Open Access
ARTICLE
Effects of platelets on characteristics of lymphocytes cultured in vitro and optimization of adoptive immunotherapy
West China School of Basic Medical Sciences and Forensic Medicine, Department of Immunology, Sichuan University, Chengdu, China
* Corresponding Authors: PEIPEI WANG. Email: ; XIA WANG. Email:
(This article belongs to the Special Issue: Extracellular Vesicles and Cancer)
BIOCELL 2023, 47(12), 2661-2669. https://doi.org/10.32604/biocell.2023.043084
Received 21 June 2023; Accepted 09 October 2023; Issue published 27 December 2023
Abstract
Background: T lymphocytes, the main participants of cellular immunity, can express a variety of surface molecules and form different lymphocyte subsets under the induction of different factors to play the functions of immune regulation and immune killing. Studies have shown that platelets play a crucial role in maintaining the stable differentiation of lymphocytes and the balance in immunomodulation. Therefore, it is necessary to study the effect of platelets on lymphocytes in vitro to better understand the role of platelets in the immune system and broaden the application of adoptive immunotherapy. Methods: Cell counting and microscopic observation were used to detect the effect of activated platelets on lymphocyte proliferation in vitro; Flow cytometry was used to detect whether changes in platelet activity affect the proportion of lymphocyte subpopulations in vitro, and to detect differences in the expression of granzyme B; lactate dehydrogenase assay (LDH) was used to determine the difference in lymphocyte killing activity caused by platelet activity in vitro. Results: This was the first to promote lymphocyte proliferation through the expression or release of certain molecules in vitro, demonstrating that platelet activation is one of the key factors. Secondly, activated platelets or inactivated platelets promoted lymphocyte subset differentiation by enhancing the proportion of CD3+CD8+ T lymphocytes (CTL cells) but had a slight effect on the proportion of CD3+CD4+ T (Th cells) and CD4+CD25+ T lymphocytes (Treg cells). Then, it was found that either activated platelets or inactivated platelets down-regulated the proportion of natural killer (NK)T lymphocytes, while activated platelets significantly enhance the proportion of NK lymphocytes. Therefore, by further detecting the killing activity of PBMCs treated with platelets, it was found that activated platelets promoted the extensive anti-tumor activity of lymphocytes and significantly increased the expression of granzyme B. Conclusion: Our results suggest that activated platelets promote lymphocyte proliferation, optimize lymphocyte subpopulation ratio, and promote cytotoxic effect of lymphocytes in vitro, which may provide a new strategy for optimizing the adoptive immunotherapy culture system and improving its efficacy.Keywords
Platelets or thrombocytes, the smallest cellular component of the hematopoietic system, are important bioactive nuclear in the megakaryocytes series (Yan et al., 2023). Platelets express several adhesion and aggregation cell surface receptors (Sang et al., 2021; Maouia et al., 2020; Yamashita and Asada, 2023), such as the glycoprotein (GP) Ib-IX-V complex, as well as other activation receptors, including thromboxane receptors. Thromboxane affects the activation and proliferation of T cells and supports its cytotoxic effects (El Filaly et al., 2023). Platelets also contain cytoplasmic granules that express a variety of molecules, such as platelet factor 4 (PF4), regulated upon activation, normal T cell expressed and secreted (RANTES), transforming growth factor-β (TGF-β), and serotonin (Manne et al., 2017; Aslan, 2021; Mandel et al., 2022). The release of these factors impacts immune cell function in various ways. PF4, for instance, promotes monocyte survival and differentiation into macrophages (El Filaly et al., 2023; Mandel et al., 2022). RANTES, regulated upon activation of normal T-cell expressed and secreted, may function as a system mediating the attraction of monocytes, natural killer (NK) cells, and T cells (El Filaly et al., 2023; Mandel et al., 2022).
Platelets in the blood were discovered over a hundred years ago, and their hemostatic and coagulation functions have been confirmed (Sang et al., 2021). Subsequently, other physiological regulatory functions, such as angiogenesis, wound healing, immune regulation, and so on, have been gradually explored (van der Meijden and Heemskerk, 2019; Yeung et al., 2018; Li et al., 2023). Due to their immunomodulatory effect, platelets were first found to play an important role in innate immunity and immune inflammation (Claushuis et al., 2019). In acquired immunity, however, the role of platelets has been constantly reported in recent years, including the influence of antigen presentation, immune cell activation, and immune cell subsets differentiation (Nurden, 2018).
The “Janus Face” of platelets in cancer (Catani et al., 2020) was recently reported by researchers. Platelets release molecules acting on tumor cells, such as platelet-derived growth factor (PDGF) and lysophosphatidic acid (LPA), which promote tumor cell proliferation, survival, and invasion. Platelets, on the other hand, secrete other substances, such as serotonin, that can interfere with cancer development and metastasis. The stimulatory and inhibitory effects of serotonin on carcinogenesis and progression are supported by a wide body of experimental evidences (Balakrishna et al., 2021; Karmakar and Lal, 2021). In patients with cancer, thrombocytosis may lead to poor prognosis (Wang et al., 2018). A recent study revealed that inhibition of platelet-related molecules in melanoma mice could enhance the activity of T lymphocytes and slow down the growth of melanoma (Rachidi et al., 2017). This study also confirmed that joint antiplatelet drugs can enhance the effect of the adoptive immunotherapy T lymphocytes, providing a new method for tumor treatment (Rachidi et al., 2017). These findings indicate that platelets may affect tumor progression and prognosis by modulating antitumor immunity, particularly T cell-mediated immunity.
Based on the dual role of platelets in tumors, further study on the effect of platelets on T cells cultured in vitro can provide a new strategy for adoptive immunotherapy and broaden its application in tumors. Here, we report a discovery that activated platelets promote T lymphocyte proliferation in vitro, optimize the proportion of lymphocyte subsets, and enhance lymphocyte cytotoxicity. Our research suggests that activated platelets can be used as activators of adoptive immunotherapy, which provides a new strategy for optimizing the culture system of adoptive immunotherapy and improving its efficacy.
The study was conducted in accordance with the 2013 Declaration of Helsinki. Informed consent was obtained from all the volunteers. Peripheral blood samples were collected from 10 healthy volunteers. There were 4 males and 6 females, ranging in age from 23 to 55 years (median age of 29.7 years). The human lymphocyte separation solution was purchased from (Tianjin Haoyang Biotechnology, Tianjin, China). CD3 monoclonal antibody OKT3 was purchased from (CytoCares, Shanghai, China). Interleukin-2 (IL-2) and interferon-gamma (IFN-γ) were purchased from (Beijing Tongli Biotechnology, Beijing, China). Solid CaCl2 were purchased from (Beijing Solarbio Science & Technology Co., Ltd., Beijing, China). Fixation membrane buffer was purchased from (BD Biosciences, New Jersey, USA). The antibodies used in flow cytometry were purchased from (BD Biosciences, New Jersey, USA), including anti-human CD3-FITC, CD4-APC, CD8-PE, CD25-PE, CD16-APC, CD56-PE, and granzyme B-FITC. CytoTox 96 Non-radioactive Cytotoxicity Assay was purchased from Promega, Madison, USA.
Human non-small cell lung cancer cell A549 and human chronic myelocytic leukemia cell K562 were preserved in the Department of Immunology, Sichuan University. The human pancreatic cancer cell line PANC-1 was purchased from Procell Life Science& Technology, Wuhan, China. The cells were maintained in Dulbecco’s Modified Eagle’s medium or Roswell Park Memorial Institute 1640 medium (Hyclone, Utah, USA) supplemented with 10% fetal bovine serum (MHBio, Gansu, China) and cultured at 37°C in a humidified atmosphere containing 5% CO2.
Isolation of human peripheral blood mononuclear cells (PBMCs)
Peripheral blood (20 mL) was taken from volunteers, added into an anticoagulant tube, mixed evenly, and centrifuged at 500 g for 10 min. The upper plasma, after centrifugation, was collected and centrifuged at 1800 g for 15 min. After centrifugation, a portion of the plasma was collected as a nutritional component for cell culture. The lower blood cell precipitates were slowly added into ficoll paque and centrifuged at 650 g for 20 min. After centrifugation, the middle layer of lymphocytes was taken into the tube and washed thrice with saline. To the in vitro culture medium of T lymphocytes, a certain concentration of 300 U/mL interleukin-2, 50 ng/mL anti-CD3, and 1,000 U/mL human rIFN-γ was added to prepare the final “Conditioned medium”. The isolated PBMC was divided for subsequent experiments.
Separation and activation of platelets
The collected upper plasma was centrifuged at 1800 g for 15 min, and a portion of it was collected as a nutritional component for cell culture, while the remaining was divided into two parts after mixing the liquid. One part of the remaining plasma was treated with 10% CaCl2, diluted at 1:10, and incubated at 37°C for 30 min, and called “activated platelets”, and the other part was called “inactive platelets”. The activated platelets were then centrifuged at 10000 rpm for 10 min. The collected supernatant was named “platelets supernatant”, and the precipitate was named “platelets precipitate”.
The isolated PBMC (as in “Isolation of human peripheral blood mononuclear cells (PBMCs)”) was divided into five parts, treated with 1/3 or 2/3 inactive platelets, 1/3 or 2/3 activated platelets or without platelets (as in “Separation and activation of platelets”), respectively, then cultured with “Conditioned medium”. Then, PBMC-treated cells were plated in 6-well plates. Over the subsequent 1 to 10 days, the number of the cells was counted in triplicate wells, and cell growth curves were plotted over time.
The isolated PBMC was divided into three parts, treated with activated platelet precipitation, platelet supernatant or without platelets, respectively, and cultured with a “Conditioned medium”. After 10 days of induction in vitro, the number of the cells was counted in triplicate wells.
The isolated PBMC (as in “Isolation of human peripheral blood mononuclear cells (PBMCs)”) was divided into three parts: treated with platelets-free, inactive platelets, and activated platelets (as in “Separation and activation of platelets”), respectively, then cultured in the conditioned medium for 10 days.
Cells were prepared and washed with phosphate buffered saline, then stained with anti-human CD3-FITC, CD4-APC, CD8-PE, CD25-PE, CD16-APC, or CD56-PE at 4°C for 30 min, and analyzed using FACS Celesta flow cytometry (BD Biosciences, New Jersey, USA). For granzyme B-FITC staining, the cells were treated with fixation membrane buffer at 4°C for 20 min before staining and analyzed by flow cytometry. The results analyzed using FlowJo V10 statistical software (BD Biosciences, New Jersey, USA).
Lactate dehydrogenase assay (LDH)
The isolated PBMC (as mentioned in the previous section) was divided into three parts: treated with platelets-free, inactive platelets, and activated platelets (as mentioned in the previous section) respectively, then cultured in the conditioned medium for 10 days. Then, the cells treated with equal volume or the same quantity of PBMC-treated cells were taken as effector cells, and tumor cells A549, PANC-1, and K562 were taken as target cells.
Target cells and effector cells were prepared in a 1:10 ratio according to the manufacturer, and then added to a 96-well plate. Cell death was detected after co-incubation for 12 h. First, 50 μL of supernatant was transferred from all wells to a fresh 96-well plate, and then 50 μL of CytoTox 96 reagent was added. The plate was covered with foil to protect it from light and incubated at room temperature for 30 min. Finally, 50 μL of stop solution was added to each well. Any large bubble was popped using a syringe needle, and the absorbance was recorded at 490 or 492 nm within 1 h after adding Stop Solution. Results were calculated as: % Cytotoxicity = (Experimental–Effector Spontaneous–Target Spontaneous)/(Target Maximum – Target Spontaneous) × 100.
The data in this study were expressed as mean ± SD. Graph-Pad Prism 7 statistical software (GraphPad Software Inc., San Diego, CA, USA) was used for single-factor t-test analysis. p ≤ 0.05 was considered statistically significant.
Activated platelets promote the proliferation of T lymphocytes
To determine whether platelets affect the proliferation of in vitro cultured T lymphocytes, PBMCs were prepared and treated with inactive platelets, activated platelets or remained platelets-free. As depicted in Figs. 1A and 1B, the quantity of PBMCs in the non-activated platelet group differed marginally compared with the group without platelet treatment, while the amount of PBMCs significantly increased in the activated platelet group. Moreover, the cell growth curves of the group treated with activated platelets in dose-dependent response showed that the effect of promoting proliferation of PBMCs was more obvious with an increase in activated platelets, suggesting that the proportion of activated platelets may play an important role in regulating immunity (Figs. 1A and 1B).
Figure 1: Activated platelets promote T lymphocyte proliferation. (A) Representative pictures of peripheral blood mononuclear cells (PBMCs) in the presence of platelets-free, inactive platelets or activated platelets for 10 days (40×), as indicated (1. Platelets-free, 2. 1/3 non-activated platelets, 3. 2/3 non-activated platelets, 4. 1/3 activated platelets, 5. 2/3 activated platelets). (B) Representative growth curves of PBMCs in the presence of platelets-free, inactive platelets or activated platelets. Cells were counted in triplicate wells at each time point, n = 3. *p < 0.05. (C) Representative pictures of PBMCs treated with platelets precipitate or supernatant released from activated platelets for 10 days (40×), as indicated (1. Platelets-free, 2. Platelets supernatant, 3. Platelets precipitate). (D) Cell quantity of PBMCs treated with platelets precipitate or supernatant released from activated platelets for 10 days, as indicated (1. Platelets-free, 2. Platelets supernatant, 3. Platelets precipitate). Cells were counted in triplicate wells, n = 3. ***p < 0.001.
To further elucidate some bioactive substances expressed or released from activated platelets that play a key role in promoting T lymphocyte proliferation, we prepared PBMC treated with activated platelet precipitation (platelet lysate), activated platelet release supernatant (platelets supernatant) or treated without platelet treatment (platelets-free). After 10 days of induction in vitro, PBMCs co-cultured with either activated platelet precipitates or activated platelet supernatants exhibited considerably higher proliferation, as demonstrated by larger cell aggregates visible under a microscope, compared to those not treated with platelet treatment. Among them, cells co-cultured with platelet precipitate showed a more prominent effect (Fig. 1C). Our results support that some substances generated in platelet precipitate or supernatant after platelet activation are favorable to PBMC proliferation. The promoting effect of either platelet precipitate or supernatant on T lymphocyte proliferation was further confirmed by counting the cell number under a microscope. As expected, the number of PBMCs treated with either precipitation or supernatant of activated platelet had significantly increased as compared with that in the untreated group (Fig. 1D). Collectively, these findings support that platelets have a pro-proliferative effect on PBMCs in vitro, since some bioactive factors may be released by activated platelets that substantially boost PBMCs proliferation. To further investigate the effect of platelets on PBMCs cultured in vitro, fully activated platelets were used in subsequent experiments without distinguishing between supernatant and precipitate.
Activated platelets affect the differentiation of T lymphocyte subsets
To investigate the effect of platelets on T-lymphocyte subsets, we measured the proportion of CD3+CD4+, CD3+CD8+, and CD3+CD4+CD25+ T cells in three groups treated with platelets-free, inactivated and activated platelets. As depicted in Figs. 2A and 2B, the proportion of CD3+ T cells cultured in a T-lymphocyte culture system for 10 days reached more than 90%, indicating that T-lymphocytes were the main subgroup in PBMCs after induction in vitro. Moreover, the expression of CD3+CD8+ on the surface of T lymphocytes significantly increased in both the activated platelets-treated group and the inactivated platelets-treated group. However, the proportion of CD3+CD4+ T and CD3+CD4+CD25+ T lymphocytes changed slightly across the three groups (Figs. 2C and 2D), indicating that platelets are essential for CD3+CD8+ T lymphocytes (CTL cells) differentiation, whereas there was no effect on CD3+CD4+ T lymphocytes (Th cells) and suppressor regulatory T-lymphocyte (Treg cells; CD3+CD4+CD25+ T), which may promote the killing activity of adoptive immunity.
Figure 2: Activated platelets affect the differentiation of T lymphocyte subsets. (A) Representative pictures of peripheral blood mononuclear cells (PBMCs) stained with immune cell subset markers: CD3, CD4, CD8 for T lymphocyte, and positive percent was detected by flow-cytometric analysis; (B) The proportion of CD3+, CD3+CD4+, and CD3+CD8+ lymphocytes in PBMCs treated with platelets-free, inactive platelets and activated platelets, respectively (1. Platelets-free, 2. Non-activated platelets, 3. Activated platelets). *p < 0.05, **p < 0.01. The column represents the results of three experiments, n = 3; bar means ± SD; (C) Representative pictures of PBMCs stained with immune cell subset markers: CD3, CD4, CD25 for T lymphocyte, and positive percent was detected by flow-cytometric analysis; (D) The proportion of CD3+, CD3+CD4+ and CD3+CD4+CD25+ lymphocytes in PBMCs treated with platelets-free, inactive platelets and activated platelets, respectively (1. Platelets-free, 2. Non-activated platelets, 3. Activated platelets). The column represents the results of three experiments, n = 3, bar means ± SD.
Activated platelets affect the differentiation of natural killer lymphocytes
To further determine whether activated platelets contribute to the differentiation of different T lymphocyte subsets, we detected the proportion of CD3+CD16+CD56+ T cells (NKT cells) in the three groups treated with platelets-free, inactive platelets and activated platelets, respectively. The results showed that activated platelets or non-activated platelets could downregulate the proportion of NKT lymphocytes (Figs. 3A and 3B). Surprisingly, activated platelets could significantly enhance the proportion of CD3-CD16+CD56+ lymphocytes (Figs. 3A and 3B), indicating an increased proportion of NK cells after activated platelets treatment. So, we questioned whether the killing effect of PBMCs treated with activated platelets can be enhanced in vitro.
Figure 3: Activated platelets affect the differentiation of natural killer (NK) T lymphocytes (including NKT). (A) Representative pictures of PBMC stained with immune cell subset markers: CD3, CD16, CD56, and positive percent were detected by flow-cytometry. (B) The proportion of CD3+, CD3+CD16+CD56+, and CD3-CD16+CD56+ lymphocytes in PBMCs treated with platelets-free, inactive platelets, and activated platelets, respectively (1. Platelets-free, 2. Non-activated platelets, 3. Activated platelets). *p < 0.05, **p < 0.01. The column represents the results of three experiments, n = 3; bar means ± SD.
Activated platelets enhance the cytotoxicity of lymphocytes cultured in vitro
To gain insight into whether platelet activation affects the cytotoxicity of lymphocytes cultured in vitro, we investigated the cell death of human non-small lung cancer cells A549, human pancreatic cancer cells PANC-1, and human chronic myelocytic leukemia cells K562 treated with platelets-free, inactive platelets or activated PBMCs. The results in Figs. 4A and 4B show that activated platelets significantly increased the killing rate of PBMCs. Taking an equal volume of lymphocytes cultured under different conditions (the activated platelet group contained more cells than the other groups), we found that the activated platelet group promoted the cell death of lymphocytes against the three tumor cells (Fig. 4A). Surprisingly, the tumor-killing rate of the activated platelet group was slightly higher than the other two groups when the same number of T lymphocytes were removed from the three groups (Fig. 4B). Notably, we found a remarkable increase in the expression of the cytotoxic marker granzyme B in immune cells in the activated platelet-treated group (Figs. 4C and 4D). Thus, the mechanism of platelet activation affecting the killing ability of peripheral blood lymphocytes may be related to increasing the proportion of NK cells releasing granzyme B. Our results also show that activated platelets can enhance the killing activity of lymphocytes cultured in vitro by promoting cell proliferation and cell differentiation.
Figure 4: Activated platelets promote tumor toxicity of lymphocytes cultured in vitro. (A) Cell death of tumor cells (A549, PANC-1, and K562) co-incubated with an equal volume of PBMC treated with platelets-free, inactive platelets or activated platelets for 10 days and cell death was detected by lactate dehydrogenase (LDH) release assay (1. Platelets-free, 2. Non-activated platelets, 3. Activated platelets). **p < 0.01, ***p < 0.001, ****p < 0.0001. The column represents the results of three experiments, n = 3; bar means ± SD; (B) Cell death of tumor cells (A549, PANC-1, and K562) co-incubated with the same quantity of PBMC treated with platelets-free, inactive platelets or activated platelets for 10 days and cell death was detected by LDH release assay (1. Platelets-free, 2. Non-activated platelets, 3. Activated platelets). *p < 0.05, **p < 0.01, ***p < 0.001. The column represents the results of three experiments, n = 3; bar means ± SD; (C) Representative pictures of granzyme B expression in the three treated groups by flow-cytometric analysis (orange: platelets-free, blue: non-activated platelets, red: activated platelets); (D) The proportion of granzyme B expression in three groups treated with platelets-free, inactive platelets and activated platelets, respectively (1. Platelets-free, 2. Non-activated platelets, 3. Activated platelets). ***p < 0.001. The column represents the results of three experiments, n = 3, bar means ± SD.
Taken together, our results demonstrated that activated platelets may provide a new strategy for optimizing the culture system for adoptive immunotherapy and improving the efficacy of adoptive immunotherapy, especially NK lymphocytes adoptive immunotherapy.
Adoptive immunity, a kind of tumor biotherapy, works by introducing activated and proliferated lymphocytes into tumor patients in vitro, reducing tumor size and preventing tumor metastasis (Wang and Cao, 2020). Transportable cells include cytokine-activated killer (NK) cells, tumor-infiltrating lymphocyte, and cytotoxic T cells (CTLs). Adoptive immunity requires a large number of lymphocytes to be effective. Therefore, obtaining lymphocytes with more proliferation and stronger killing activity during in vitro culture is an indispensable means to improve adoptive immunity.
Platelets are an important component of the immune system that stores and releases many biologically active substances, including cytokines, chemokines, and surface receptors (Mandel et al., 2022). Platelets not only interact with various cells in the immune system and affect the migration, adhesion, activation, differentiation, killing, and other processes of immune cells, but also directly remove pathogenic microorganisms (Mandel et al., 2022). The imbalance of platelets and T lymphocytes is an important feature of some immune diseases. For example, in idiopathic thrombocytopenic purpura (ITP), characterized by thrombocytopenia and cutaneous mucosal bleeding, there is an imbalance in immune function, including an imbalance in the ratio of lymphocyte and changes in various cytokines and chemokines (Onisâi et al., 2019). However, there is no literature on the effect of platelets on lymphocytes in vitro.
In this study, we identified, for the first time, that platelet activation plays an important role in promoting lymphocyte proliferation and activation in vitro. First, to optimize the culture system of PBMCs, we explored the effect of some parameters of platelets on PBMC proliferation, including different states of platelets, activated or inactivated, and the number of activated platelets. By analyzing the growth curve of PBMCs at different amounts of activated platelets, we found that lymphocyte proliferation requires Ca2+ to activate platelets (Davlouros et al., 2016), and is dose-dependent with activated platelets. We also noticed that the results of lymphocyte status and number showed that both platelet precipitation and Ca2+-activated supernatant significantly promoted the proliferation of lymphocytes. Activated platelets are well known to not only release a variety of substances such as TGF-β, PDGF, PF4, and serotonin, but also express P-selectin, CD40L, and TLR on the cell membrane (Anka Idrissi et al., 2021). The significant difference between the effects of activated and inactivated platelets suggests that platelet activation affects the proliferation of lymphocytes through releasing certain factors or expressing some membrane molecules, which lays the foundation for further study on the mechanism of platelet action in immunomodulation.
Culturing lymphocytes in vitro, in addition to the importance of cell quantity, is more important to improve the killing activity, which involves the role of platelets in lymphocyte differentiation. In vivo, CD4+ T lymphocytes can be classified into two different subsets: Th cells and Treg cells. Th cells, also known as helper T cells, can secrete a variety of cytokines that aid in immune response. Treg cells, or CD4+CD25+ suppressor T cells, can prevent T lymphocytes from being activated and proliferating. CD8+ T lymphocytes, also known as Tc cells, can directly kill virus-infected or tumor-bearing cells (Kumar et al., 2018). To explore the differentiation of Th, Treg, and killer T-lymphocytes (Tc cells) within the T-lymphocyte culture system in vitro, especially the effect of platelets on T lymphocyte subsets, we detected the proportion of CD4+CD25− (Th), CD4+CD25+ (Treg) and CD8+ (Tc) cells in CD3+ cells (≥90%). The amount of Tc cells in the culture system containing activated platelets or inactivated platelets increased significantly when compared to the group in the presence of platelet-free, whereas the amount of Th cells and Treg cells remained unchanged (Fig. 2). Because of in vitro CD8+ T lymphocytes limited by major histocompatibility complex (MHC), they cannot directly kill tumor cells. NK cells (including NKT) are NK lymphocytes with characteristics not limited by MHC, which can directly kill target cells through a variety of mechanisms (Pyaram and Yadav, 2018). By detecting the proportion of NK cells with more broad-spectrum killing activity, we surprisingly found that activated platelets can significantly enhance the proportion of CD3-CD16+CD56+ lymphocytes but downregulate the proportion of NKT lymphocytes (Figs. 3A and 3B). Obviously, whether it is CD8+ T lymphocytes or NK lymphocytes, the increase in their number is undoubtedly beneficial for adoptive immunotherapy (Buck et al., 2015).
We further explored the effect of activated platelets on the cytotoxicity of NK cells in a T lymphocyte culture system; we used tumor cells A549, PANC-1, and K562 as target cells to detect the change in the killing rate of PBMCs under different conditions. Unexpectedly, we found that PBMCs containing activated platelets exhibited greater cytotoxicity to the three cells compared than platelets-free or non-activated platelets treated group, even though all three different treatment groups had the same number of PBMCs (Fig. 4). We clearly concluded that activated platelets promote the cytotoxicity of PBMCs in vitro as well as the natural killer capabilities of the culture system, which is favorable for adoptive immunotherapy. In the culture system, lymphocytes can exert cytotoxic effects in a variety of ways, including the release of perforin, granzyme, interferon-γ and the expression of Fas ligand, all of which have anti-tumor properties (Bae et al., 2019). Therefore, we discovered that in the culture system including activated platelets, the expression of granzyme B, a cytotoxic substance, was significantly elevated (Figs. 4C and 4D). Both CD8+ T lymphocytes and NK cells can kill target cells in vivo by releasing granzyme B (Turner et al., 2019). As a result, the rise in granzyme B suggests that activated platelets can greatly enhance the total lymphocyte-killing ability after reinfusion, which is of great significance for tumor patients.
Therefore, our research innovatively found that platelets can promote lymphocyte proliferation, optimize lymphocyte subpopulations, and, more importantly, enhance anti-tumor activity in vitro, which is advantageous for cell immunotherapy, especially NK cell immunotherapy. However, the general thought about platelets in the field of cancer is that antiplatelet therapy can indeed improve the prognosis of some forms of cancers because platelets can promote tumorigenesis and metastasis, especially as they are a major source for TGF-β which is immunosuppressive in nature (Balakrishna et al., 2021; Li et al., 2023; Catani et al., 2020). However, it is undeniable that platelets can release many factors, which have completely different effects (Catani et al., 2020), that may have important effects on lymphocytes cultured in vitro. This study is simply a preliminary exploration of the immunomodulation of platelets in vitro. The mechanism of platelets in immunomodulation and the mechanism of platelets in vivo require further investigation, but our research has laid a foundation for further elucidating the mechanism of platelets in vivo and in vitro, even for elucidating the complicated pathophysiological mechanism of immunological diseases.
In summary, our study pleasantly revealed that activated platelets can promote the proliferation of PBMCs, increase the proportion of CD8+ T cells and NK cells, and even promote the killing activity of PBMCs in vitro. Undoubtedly, our research will provide a new strategy for adoptive immunotherapy, especially for adoptive immunity of NK lymphocytes.
Acknowledgement: We acknowledge Dr. Hui Wang from Pub-Lab Platform, West China School of Basic Medical Sciences and Forensic Medicine, Sichuan University, for assistance with the flow cytometry (BD, FACS Melody).
Funding Statement: The authors received no specific funding for this study.
Author Contributions: The authors confirm contribution to the paper as follows: study conception and design: XW, PPW, DPW; data collection: PPW, CLC, XHL; analysis and interpretation of results: PPW, CLC, YZ; draft manuscript preparation: PPW, CLC, YZ, JMH. All authors reviewed the results and approved the final version of the manuscript.
Availability of Data and Materials: All data generated or analyzed during this study are included in this published article.
Ethics Approval: The authors are accountable for all aspects of the work in ensuring that questions related to the accuracy or integrity of any part of the work are appropriately investigated and resolved. The study was conducted in accordance with the Declaration of Helsinki (as revised in 2013). The study was approved by the Medical Ethics Committee of Sichuan University (No. K2021006), and informed consent was taken from all the volunteers.
Conflicts of Interest: The authors declare that they have no conflicts of interest to report regarding the present study.
References
Anka Idrissi D, Senhaji N, Aouiss A, Khalki L, Tijani Y et al. (2021). IL-1 and CD40/CD40L platelet complex: Elements of induction of Crohn’s disease and new therapeutic targets. Archives Pharmacal Research 44: 117–132. https://doi.org/10.1007/s12272-020-01296-1 [Google Scholar] [PubMed] [CrossRef]
Aslan JE (2021). Platelet proteomes, pathways, and phenotypes as informants of vascular wellness and disease. Arteriosclerosis, Thrombosis, and Vascular Biology 41: 999–1011. https://doi.org/10.1161/ATVBAHA.120.314647 [Google Scholar] [PubMed] [CrossRef]
Bae EA, Seo H, Kim IK, Jeon I, Kang CY (2019). Roles of NKT cells in cancer immunotherapy. Archives of Pharmacal Research 42: 543–548. https://doi.org/10.1007/s12272-019-01139-8 [Google Scholar] [PubMed] [CrossRef]
Balakrishna P, George S, Hatoum H, Mukherjee S (2021). Serotonin pathway in cancer. International Journal of Molecular Sciences 22: 1268. https://doi.org/10.3390/ijms22031268 [Google Scholar] [PubMed] [CrossRef]
Buck MD, O’Sullivan D, Pearce EL (2015). T cell metabolism drives immunity. The Journal of Experimental Medicine 212: 1345–1360. https://doi.org/10.1084/jem.20151159 [Google Scholar] [PubMed] [CrossRef]
Catani MV, Savini I, Tullio V, Gasperi V (2020). The “janus face” of platelets in cancer. International Journal of Molecular Sciences 21: 788. https://doi.org/10.3390/ijms21030788 [Google Scholar] [PubMed] [CrossRef]
Claushuis TAM, van der Veen AIP, Horn J, Schultz MJ, Houtkooper RH, Van’t Veer C, van Der Poll T (2019). Platelet toll-like receptor expression and activation induced by lipopolysaccharide and sepsis. Platelets 30: 296–304. https://doi.org/10.1080/09537104.2018.1445841 [Google Scholar] [PubMed] [CrossRef]
Davlouros P, Xanthopoulou I, Mparampoutis N, Giannopoulos G, Deftereos S, Alexopoulos D (2016). Role of calcium in platelet activation: Novel insights and pharmacological implications. Medicinal Chemistry 12: 131–138. https://doi.org/10.2174/157340641202160208195923 [Google Scholar] [PubMed] [CrossRef]
El Filaly H, Mabrouk M, Atifi F, Guessous F, Akarid K, Merhi Y, Zaid Y (2023). Dissecting platelet’s role in viral infection: A double-edged effector of the immune system. International Journal of Molecular Sciences 24: 2009. https://doi.org/10.3390/ijms24032009 [Google Scholar] [PubMed] [CrossRef]
Karmakar S, Lal G (2021). Role of serotonin receptor signaling in cancer cells and anti-tumor immunity. Theranostics 11: 5296–5312. https://doi.org/10.7150/thno.55986 [Google Scholar] [PubMed] [CrossRef]
Kumar BV, Connors TJ, Farber DL (2018). Human T cell development, localization, and function throughout life. Immunity 48: 202–213. https://doi.org/10.1016/j.immuni.2018.01.007 [Google Scholar] [PubMed] [CrossRef]
Li Y, Wang H, Zhao Z, Yang Y, Meng Z, Qin L (2023). Effects of the interactions between platelets with other cells in tumor growth and progression. Frontiers in Immunology 14: 1165989. https://doi.org/10.3389/fimmu.2023.1165989 [Google Scholar] [PubMed] [CrossRef]
Mandel J, Casari M, Stepanyan M, Martyanov A, Deppermann C (2022). Beyond hemostasis: Platelet innate immune interactions and thromboinflammation. International Journal of Molecular Sciences 23: 3868. https://doi.org/10.3390/ijms23073868 [Google Scholar] [PubMed] [CrossRef]
Manne BK, Xiang SC, Rondina MT (2017). Platelet secretion in inflammatory and infectious diseases. Platelets 28: 155–164. https://doi.org/10.1080/09537104.2016.1240766 [Google Scholar] [PubMed] [CrossRef]
Maouia A, Rebetz J, Kapur R, Semple JW (2020). The immune nature of platelets revisited. Transfusion Medicine Reviews 34: 209–220. https://doi.org/10.1016/j.tmrv.2020.09.005 [Google Scholar] [PubMed] [CrossRef]
Nurden AT (2018). The biology of the platelet with special reference to inflammation, wound healing and immunity. Frontiers in Bioscience 23: 726–751. https://doi.org/10.2741/4613 [Google Scholar] [PubMed] [CrossRef]
Onisâi M, Vlădăreanu AM, Spînu A, Găman M, Bumbea H (2019). Idiopathic thrombocytopenic purpura (ITP)—new era for an old disease. Romanian Journal of Internal Medicine 57: 273–283. https://doi.org/10.2478/rjim-2019-0014 [Google Scholar] [PubMed] [CrossRef]
Pyaram K, Yadav VN (2018). Advances in NKT cell immunotherapy for glioblastoma. Journal of Cancer Science & Therapy 10: 533. https://doi.org/10.4172/1948-5956.1000533 [Google Scholar] [PubMed] [CrossRef]
Rachidi S, Metelli A, Riesenberg B, Wu BX, Nelson MH et al. (2017). Platelets subvert T cell immunity against cancer via GARP-TGFβ axis. Science Immunology 2: eaai7911. https://doi.org/10.1126/sciimmunol.aai7911 [Google Scholar] [PubMed] [CrossRef]
Sang Y, Roest M, de Laat B, de Groot PG, Huskens D (2021). Interplay between platelets and coagulation. Blood Reviews 46: 100733. https://doi.org/10.1016/j.blre.2020.100733 [Google Scholar] [PubMed] [CrossRef]
Turner CT, Lim D, Granville DJ (2019). Granzyme B in skin inflammation and disease. Matrix Biology 75–76: 126–140. https://doi.org/10.1016/j.matbio.2017.12.005 [Google Scholar] [PubMed] [CrossRef]
van der Meijden P E J, Heemskerk J W M (2019). Platelet biology and functions: New concepts and clinical perspectives. Nature Reviews Cardiology 16: 166–179. https://doi.org/10.1038/s41569-018-0110-0 [Google Scholar] [PubMed] [CrossRef]
Wang Z, Cao YJ (2020). Adoptive cell therapy targeting neoantigens: A frontier for cancer research. Frontiers in Immunology 11: 176. https://doi.org/10.3389/fimmu.2020.00176 [Google Scholar] [PubMed] [CrossRef]
Wang S, Li Z, Xu R (2018). Human cancer and platelet interaction, a potential therapeutic target. International Journal of Molecular Sciences 19: 1246. https://doi.org/10.3390/ijms19041246 [Google Scholar] [PubMed] [CrossRef]
Yamashita A, Asada Y (2023). Underlying mechanisms of thrombus formation/growth in atherothrombosis and deep vein thrombosis. Pathology International 73: 65–80. https://doi.org/10.1111/pin.13305 [Google Scholar] [PubMed] [CrossRef]
Yan C, Wu H, Fang X, He J, Zhu F (2023). Platelet, a key regulator of innate and adaptive immunity. Frontiers in Medicine 10: 1074878. https://doi.org/10.3389/fmed.2023.1074878 [Google Scholar] [PubMed] [CrossRef]
Yeung J, Li W, Holinstat M (2018). Platelet signaling and disease: Targeted therapy for thrombosis and other related diseases. Pharmacological Review 70: 526–548. https://doi.org/10.1124/pr.117.014530 [Google Scholar] [PubMed] [CrossRef]
Cite This Article
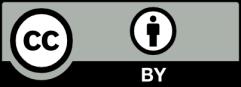
This work is licensed under a Creative Commons Attribution 4.0 International License , which permits unrestricted use, distribution, and reproduction in any medium, provided the original work is properly cited.