were selected to undergo Masson staining and western blotting simultaneously, and showed increased expressions of TGF-β1 and collagen-III, GSDMD, caspase-1, Bcl2, pro-apoptotic Bax, and cleaved Caspase 3, except for NLRP3 and ASC. Pyroptosis and apoptosis are crucial causes of fibrosis and DCM; therefore, this damage may be associated with TGF-β1 signaling pathways, and disordered expression of TGF-β1 has been associated with the development of a variety of diseases (Healy et al., 2009). Nonetheless, the specific relations and the mechanism still need more clinical and laboratory studies.
This study had some limitations. First, we only conducted animal experiments without the support of relevant cell experiments and clinical research. Second, to examine apoptosis and pyroptosis, we only assessed the role of the intrinsic mitochondrial apoptosis pathway and the classical pathway of pyroptosis, and did not explore the external and non-classical pathways. Third, the functional damage of the heart was not recorded and the results of this study were not compared with those of other studies (Healy et al., 2009). Furthermore, the specific relationship between fibrosis and pyroptosis still needs to be further studied.
Taken together, these results suggest that high blood glucose in mice with diabetes can induce myocardial fibrosis. Fibrosis is one of the striking features of diabetic cardiomyopathy. Our results suggest that apoptosis and pyroptosis of cardiomyocytes probably cause myocardial fibrosis in mice with diabetes. These results may provide a direction for further targeted therapies for DCM.
Availability of Data and Materials: The datasets generated during and/or analysed during the current study are available from the corresponding author on reasonable request.
Author Contribution: The authors confirm contribution to the paper as follows: study conception and design: YL, QW; data collection: QW, CZ; analysis and interpretation of results: QW, CZ; draft manuscript preparation: YL, QW, CZ. All authors reviewed the results and approved the final version of the manuscript.
Ethics Approval: This study were approved by the animal care and use ethics committee of Anhui Medical University (LLSC20210523, March 2021).
Funding Statement: This work is supported by the Advanced Projects of Innovation Program for the Selected Returned Overseas Chinese Scholars, Anhui Province (2020LCX022).
Conflicts of Interest: The authors declare that they have no conflicts of interest to report regarding the present study.
References
Ares-Carrasco S, Picatoste B, Benito-Martin A, Zubiri I, Sanz AB, Sánchez-Niño MD, Ortiz A, Egido J, Tuñón J, Lorenzo O (2009). Myocardial fibrosis and apoptosis, but not inflammation, are present in long-term experimental diabetes. American Journal of Physiology-Heart and Circulatory Physiology 6: 2109–2119. DOI 10.1152/ajpheart.00157.2009. [Google Scholar] [CrossRef]
Bao JW, Sun B, Ma PP, Gai YS, Sun WZ, Yu HQ, Li J (2018). Rosuvastatin inhibits inflammatory response and resists fibrosis after myocardial infarction. European Review for Medical and Pharmacological Sciences 1: 238–245. DOI 10.26355/eurrev_201801_14123. [Google Scholar] [CrossRef]
Bornfeldt KE, Tabas I (2011). Insulin resistance, hyperglycemia, and atherosclerosis. Cell Metabolism 5: 575–585. DOI 10.1016/j.cmet.2011.07.015. [Google Scholar] [CrossRef]
Brown A, Reynolds LR, Bruemmer D (2010). Intensive glycemic control and cardiovascular disease: An update. Nature Reviews Cardiology 7: 369–375. DOI 10.1038/nrcardio.2010.35. [Google Scholar] [CrossRef]
Cho NH, Shaw JE, Karuranga S, Huang Y, da Rocha Fernandes JD, Ohlrogge AW, Malanda B (2018). IDF Diabetes Atlas: Global estimates of diabetes prevalence for 2017 and projections for 2045. Diabetes Research and Clinical Practicet 138: 271–281. DOI 10.1016/j.diabres.2018.02.023. [Google Scholar] [CrossRef]
Dillmann WH (2019). Diabetic cardiomyopathy. Circulation Research 8: 1160–1162. DOI 10.1161/CIRCRESAHA.118.314665. [Google Scholar] [CrossRef]
Diwan A, Wansapura J, Syed FM, Matkovich SJ, Lorenz JN, Dorn GW (2008). Nix-mediated apoptosis links myocardial fibrosis, cardiac remodeling, and hypertrophy decompensation. Circulation 3: 396–404. DOI 10.1161/CIRCULATIONAHA.107.727073. [Google Scholar] [CrossRef]
Fuchs Y, Steller H (2011). Programmed cell death in animal development and disease. Cell 4: 742–758. DOI 10.1016/j.cell.2011.10.033. [Google Scholar] [CrossRef]
Guo X, Xue M, Li CJ, Yang W, Wang SS et al. (2016). Protective effects of triptolide on TLR4 mediated autoimmune and inflammatory response induced myocardial fibrosis in diabetic cardiomyopathy. Journal of Ethnopharmacology 193: 333–344. DOI 10.1016/j.jep.2016.08.029. [Google Scholar] [CrossRef]
Healy J, Dionne J, Belanger H, Lariviere M, Beaulieu P, Labuda D, Sinnett D (2009). Functional impact of sequence variation in the promoter region of TGFB1. International Journal of Cancer 125: 1483–1489. DOI 10.1002/ijc.24526. [Google Scholar] [CrossRef]
Hu J, Lu X, Zhang X, Shao X, Wang Y, Chen J, Zhao B, Li S, Xu C, Wei C (2020). Exogenous spermine attenuates myocardial fibrosis in diabetic cardiomyopathy by inhibiting endoplasmic reticulum stress and the canonical Wnt signaling pathway. Cell Biology International 8: 1660–1670. DOI 10.1002/cbin.11360. [Google Scholar] [CrossRef]
Jeyabal P, Thandavarayan RA, Joladarashi D, Suresh BS, Krishnamurthy S, Bhimaraj A, Youker KA, Kishore R, Krishnamurthy P (2016). MicroRNA-9 inhibits hyperglycemia-induced pyroptosis in human ventricular cardiomyocytes by targeting ELAVL1. Biochemical and Biophysical Research Communications 471: 423–429. DOI 10.1016/j.bbrc.2016.02.065. [Google Scholar] [CrossRef]
Jia G, Whaley-Connell A, Sowers JR (2018). Diabetic cardiomyopathy: A hyperglycaemia- and insulin-resistance-induced heart disease. Diabetologia 1: 21–28. DOI 10.1007/s00125-017-4390-4. [Google Scholar] [CrossRef]
Kanamori H, Naruse G, Yoshida A, Minatoguchi S, Watanabe T et al. (2021). Morphological characteristics in diabetic cardiomyopathy associated with autophagy. Journal of Cardiology 1: 30–40. DOI 10.1016/j.jjcc.2020.05.009. [Google Scholar] [CrossRef]
Knapp M, Tu X, Wu R (2019). Vascular endothelial dysfunction, a major mediator in diabetic cardiomyopathy. Acta Pharmacologica Sinica 1: 1–8. DOI 10.1038/s41401-018-0042-6. [Google Scholar] [CrossRef]
Ling W, Huang Y, Huang YM, Fan RR, Sui Y, Zhao HL (2020). Global trend of diabetes mortality attributed to vascular complications, 2000–2016. Cardiovascular Diabetology 19: 182. DOI 10.1186/s12933-020-01159-5. [Google Scholar] [CrossRef]
Li X, Li Z, Li B, Zhu X, Lai X (2019). Klotho improves diabetic cardiomyopathy by suppressing the NLRP3 inflammasome pathway. Life Science 234: 116773. DOI 10.1016/j.lfs.2019.116773. [Google Scholar] [CrossRef]
Luo B, Huang F, Liu Y, Liang Y, Wei Z, Ke H, Zeng Z, Huang W, He Y (2017). NLRP3 Inflammasome as a molecular marker in diabetic cardiomyopathy. Frontiers in Physiology 25: 519. DOI 10.3389/fphys.2017.00519. [Google Scholar] [CrossRef]
Paolillo S, Marsico F, Prastaro M, Renga F, Esposito L et al. (2019). Diabetic cardiomyopathy: Definition, diagnosis, and therapeutic implications. Heart Failure Clinics 3: 341–347. DOI 10.1016/j.hfc.2019.02.003. [Google Scholar] [CrossRef]
Rahbarghazi A, Siahkouhian M, Rahbarghazi R, Ahmadi M, Bolboli L, Mahdipour M, Haghighi L, Hassanpour M, Sokouti Nasimi F, Keyhanmanesh R (2021). Melatonin and prolonged physical activity attenuated the detrimental effects of diabetic condition on murine cardiac tissue. Tissue & Cell 69: 101486. DOI 10.1016/j.tice.2021.101486. [Google Scholar] [CrossRef]
Robinson N, Ganesan R, Hegedus C, Kovacs K, Kufer TA, Virág L (2019). Programmed necrotic cell death of macrophages: Focus on pyroptosis, necroptosis, and parthanatos. Redox Biology 26: 101239. DOI 10.1016/j.redox.2019.101239. [Google Scholar] [CrossRef]
Ruhl S, Shkarina K, Demarco B, Heilig R, Santos JC, Broz P (2018). ESCRT-dependent membrane repair negatively regulates pyroptosis downstream of GSDMD activation. Science 362: 956–960. DOI 10.1126/science.aar7607. [Google Scholar] [CrossRef]
Russo I, Frangogiannis NG (2016). Diabetes-associated cardiac fibrosis: Cellular effectors, molecular mechanisms and therapeutic opportunities. Journal of Molecular and Cellular Cardiology 90: 84–93. DOI 10.1016/j.yjmcc.2015.12.011. [Google Scholar] [CrossRef]
Saxena A, Chen W, Su Y, Rai V, Uche OU, Li N, Frangogiannis NG (2013). IL-1 induces proinflammatory leukocyte infiltration and regulates fibroblast phenotype in the infarcted myocardium. The Journal of Immunology 9: 838–848. DOI 10.4049/jimmunol.1300725. [Google Scholar] [CrossRef]
Saxena A, Shinde AV, Haque Z, Wu YJ, Chen W, Su Y, Frangogiannis NG (2015). The role of Interleukin Receptor Associated Kinase (IRAK)-M in regulation of myofibroblast phenotype in vitro, and in an experimental model of non-reperfused myocardial infarction. Journal of Molecular and Cellular Cardiology 89: 223–231. DOI 10.1016/j.yjmcc.2015.11.001. [Google Scholar] [CrossRef]
Sulistiyowati I, Yunus J, Sari D, Arfian N (2020). Upregulation of p16, Bax and Bcl-2 mRNA expression associated with epithelial apoptosis and myofibroblast proliferation in kidney fibrosis model in mice. Malawi Medical Journal 2: 7–44. DOI 10.21315/mjms2020.27.2.4. [Google Scholar] [CrossRef]
Tallquist MD, Molkentin JD (2017). Redefining the identity of cardiac fibroblasts. Nature Reviews Cardiology 8: 484–491. DOI 10.1038/nrcardio.2017.57. [Google Scholar] [CrossRef]
Tsuchiya K (2020). Inflammasome-associated cell death: Pyroptosis, apoptosis, and physiological implications. Microbiology and Immunology 4: 252–269. DOI 10.1111/1348-0421.12771. [Google Scholar] [CrossRef]
Venkatachalam K, Venkatesan B, Valente AJ, Melby PC, Nandish S, Reusch JE, Clark RA, Chandrasekar B (2009). WISP1, a pro-mitogenic, pro-survival factor, mediates tumor necrosis factor-alpha (TNF-α)-stimulated cardiac fibroblast proliferation but inhibits TNF-alpha-induced cardiomyocyte death. The Journal of Biological Chemistry 21: 14414–14427. DOI 10.1074/jbc.M809757200. [Google Scholar] [CrossRef]
Voulgari C, Papadogiannis D, Tentolouris N (2010). Diabetic cardiomyopathy: From the pathophysiology of the cardiac myocytes to current diagnosis and management strategies. Vascular Health and Risk Management 6: 883–903. DOI 10.2147/VHRM.S11681. [Google Scholar] [CrossRef]
Wang P, Li G, Gao L, Zhao C (2022). Human β-defensin 2 enhances IL-1β production and pyroptosis through P2X7-mediated NLRP3 expression in macrophages. BIOCELL 46: 1197–1207. DOI 10.32604/biocell.2022.016607. [Google Scholar] [CrossRef]
Wang XT, Gong Y, Zhou B, Yang JJ, Cheng Y, Zhao JG, Qi MY (2018). Ursolic acid ameliorates oxidative stress, inflammation and fibrosis in diabetic cardiomyopathy rats. Biomedicine & Pharmacotherapy 97: 1461–1467. DOI 10.1016/j.biopha.2017.11.032. [Google Scholar] [CrossRef]
Wang Y, Li H, Li Y, Zhao Y, Xiong F et al. (2019). Coriolus versicolor alleviates diabetic cardiomyopathy by inhibiting cardiac fibrosis and NLRP3 inflammasome activation. Phytotherapy Research 33: 2737–2748. DOI 10.1002/ptr.6448. [Google Scholar] [CrossRef]
Wu B, Wang R, Li S, Wang Y, Song F, Gu Y, Yuan Y (2019). Antifibrotic effects of Fraxetin on carbon tetrachloride-induced liver fibrosis by targeting NF-kappaB/IkappaBalpha, MAPKs and Bcl-2/Bax pathways. Pharmacological Reports 3: 409–416. DOI 10.1016/j.pharep.2019.01.008. [Google Scholar] [CrossRef]
Xiao B, Huang X, Wang Q, Wu Y (2020). Beta-asarone alleviates myocardial ischemia-reperfusion injury by inhibiting inflammatory response and NLRP3 inflammasome mediated pyroptosis. Biological and Pharmaceutical Bulletin 43: 1046–1051. DOI 10.1248/bpb.b19-00926. [Google Scholar] [CrossRef]
Ye Y, Birnbaum Y, Widen SG, Zhang Z, Zhu S, Bajaj M, Chen H (2020). Acupuncture reduces hypertrophy and cardiac fibrosis, and improves heart function in mice with diabetic cardiomyopathy. Cardiovascular Drugs and Therapy 34: 835–848. DOI 10.1007/s10557-020-07043-4. [Google Scholar] [CrossRef]
Zhang Y, Cui L, Guan G, Wang J, Qiu C, Yang T, Guo Y, Liu Z (2018). Matrine suppresses cardiac fibrosis by inhibiting the TGFbeta/Smad pathway in experimental diabetic cardiomyopathy. Molecular Medicine Reports 1: 1775–1781. DOI 10.3892/mmr.2017.8054. [Google Scholar] [CrossRef]
Cite This Article
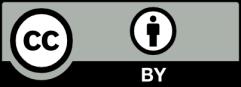