Open Access
ARTICLE
NR4A1 enhances glycolysis in hypoxia-exposed pulmonary artery smooth muscle cells by upregulating HIF-1α expression
1 Cardiovascular Department, The Third Xiangya Hospital of Central South University, Changsha, 410013, China
2 Department of Cardiovascular Medicine, The Second Xiangya Hospital, Central South University, Changsha, 410011, China
* Corresponding Authors: CHENYANG CHEN. Email: ; JIANG LI. Email:
(This article belongs to the Special Issue: Cellular Signal Transduction in Biological Activities)
BIOCELL 2023, 47(11), 2423-2433. https://doi.org/10.32604/biocell.2023.044459
Received 31 July 2023; Accepted 27 September 2023; Issue published 27 November 2023
Abstract
Background: Pulmonary arterial hypertension (PAH) is a chronic and progressive disease that is strongly associated with dysregulation of glucose metabolism. Alterations in nuclear receptor subfamily 4 group A member 1 (NR4A1) activity alter the outcome of PAH. This study aimed to investigate the effects of NR4A1 on glycolysis in PAH and its underlying mechanisms. Methods: This study included twenty healthy volunteers and twenty-three PAH patients, and plasma samples were collected from the participants. To mimic the conditions of PAH in vitro, a hypoxia-induced model of pulmonary artery smooth muscle cell (PASMC) model was established. The proliferation of PASMCs was assessed using CCK8 assays. Results: Levels of NR4A1, hypoxia-inducible factor-1α (HIF-1α), and various glycolysis-related enzymes were measured. In addition, extracellular glucose and lactate production were assessed. The interaction between NR4A1 and HIF-1α was evaluated by co-immunoprecipitation assays. Levels of NR4A1 and HIF-1α was increased in PAH patients, and exposure to hypoxia resulted in increased levels of NR4A1 and HIF-1α in PASMCs. NR4A1 interacted with HIF-1α. NR4A1 overexpression enhanced hypoxia-induced expression of HIF-1α, GLUT1, PKM2, HK2, and CD36, decreased glucose levels, increased lactate levels and promoted hypoxic PASMC viability. Conversely, silencing NR4A1 decreased hypoxia-induced expression of HIF-1α, GLUT1, PKM2, HK2, and CD36, promoted glucose production, reduced lactate levels and inhibited hypoxic PASMC viability. Furthermore, overexpression of HIF-1α reversed the regulation of glycolysis caused by NR4A1 knockdown. Conclusion: NR4A1 enhances glycolysis in hypoxia-induced PASMCs by upregulating HIF-1α. Our findings indicate that the management of NR4A1 activity may be a promising strategy for PAH therapy.Graphical Abstract
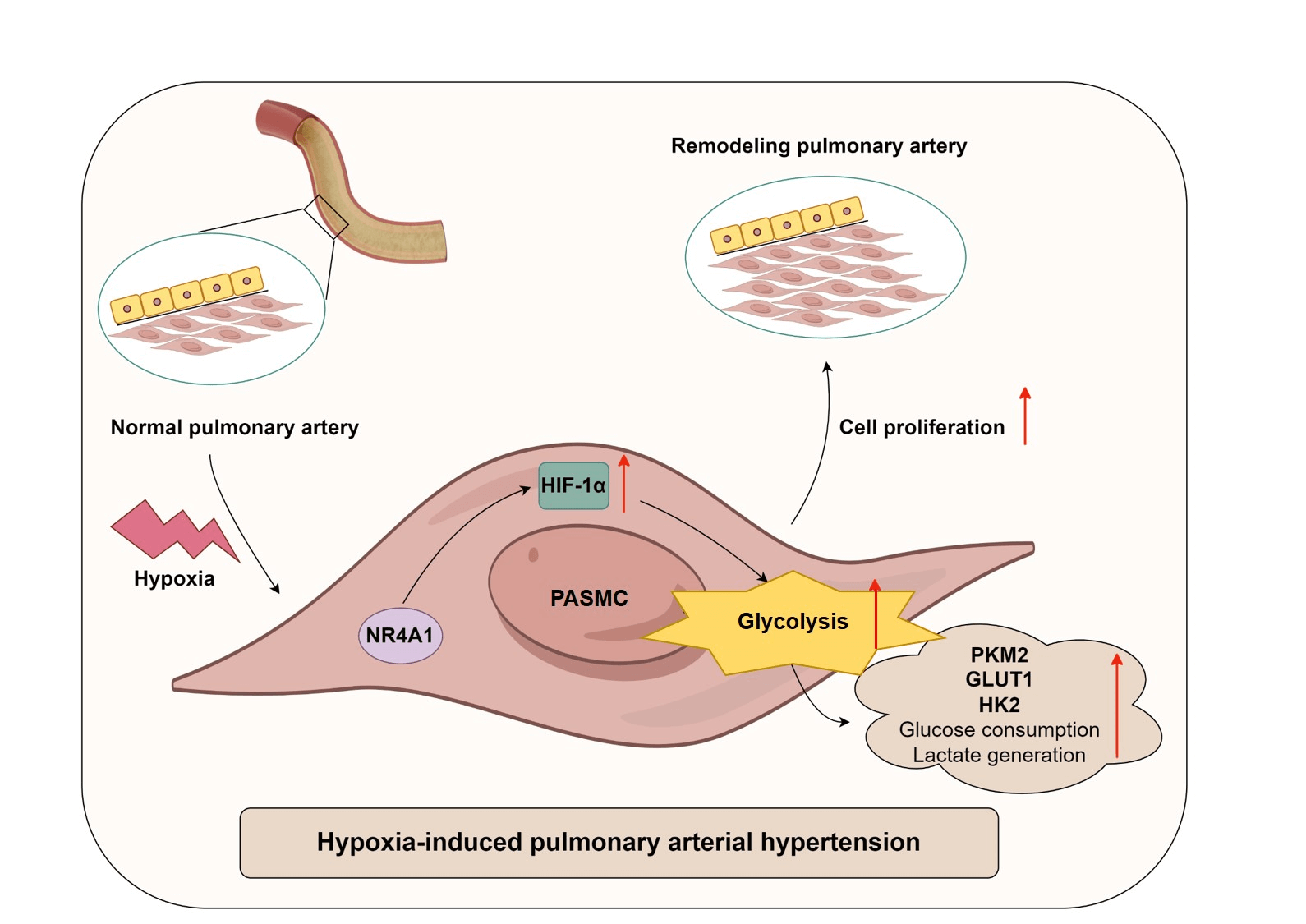
Keywords
Pulmonary arterial hypertension (PAH) is a severe cardiopulmonary disease characterized by the progressive pulmonary arterial pan-vasculopathy, resulting in elevated pulmonary artery pressure, enhanced pulmonary vascular resistance, and subsequent right ventricular failure and functional decline, ultimately leading to death (Beshay et al., 2020; Shah et al., 2022). Epidemiologic studies have indicated a minimum incidence of 15 cases per million, but patient survival is not optimistic, highlighting the need to improve patient survival rates (Lau et al., 2017; He et al., 2022). Therefore, understanding the pathogenesis of PAH is critical to improving patient outcomes.
Loss of the pulmonary vascular bed and obstructive remodeling are important contributors to the pathophysiology of PAH (Humbert et al., 2019). The accumulation of various vascular cells, particularly pulmonary arterial smooth muscle cells (PASMCs), within the pulmonary arterial wall is considered a critical indicator of pulmonary vascular remodeling (Kovacs et al., 2019; Li et al., 2021). Furthermore, altered metabolic pathways are frequently observed in both PAH patients and animal models (Xu et al., 2021). Glycolysis, the breakdown of glucose to pyruvate, is a key metabolic process. Under aerobic conditions, pyruvate enters the mitochondria and is converted to acetyl-coenzyme A; under anaerobic conditions, pyruvate is converted to lactate (Chandel, 2021). In the context of PAH, glycolysis is upregulated in pulmonary artery endothelial cells (PAECs) and PASMCs (Li et al., 2019; Ji et al., 2022). Inhibition of glycolysis has been shown to reduce the excessive proliferation of PASMCs (Li et al., 2021). Hypoxia-inducible factor-1α (HIF-1α) has been found to play an important role in glycolysis (Seagroves et al., 2001). In the hypoxic conditions, HIF-1α induces the upregulation of glucose transport proteins and glycolytic enzymes (Tirpe et al., 2019; Kierans and Taylor, 2021). Exposure to hypoxia or aberrant activation of HIF-1α leads to hyperproliferation of PASMCs and pulmonary vascular remodeling, which facilitates the development of PAH (Veith et al., 2016; Luo et al., 2019). Conversely, HIF-1α deficiency attenuates pulmonary vascular remodeling in hypoxic mice (Veith et al., 2016; Luo et al., 2019). Therefore, investigation of factors associated with HIF-1α-induced glycolysis is critical for understanding the pathogenesis of PAH.
Nuclear receptor subfamily 4 group A member 1 (NR4A1) belongs to the NR4A orphan nuclear receptor family. Its expression is rapidly and transiently induced by extracellular signals, making it an early gene and act as a transcription factor (Odagiu et al., 2020). NR4A1 is significantly involved in the maintenance of cellular homeostasis and pathophysiology (Wu and Chen, 2018; Herring et al., 2019). A previous report has demonstrated that NR4A1 expression in rat PASMCs is promoted by pathological stimuli associated with PAH, such as hypoxia and 5-hydroxytryptamine (5-HT) exposure. Overexpression of NR4A1 inhibits 5-HT-induced PASMC proliferation (Liu et al., 2014). Mechanistically, Liu et al. (2014) demonstrated that NR4A1 interacts with STAT3 to inhibit its phosphorylation and downregulate the transcription of downstream targets (Liu et al., 2014). NR4A1 expression was found to be induced in the lungs of PAH patients and hypoxic mice, but reduced in PASMCs from patients (Nie et al., 2016). Nie et al. (2016) also found that NR4A1 knockdown increased PASMC proliferation and migration, whereas NR4A1 overexpression inhibited these processes (Nie et al., 2016). Additionally, Kurakula et al. (2019) reported decreased expression of NR4A1 in pulmonary microvascular endothelial cells (MVECs) and the lung tissue from PAH patients, and activation of NR4A1 reduced cell proliferation and inflammation while enhancing BMP signaling to restore MVEC function (Kurakula et al., 2019). However, no information is currently available on the effects of NR4A1 on HIF-1α-induced glycolysis in PAH.
Therefore, in this study, the expression patterns of NR4A1 and HIF-1α in healthy subjects and PAH patients were investigated. In addition, we established a hypoxia-induced PASMC model to investigate the involvement of the NR4A1/HIF-1α axis in glycolysis in PAH. Our results showed that hypoxia stimulation promoted HIF-1α-mediated glycolysis in PASMCs, and this process was closely associated with the upregulation of NR4A1.
The study included a total of 20 healthy volunteers and 23 patients diagnosed with PAH. Among the PAH patients, 3 had idiopathic PAH (IPAH) and 20 had PAH associated with congenital heart disease. Patients with PAH were diagnosed according to the guidelines of the 6th World Symposium on Pulmonary Hypertension (Galie et al., 2019; Montani et al., 2021; Shu et al., 2023). PAH subjects included patients with idiopathic and congenital heart disease-related PAH. Patients with other known etiologies of PAH were excluded, therefore not including patients with PAH associated with clinically diagnosed autoimmune diseases. Based on the World Health Organization Functional Class (WHO-FC) classification, 15 patients were classified as class II, all of them female, with a mean pulmonary artery pressure of 52.13 ± 13.56 mmHg. Eight patients were classified as class III, equally divided males and females, with a mean pulmonary artery pressure of 44.13 ± 16.47 mmHg (Table 1). This study was conducted with the approval of the IRB of Third Xiangya Hospital, Central South University (2020-S109), and written informed consent was obtained from each participant.
Primary human PASMCs (ScienCell Research Laboratories, Carlsbad, CA, USA) were cultured in smooth muscle cell-specific medium (AW-MC016, Abiowell, Changsha, China). The 3–5th generation of PASMCs were used for further experiments (Xu et al., 2022). Normoxic PASMCs were cultured in an incubator at 37°C with a gas mixture of 21% O2, 5% CO2, and 74% N2. For hypoxic treatment, PASMCs were starved for 12 h and then cultured for an additional 24 h at 37°C with a gas mixture of 3% O2, 5% CO2, and 92% N2 (Xu et al., 2022). Cell morphology was observed by a light microscopy.
To study the role of NR4A1 in HIF-1α expression and glycolysis, si-NC and si-NR4A1 (HG-Hi0080114, HonorGene, Changsha, China) were transfected into hypoxia-treated PASMCs. Lipofectamine 2000 kit (11668019, Invitrogen, Carlsbad, CA, USA) was used for transfection. In addition, to study the role of NR4A1/HIF-1α axis in glycolysis, si-NR4A1 and oe-HIF-1α (HG-HO0090012, HonorGene) were co-transfected into hypoxia-treated PASMCs. To construct the overexpression vectors, the sequences of NR4A1 or HIF-1α were obtained from NCBI, and primers were designed accordingly to ligate them into the pcDNA3.1(+) vector. The sequence of si-NR4A1 used was 5′-GCCCTGTATCCAAGCTCAATATGGA-3′, and the sequence of si-NC used was 5′-TTCTCCGAACGTGTCACGTTACGG-3′.
Based on the presence of the disease, individuals were categorized into Normal and PAH groups. PAH patients were further divided into WHO-FC (II) and WHO-FC (III) groups based on their functional classification.
According to different treatment conditions, PASMCs were divided into the Normoxia group (treated under normal oxygen conditions) and the Hypoxia group (treated under hypoxic conditions).
According to different transfection reagents, hypoxic PASMCs treated with si-NC were defined as the si-NC group, and hypoxic PASMCs treated with si-NR4A1 were defined as the si-NR4A1 group. Hypoxic PASMCs treated with oe-NC were defined as the oe-NC group, and hypoxic PASMCs treated with oe-NR4A1 were defined as the oe-NR4A1 group.
Hypoxic PASMCs treated with si-NC were defined as the si-NC group, hypoxic PASMCs treated with si-NR4A1 were defined as the si-NR4A1 group, hypoxic PASMCs treated with si-NR4A1 and oe-NC were defined as the si-NR4A1+oe-NC group, and hypoxic PASMCs treated with si-NR4A1 and oe-HIF-1α were defined as the si-NR4A1+oe-HIF-1α group.
Cell counting kit-8 (CCK8) assay
CCK8 assays were used to evaluate cell viability. Briefly, after digestion with pancreatic enzymes, cells were seeded in a 96-well plate at a density of 5 × 103 cells/well/100 μL. Then, 10 μL of CCK-8 reagent (AWC0114a, Abiowell) was added to each well, and the cells were incubated for 4 h. Optical density (OD) values at 450 nm were measured using a microplate reader (MB-530, Heales Technology Development Co., Ltd., Shenzhen, China). There were at least three replicates for each group.
Quantitative real-time PCR (qRT-PCR)
Total cellular RNA was extracted from PASMCs using TRIzol (15596026, Thermo Fisher Scientific, Waltham, MA, USA). The RNA was then reverse transcribed to obtain cDNAs using HiFiScript cDNA synthesis kits (CW2569, ConWin Biosciences, Taizhou, China). The UltraSYBR Mixture kits (CW2601, ConWin Biosciences) were used for PCR amplification according to the manufacturer’s instructions. The relative mRNA expression of the targets was normalized to β-actin and determined by the 2−ΔΔCt method. Primers are shown below: NR4A1 (forward, 5′-CCTCGCCTTGGTTGGAGG-3′; reverse, 5′-ACAGGGCATCTCACTCTGGA-3′), HIF-1α (forward, 5′-TGGTATTATTCAGCACGACT-3′; reverse, 5′-GCCAGCAAAGTTAAAGCATC-3′), pyruvate kinase M2 (PKM2, forward, 5′-CGTCATTCATCCGCAAGGCAT-3′; reverse, 5′-CACGAGCCACCATGATCCCA-3′), glucose transporter type 1 (GLUT1, forward, 5′-GGCTTCTCCAACTGGACCTC-3′; reverse, 5′-CCGGAAGCGATCTCATCGAA-3′), hexokinase 2 (HK2, forward, 5′-GTGAATCGGAGAGGTCCCAC-3′; reverse, 5′-GCTAACTTCGGCCACAGGAT-3′), platelet glycoprotein 4 (CD36, forward, 5′-AAAATGTAACCCAGGACG-3′; reverse, 5′-GTGTCGATTATGGCAACT-3′), and β-actin (forward, 5′-ACCCTGAAGTACCCCATCGAG-3′; reverse, 5′-AGCACAGCCTGGATAGCAAC-3′).
Total protein was extracted from PASMCs using RIPA (AWB0136, Abiowell). After protein quantification using a BCA kit (AWB0104, Abiowell), proteins were separated by 10% sodium dodecyl sulfate-polyacrylamide gel electrophoresis, and then electrotransferred to nitrocellulose membranes. The membranes were blocked in 5% skimmed milk for 90 min and then incubated overnight at 4°C with NR4A1 (1:600, 25851-1-AP, Proteintech, Rosemont, IL, USA), HIF-1α (1:1000, ab179483, Abcam, Cambridge, MA, UK), PKM2 (1:4000, 15822-1-AP, Proteintech), GLUT1 (1:4000, 21829-1-AP, Proteintech), HK2 (1:5000, 22029-1-AP, Proteintech), CD36 (1:1000, 18836-1-AP, Proteintech), and β-actin (1:5000, 66009-1-Ig, Proteintech). The membranes were then incubated with anti-mouse (1:5000, SA00001-1, Proteintech) and anti-rabbit (1:6000, SA00001-2, Proteintech) for 90 min. ECL Plus Substrate (AWB0005, Abiowell) was used to visualize the proteins on a gel imaging system (ChemiScope 6100, Clinx, Shanghai, China). Protein expression levels were quantified using Quantity One 4.6 software.
Enzyme-linked immunosorbent assay (ELISA)
Levels of NR4A1 and HIF-1α in patient plasma were measured using the NR4A1 ELISA kit (ml544170V, Shanghai Enzyme-linked Biotechnology Co., Ltd., Shanghai, China) and the HIF-1α ELISA kit (CSB-E12112h, Cusabio, Wuhan, China) according to the kit instructions.
Extracellular glucose levels were quantified by the glucose oxidase method according to the instructions (A154-1-1, Nanjing Jiancheng Bioengineering Institute, Nanjing, China). Meanwhile, lactate levels were determined using the LD assay kit (A019-2-1, Nanjing Jiancheng Bioengineering Institute, Nanjing, China) following the instructions.
Co-inmunoprecipitation (co-IP) assay
The interaction between NR4A1 and HIF-1α was examined using co-IP assay. Briefly, PASMCs were lysed using IP lysis buffer (AWB0144, Abiowell) to extract proteins. Protein supernatants were incubated with normal rabbit IgG (B900610, Proteintech) or NCOA4 (25851-1-AP, Proteintech) antibodies overnight at 4°C with rotation. The IP lysate was then added to Protein A/G agarose beads, mixed, and centrifuged to collect the precipitate. After antibody incubation, the cell lysate was fully incubated with agarose beads for 2 h at 4°C to allow the antibodies to couple to the beads. The agarose beads were washed with IP lysis buffer, and the coupling product was subsequently analyzed by Western blotting analysis.
Statistical analysis was performed using GraphPad Prism 9, and all data are presented as mean ± standard deviation (SD). Unpaired student’s t-test was used to compare the independent samples of a single variable. Kolmogorov-Smirnov test and exploratory descriptive statistics test were used to analyze normal distribution and homogeneity of variance. Parametric tests were used for data analysis. Differences among four groups were determined by one-way analysis of variance (ANOVA) and Tukey’s post-hoc test. Two-way ANOVA and Sidak’s test were used to analyze two variables. Pearson correlation coefficient was used to analyze the correlation between NR4A1 and HIF-1α. p < 0.05 was considered statistically significant. One symbol indicates p < 0.05, two symbols indicates p < 0.01, three symbols indicate p < 0.001, and four symbols indicate p < 0.0001.
NR4A1 and HIF-1α are highly expressed in the plasma of PAH patients
A total of 20 healthy volunteers (Normal) and 23 PAH patients (PAH) were recruited for this study. Of the PAH patients, 3 had IPAH and 20 had PAH associated with congenital heart disease. Baseline information for the patients is shown in Table 1.
To determine whether NR4A1 and HIF-1α are involved in the regulation of PAH processes and the relationship between NR4A1 and HIF-1α, we detected the expression of these two factors in plasma. From the results of the assay, the levels of both NR4A1 and HIF-1α were upregulated compared to healthy subjects (Fig. 1A). The levels of NR4A1 and HIF-1α in plasma did not show significant differences among patients with different cardiac functional classes (Fig. 1B). Furthermore, NR4A1 and HIF-1α were positively correlated using Pearson correlation analysis (Fig. 1C). These clinical data indicate that the NR4A1/HIF-1α axis may play an important role in the progression of PAH.
Figure 1: NR4A1 and HIF-1α expression are elevated and positively correlated in PAH patients. (A) The levels of NR4A1 and HIF-1α in the plasma of healthy subjects (n = 20) and PAH patients (n = 23) were determined by ELISA. (B) The levels of NR4A1 and HIF-1α in the plasma of PAH patients with different cardiac function classes (II and III). (C) The correlation between NR4A1 and HIF-1α was analyzed by Pearson correlation coefficient. ****p < 0.0001 vs. Normal.
Hypoxia induces increased glycolysis in PASMCs
Hypoxia is a known factor that induces PAH and contributes to pulmonary vascular remodeling (Stenmark et al., 2006; Liu et al., 2022). To study the impact of hypoxia on glycolysis in PASMCs, we cultured PASMCs under both normoxic and hypoxic conditions. Our results showed that under normoxic conditions, the cells displayed a contractile phenotype characterized by elongated spindle-like shape and distinct “peak-trough” growth pattern. Conversely, under hypoxic conditions, the cells exhibited a synthetic phenotype, with increased cell volume and pronounced “peak-trough” growth potential (Fig. 2A). In addition, the CCK8 assay showed enhanced proliferation of the cells after 24 h of hypoxia induction (Fig. 2B). In the hypoxia-induced PASMCs, the levels of NR4A1 and HIF-1α were elevated compared to cells in normoxic conditions (Fig. 2C). Furthermore, hypoxia treatment increased the mRNA levels of PKM2, GLUT1, and HK2 in PASMCs (Fig. 2D). It was also observed that hypoxia decreased extracellular glucose levels and increased lactate production (Fig. 2E). These findings collectively demonstrate that hypoxia upregulates the expression of NR4A1 and HIF-1α in PASMCs, thereby promoting the glycolytic process.
Figure 2: Hypoxia increases the expression of NR4A1 and HIF-1α and promotes glycolysis in PASMCs. (A) Cell morphology was observed by a light microscopy, scale bar = 100 μm. (B) CCK8 assays (absorbance at OD450 nm) were used to measure the cell viability of PASMCs at 0 and 24 h. (C) The levels of NR4A1 and HIF-1α were examined in PASMCs. (D) Relative mRNA levels of PKM2, GLUT1, and HK2 were examined. (E) Extracellular glucose and lactate levels were determined. n = 3. ****p < 0.0001 vs. Normoxia.
NR4A1 upregulates HIF-1α expression in PASMCs
We further investigated the relationship between NR4A1 and HIF-1α in hypoxia-induced PASMCs. Co-IP analysis revealed binding between NR4A1 and HIF-1α (Fig. 3A). CCK8 assays showed that transfection with si-NR4A1 led to a decrease in the viability of hypoxia-induced PASMCs compared to the si-NC group (Fig. 3B). Additionally, si-NR4A1 decreased the protein expression of NR4A1 and HIF-1α compared to si-NC (Fig. 3C). These results indicate that NR4A1 targets HIF-1α and increases its expression in hypoxia-induced PASMCs and NR4A1 silencing inhibited cell proliferation.
Figure 3: NR4A1 targets HIF-1α and regulates its expression in PASMCs. (A) Co-IP assay to evaluate the interaction between NR4A1 and HIF-1α. (B) Hypoxia-induced PASMC viability (absorbance at OD450 nm) was assessed by CCK8 at 0 and 24 h. (C) Protein levels of NR4A1 and HIF-1α analyzed in hypoxia-induced PASMCs. n = 3. ****p < 0.0001 vs. si-NC.
HIF-1α-mediated enhancement of glycolysis is associated with NR4A1 upregulation
We next investigated the potential involvement of the NR4A1/HIF-1α axis in glycolysis in hypoxia-induced PASMCs. Compared to the oe-NC, transfection with oe-NR4A1 enhanced the viability of hypoxia-induced PASMCs (Fig. 4A). Transfection with oe-NR4A1 resulted in increased expression of NR4A1 and HIF-1α in hypoxia-induced PASMCs (Fig. 4B). In addition, oe-NR4A1 upregulated the mRNA expression of PKM2, GLUT1, HK2, and CD36 in hypoxia-induced PASMCs (Fig. 4C). Additional analysis revealed that oe-NR4A1 increased the protein levels of PKM2, GLUT1, HK2, and CD36 in hypoxia-induced PASMCs (Fig. 4D). In addition, oe-NR4A1 decreased extracellular glucose levels and increased lactate production when compared with oe-NC (Fig. 4E). These collective findings suggest that NR4A1 overexpression promotes glycolysis in hypoxia-induced PASMCs.
Figure 4: Overexpression of NR4A1 promotes glycolysis in hypoxia-induced PASMCs. (A) The effects of oe-NC and oe-NR4A1 on hypoxia-induced PASMC viability (absorbance at OD450 nm) by CCK8. (B) Relative protein expression of NR4A1 and HIF-1α determined in hypoxia-induced PASMCs. (C) The effects of NR4A1 on PKM2, GLUT1, HK2, and CD36 mRNA levels were evaluated. (D) The effects of NR4A1 on PKM2, GLUT1, HK2, and CD36 levels were measured. (E) Extracellular glucose and lactate levels were determined. n = 3. ****p < 0.0001 vs. oe-NC.
NR4A1 promotes glycolysis in hypoxia-exposed PASMCs by upregulating HIF-1α expression
To investigate the role of NR4A1 in promoting glycolysis by HIF-1α in hypoxia-induced PASMCs, we performed cotransfection experiments using si-NR4A1 and oe-HIF-1α. Transfection with the HIF-1α overexpression vector increased the viability of hypoxic PASMCs compared with the si-NR4A1+oe-NC group (Fig. 5A), indicating that HIF-1α overexpression reverses the inhibitory effect of NR4A1 silencing. Transfection with si-NR4A1 resulted in reduced levels of NR4A1 and HIF-1α in hypoxia-induced PASMCs. Compared to the si-NR4A1+oe-NC, oe-HIF-1α increased the levels of HIF-1α (Fig. 5B). Furthermore, si-NR4A1 transfection resulted in decreased expression of PKM2, GLUT1, HK2, and CD36 compared to si-NC. Compared to the si-NR4A1+oe-NC, oe-HIF-1α counteracted this effect by increasing the expression of PKM2, GLUT1, HK2, and CD36 (Figs. 5C and 5D). In addition, si-NR4A1 transfection resulted in increased extracellular glucose levels and decreased lactate production. Compared to the si-NR4A1+oe-NC, oe-HIF-1α decreased glucose levels and promoted lactate production (Fig. 5E). These findings suggest that NR4A1 promotes cell proliferation and glycolysis in a hypoxic environment by upregulating HIF-1α expression.
Figure 5: NR4A1 induces HIF-1α expression to promote cell proliferation and glycolysis in hypoxia-exposed PASMCs. (A) Hypoxic PASMC viability (absorbance at OD450 nm) was measured by CCK8. (B) Protein levels of NR4A1 and HIF-1α were determined in hypoxia-induced PASMCs. (C) Relative mRNA levels of PKM2, GLUT1, HK2, and CD36 were examined in hypoxia-induced PASMCs. (D) Protein levels of PKM2, GLUT1, HK2, and CD36 were determined in hypoxia-induced PASMCs. (E) Determination of extracellular glucose and lactate levels in hypoxia-induced PASMCs. n = 3. ****p < 0.0001 vs. si-NC. ###p < 0.001, ####p < 0.0001 vs. si-NR4A1+oe-NC.
Glycolysis is a critical metabolic pathway and its dysregulation has been observed in the development of several diseases (Chelakkot et al., 2023). Accumulating reports suggest that glycolysis serves as a key mechanism in PAH by providing the necessary energy and metabolic intermediates for cell proliferation (Liang et al., 2022). In pathological conditions such as PAH, pyruvate dehydrogenase is inhibited, leading to the conversion of pyruvate to lactate via lactate dehydrogenase (Chen et al., 2018). Previous research has shown that increased expression of PFKFB3 drives glycolysis, resulting in ERK1/2 phosphorylation and calpain activation, which promotes PASMC proliferation and collagen synthesis, ultimately leading to pulmonary vascular remodeling (Kovacs et al., 2019). In addition, cannabidiol has been shown to reduce lactate overproduction and alleviate abnormal glycolysis, thereby attenuating PAH pathology (Lu et al., 2021). JMJD1C knockdown has also been shown to reduce the hypoxia-induced accumulation of glycolytic enzymes and lactate in the mouse lung, likely through the modulation of STAT3 signaling (Zhang et al., 2023). In this study, our data demonstrated that NR4A1 may serve as a novel inducer of glycolysis in PAH. We demonstrated that NR4A1 regulates glycolysis in PASMCs under hypoxic conditions by upregulating HIF-1α expression.
Accumulating evidence reveals that NR4A1 was widely involved in glucose metabolism (Deng et al., 2022). A study proposes that NR4A1 upregulates the expression of lncRNA WFDC21P in hepatocellular carcinoma (HCC) cells, which inhibits glycolysis and hinders the development of HCC (Guan et al., 2020). In addition, Serpina3c was found to block the transcription of enolase (ENO1) by suppressing the acetylation of NR4A1, thereby inhibiting glycolysis and ultimately reducing cardiac fibrosis (Ji et al., 2022). There is also evidence that low levels of NR4A1 expression in T cells show enhanced glycolysis and mitochondrial respiration for high metabolic activity (Liebmann et al., 2018). However, NR4A1 was found to increase the transcriptional activity of HIF-1α, which is critical for tumor progression and metastasis (Yoo et al., 2004; Zhang et al., 2020). HIF-1α is stabilized and translocated to the nucleus under hypoxic conditions, activating hypoxia-induced gene transcription, which contributes to glycolytic processes and vascular remodeling (Abe et al., 2017; Wujak et al., 2021). The regulatory role of NR4A1 on HIF-1α has been reported in diseases such as arthritis (Vyawahare et al., 2022), cancer (Deng et al., 2022), and myocardial infarction (Liao et al., 2023). In this study, the significant elevated levels of NR4A1 and HIF-1α were observed in the plasma of patients with PAH, and these two proteins showed a positive correlation. Hypoxia-induced PASMC proliferation may be associated with increased HIF-1α expression and accelerated glycolysis (Li et al., 2023). To further investigate the molecular mechanisms involved, we established a hypoxia-induced PASMC model. We observed that hypoxia promoted the viability of PASMCs, consistent with previous reports (Masson et al., 2022). Furthermore, our results confirmed that hypoxia also led to the upregulation of HIF-1α, PKM2, GLUT1, and HK2 expression in PASMCs (Chen et al., 2016), as well as a decrease in extracellular glucose levels and an increase in lactate levels. GLUT1 is responsible for the transport of glucose into the cells (Koepsell, 2020), while PKM2 and HK2 are key enzymes in the glycolytic pathway. HK2 catalyzes the conversion of glucose to glucose-6-phosphate, and PKM2 mediates the production of pyruvate and ATP from phosphoenolpyruvate (Feng et al., 2020). Our results found that hypoxia induced the upregulation of NR4A1 expression and an interaction between NR4A1 and HIF-1α. Silencing of NR4A1 resulted in the suppression of HIF-1α expression in hypoxia-induced PASMCs, whereas overexpression of NR4A1 led to an increase in HIF-1α expression. Moreover, NR4A1 silencing reduced the proliferation of hypoxic PASMCs, whereas NR4A1 overexpression played the opposite role. HIF-1α overexpression abolished the inhibitory effects of NR4A1 silencing on the viability of hypoxic PASMCs. Additionally, NR4A1 overexpression upregulated the expression of PKM2, GLUT1, HK2, and CD36, decreased extracellular glucose levels and increased lactate levels. CD36 is a transmembrane glycoprotein involved in accelerated glucose metabolism (Luo et al., 2021). Conversely, silencing of NR4A1 had the opposite effect. These results suggest that NR4A1 overexpression enhances hypoxia-induced glycolysis and the improvement of hypoxia-exposed PASMC viability by NR4A1 may be accompanied by an increase in glycolysis. Further investigation revealed that overexpression of HIF-1α reversed the inhibitory effect of NR4A1 silencing on glycolysis, suggesting that NR4A1 mediates hypoxia-induced glycolysis by upregulating HIF-1α.
Notably, the role of NR4A1 in PAH is controversial. Liu et al. (2014) found that the levels of Nur77 (NR4A1) were increased in commercial PASMCs induced by hypoxia (1% O2, 3 h), and overexpression of Nur77 inhibited PASMC proliferation (Liu et al., 2014). Our study further confirmed an increase in NR4A1 levels in the plasma of PAH patients. NR4A1 levels were also observed to increase in commercial PASMCs exposed to hypoxia (3% O2/5% CO2/92% N2, 24 h). Another study showed decrease levels of Nur77 mRNA and protein were observed in isolated PASMCs from PAH patients and hypoxic mice (10% O2, 3 weeks), but an increase in the lung tissue from PAH patients and hypoxic mice (Nie et al., 2016). However, Nie et al. (2016) did not include an analysis of Nur77 expression in isolated PASMCs induced by hypoxia (2.5% O2/4.5% CO2/92% N2, 48 h). Kurakula et al. (2019) found that Nur77 levels were downregulated in MVECs from iPAH and hereditary PAH (HPAH) patients. Silencing Nur77 exacerbated inflammation and proliferation in MVECs, while Nur77 agonist 6-MP restored aberrant vascular remodeling in PAH rats (Kurakula et al., 2019). According to the current reports, NR4A1 exhibits different expressions in PASMCs stimulated under different conditions (different oxygen levels and treatment durations), different expressions in PASMCs from PAH patients and lung/plasma, and different expressions in different cells (PASMCs vs. MVECs). These controversial results may be attributed to different sampling sites, different triggers that simulate PAH, variations in microenvironments created by different models, and changes in regulatory factors surrounding NR4A1 that may result in different outcomes. Therefore, further research is still needed to clarify these issues.
There are still several limitations of this study. The sample size of patients with PAH included in this study was relatively small. In addition, PAH can be induced by various stimuli, and the consequences may differ depending on the specific etiology. The pathological environment represented by the hypoxia treatment of PASMCs may not encompass all pathological phenomena of PAH. Moreover, three biological replicates may still not be considered strict enough. The functional class (II and III) in the included PAH patients does not affect the levels of NR4A1 and HIF-1α in plasma. It is unclear whether differences exist in classes I and IV. It also remains to be determined whether there are differences between functional classes within specific types of PAH. Therefore, future studies should consider including more diverse samples of PAH patients and models with PAH to better understand the broader implications. Furthermore, due to time and financial constraints, we were unable to explore the regulatory mechanism of NR4A1 on PASMC function or establish an animal model of PAH for in vivo validation. Regarding the regulation of glycolysis, relying solely on the expression of glycolysis-related enzyme genes may not be sufficient. Additional evidence, such as measurements of glycolysis rates and examination of hippocampal energy metabolism, needs to be included to gain a deeper understanding of the NR4A1-mediated glycolytic processes. Glucose metabolism is closely linked to mitochondrial function (Rencelj et al., 2021). Exploring the regulation of mitochondrial function by NR4A1 may be an interesting avenue for future investigations to further elucidate the underlying mechanisms involved in the modulation of glycolysis.
In conclusion, this work lies in linking NR4A1 and HIF-1α in the context of PAH and suggests a potential role for glycolysis in the NR4A1/HIF-1α axis-mediated PAH progression. Our study explored for the first time the regulatory relationship between NR4A1 and HIF-1α in PAH, demonstrating that activation of the NR4A1/HIF-1α axis enhances PASMC viability under hypoxic conditions. We provide in vitro evidence that overexpression of NR4A1 enhances glycolysis by affecting the expression of key glycolysis-related regulators. Conversely, silencing of NR4A1 impairs glycolysis by inhibiting HIF-1α expression. These findings suggest that targeting NR4A1 may offer potential therapeutic strategies for the management of PAH.
Acknowledgement: None.
Funding Statement: This study was supported by the National Natural Science Foundation of China (No. 82000300).
Author Contributions: The authors confirm contribution to the paper as follows: conceptualization: C. Chen; funding acquisition: C. Chen; writing-original draft: C. Chen; data curation: J. Wen, W. Huang; formal analysis: J. Wen; visualization: W. Huang; methodology: J. Li; project administration: J. Li. All authors reviewed the results and approved the final version of the manuscript.
Availability of Data and Materials: All data generated or analyzed during this study are included in this published article.
Ethics Approval: The study was approved by The IRB of Third Xiangya Hospital, Central South University (2020-S109). The study was performed in accordance with the Declaration of Helsinki. Written informed consent was obtained, and participants knew and agreed with the usage of their samples.
Conflicts of Interest: The authors declare that they have no conflicts of interest to report regarding the present study.
References
Abe H, Semba H, Takeda N (2017). The roles of hypoxia signaling in the pathogenesis of cardiovascular diseases. Journal of Atherosclerosis and Thrombosis 24: 884–894. [Google Scholar]
Beshay S, Sahay S, Humbert M (2020). Evaluation and management of pulmonary arterial hypertension. Respiratory Medicine 171: 106099. [Google Scholar]
Chandel NS (2021). Glycolysis. Cold Spring Harbor Perspectives in Biology 13: a040535. [Google Scholar]
Chelakkot C, Chelakkot VS, Shin Y, Song K (2023). Modulating glycolysis to improve cancer therapy. International Journal of Molecular Sciences 24: 2606. [Google Scholar]
Chen Z, Liu M, Li L, Chen L (2018). Involvement of the Warburg effect in non-tumor diseases processes. Journal of Cellular Physiology 233: 2839–2849. [Google Scholar]
Chen T, Zhou Q, Tang H, Bozkanat M, Yuan JX, Raj JU, Zhou G (2016). miR-17/20 controls prolyl hydroxylase 2 (PHD2)/hypoxia-inducible factor 1 (HIF1) to regulate pulmonary artery smooth muscle cell proliferation. Journal of the American Heart Association 5: e004510. https://doi.org/10.1161/JAHA.116.004510 [Google Scholar] [CrossRef]
Deng S, Chen B, Huo J, Liu X (2022). Therapeutic potential of NR4A1 in cancer: Focus on metabolism. Frontiers in Oncology 12: 972984. [Google Scholar]
Feng J, Li J, Wu L, Yu Q, Ji J, Wu J, Dai W, Guo C (2020). Emerging roles and the regulation of aerobic glycolysis in hepatocellular carcinoma. Journal of Experimental & Clinical Cancer Research 39: 126. [Google Scholar]
Galie N, McLaughlin VV, Rubin LJ, Simonneau G (2019). An overview of the 6th world symposium on pulmonary hypertension. The European Respiratory Journal 53: 1802148. [Google Scholar]
Guan YF, Huang QL, Ai YL, Chen QT, Zhao WX, Wang XM, Wu Q, Chen HZ (2020). Nur77-activated lncRNA WFDC21P attenuates hepatocarcinogenesis via modulating glycolysis. Oncogene 39: 2408–2423. [Google Scholar]
He Z, Dai L, Zuo Y, Chen Y, Wang H, Zeng H (2022). Hotspots and frontiers in pulmonary arterial hypertension research: A bibliometric and visualization analysis from 2011 to 2020. Bioengineered 13: 14667–14680. [Google Scholar]
Herring JA, Elison WS, Tessem JS (2019). Function of Nr4a orphan nuclear receptors in proliferation, apoptosis and fuel utilization across tissues. Cells 8: 1373. [Google Scholar]
Humbert M, Guignabert C, Bonnet S, Dorfmuller P, Klinger JR et al. (2019). Pathology and pathobiology of pulmonary hypertension: State of the art and research perspectives. The European Respiratory Journal 53: 1801887. [Google Scholar]
Ji JJ, Qian LL, Zhu Y, Jiang Y, Guo JQ, Wu Y, Yang ZW, Yao YY, Ma GS (2022). Kallistatin/Serpina3c inhibits cardiac fibrosis after myocardial infarction by regulating glycolysis via Nr4a1 activation. Biochimica et Biophysica Acta (BBA)-Molecular Basis of Disease 1868: 166441. [Google Scholar]
Kierans SJ, Taylor CT (2021). Regulation of glycolysis by the hypoxia-inducible factor (HIFImplications for cellular physiology. The Journal of Physiology 599: 23–37. [Google Scholar]
Koepsell H (2020). Glucose transporters in brain in health and disease. Pflugers Archiv-European Journal of Physiology 472: 1299–1343. [Google Scholar]
Kovacs L, Cao Y, Han W, Meadows L, Kovacs-Kasa A et al. (2019). PFKFB3 in smooth muscle promotes vascular remodeling in pulmonary arterial hypertension. American Journal of Respiratory and Critical Care Medicine 200: 617–627. [Google Scholar]
Kurakula K, Sun XQ, Happe C, da Silva Goncalves Bos D, Szulcek R et al. (2019). Prevention of progression of pulmonary hypertension by the Nur77 agonist 6-mercaptopurine: Role of BMP signalling. The European Respiratory Journal 54: 1802400. [Google Scholar]
Lau EMT, Giannoulatou E, Celermajer DS, Humbert M (2017). Epidemiology and treatment of pulmonary arterial hypertension. Nature Reviews Cardiology 14: 603–614. [Google Scholar]
Li X, Kumar A, Carmeliet P (2019). Metabolic pathways fueling the endothelial cell drive. Annual Review of Physiology 81: 483–503. [Google Scholar]
Li D, Shao NY, Moonen JR, Zhao Z, Shi M et al. (2021). ALDH1A3 coordinates metabolism with gene regulation in pulmonary arterial hypertension. Circulation 143: 2074–2090. [Google Scholar]
Li M, Ying M, Gu S, Zhou Z, Zhao R (2023). SIRT6 inhibits hypoxia-induced pulmonary arterial smooth muscle cells proliferation via HIF-1α/PDK4 signaling. Life Science 312: 121192. [Google Scholar]
Liang S, Yegambaram M, Wang T, Wang J, Black SM, Tang H (2022). Mitochondrial metabolism, redox, and calcium homeostasis in pulmonary arterial hypertension. Biomedicines 10: 341. [Google Scholar]
Liao J, Shao M, Wang Y, Yang P, Fu D, Liu M, Gao T, Wei K, Li X, Du J (2023). Xuesaitong promotes myocardial angiogenesis in myocardial infarction mice by inhibiting MiR-3158-3p targeting Nur77. Aging 15: 4084–4095. [Google Scholar]
Liebmann M, Hucke S, Koch K, Eschborn M, Ghelman J et al. (2018). Nur77 serves as a molecular brake of the metabolic switch during T cell activation to restrict autoimmunity. Proceedings of the National Academy of Sciences of the United States of America 115: E8017–E8026. [Google Scholar]
Liu R, Xu C, Zhang W, Cao Y, Ye J et al. (2022). FUNDC1-mediated mitophagy and HIF1alpha activation drives pulmonary hypertension during hypoxia. Cell Death & Disease 13: 634. [Google Scholar]
Liu Y, Zhang J, Yi B, Chen M, Qi J, Yin Y, Lu X, Jasmin JF, Sun J (2014). Nur77 suppresses pulmonary artery smooth muscle cell proliferation through inhibition of the STAT3/Pim-1/NFAT pathway. American Journal of Respiratory Cell and Molecular Biology 50: 379–388. [Google Scholar]
Lu X, Zhang J, Liu H, Ma W, Yu L, Tan X, Wang S, Ren F, Li X, Li X (2021). Cannabidiol attenuates pulmonary arterial hypertension by improving vascular smooth muscle cells mitochondrial function. Theranostics 11: 5267–5278. [Google Scholar]
Luo Y, Teng X, Zhang L, Chen J, Liu Z, Chen X, Zhao S, Yang S, Feng J, Yan X (2019). CD146-HIF-1α hypoxic reprogramming drives vascular remodeling and pulmonary arterial hypertension. Nature Communications 10: 3551. [Google Scholar]
Luo X, Zheng E, Wei L, Zeng H, Qin H et al. (2021). The fatty acid receptor CD36 promotes HCC progression through activating Src/PI3K/AKT axis-dependent aerobic glycolysis. Cell Death & Disease 12: 328. [Google Scholar]
Masson B, Le Ribeuz H, Sabourin J, Laubry L, Woodhouse E et al. (2022). Orai1 inhibitors as potential treatments for pulmonary arterial hypertension. Circulation Research 131: e102–e119. [Google Scholar]
Montani D, Girerd B, Jais X, Laveneziana P, Lau EMT et al. (2021). Screening for pulmonary arterial hypertension in adults carrying a BMPR2 mutation. The European Respiratory Journal 58: 2004229. [Google Scholar]
Nie X, Tan J, Dai Y, Mao W, Chen Y, Qin G, Li G, Shen C, Zhao J, Chen J (2016). Nur77 downregulation triggers pulmonary artery smooth muscle cell proliferation and migration in mice with hypoxic pulmonary hypertension via the Axin2-β-catenin signaling pathway. Vascular Pharmacology 87: 230–241. [Google Scholar]
Odagiu L, May J, Boulet S, Baldwin TA, Labrecque N (2020). Role of the orphan nuclear receptor NR4A family in T-cell biology. Frontiers in Endocrinology 11: 624122. [Google Scholar]
Rencelj A, Gvozdenovic N, Cemazar M (2021). MitomiRs: Their roles in mitochondria and importance in cancer cell metabolism. Radiology and Oncology 55: 379–392. [Google Scholar]
Seagroves TN, Ryan HE, Lu H, Wouters BG, Knapp M, Thibault P, Laderoute K, Johnson RS (2001). Transcription factor HIF-1 is a necessary mediator of the Pasteur effect in mammalian cells. Molecular and Cellular Biology 21: 3436–3444. [Google Scholar]
Shah AJ, Vorla M, Kalra DK (2022). Molecular pathways in pulmonary arterial hypertension. International Journal of Molecular Sciences 23: 10001. [Google Scholar]
Shu T, Zhang J, Zhou Y, Chen Z, Li J, Tang Q, Lei W, Xing Y, Wang J, Wang C (2023). Eosinophils protect against pulmonary hypertension through 14-HDHA and 17-HDHA. The European Respiratory Journal 61: 2200582. [Google Scholar]
Stenmark KR, Fagan KA, Frid MG (2006). Hypoxia-induced pulmonary vascular remodeling: Cellular and molecular mechanisms. Circulation Research 99: 675–691. [Google Scholar]
Tirpe AA, Gulei D, Ciortea SM, Crivii C, Berindan-Neagoe I (2019). Hypoxia: Overview on hypoxia-mediated mechanisms with a focus on the role of HIF genes. International Journal of Molecular Sciences 20: 6140. [Google Scholar]
Veith C, Schermuly RT, Brandes RP, Weissmann N (2016). Molecular mechanisms of hypoxia-inducible factor-induced pulmonary arterial smooth muscle cell alterations in pulmonary hypertension. The Journal of Physiology 594: 1167–1177. [Google Scholar]
Vyawahare A, Prakash R, Jori C, Ali A, Raza S, Khan R, Id O (2022). Caffeic acid modified nanomicelles inhibit articular cartilage deterioration and reduce disease severity in experimental inflammatory arthritis. ACS Nano 16: 18579–18591. [Google Scholar]
Wu L, Chen L (2018). Characteristics of Nur77 and its ligands as potential anticancer compounds (Review). Molecular Medicine Reports 18: 4793–4801. [Google Scholar]
Wujak M, Veith C, Wu CY, Wilke T, Kanbagli ZI et al. (2021). Adenylate kinase 4—A key regulator of proliferation and metabolic shift in human pulmonary arterial smooth muscle cells via Akt and HIF-1α signaling pathways. International Journal of Molecular Sciences 22: 10371. [Google Scholar]
Xu W, Janocha AJ, Erzurum SC (2021). Metabolism in pulmonary hypertension. Annual Review of Physiology 83: 551–576. [Google Scholar]
Xu J, Zhong Y, Wang Z (2022). Decrease in tripartite motif containing 24 suppresses hypoxia-induced proliferation and migration of pulmonary arterial smooth muscle cells via the AKT/mammalian target of rapamycin complex 1 pathway. Bioengineered 13: 13596–13606. [Google Scholar]
Yoo YG, Yeo MG, Kim DK, Park H, Lee MO (2004). Novel function of orphan nuclear receptor Nur77 in stabilizing hypoxia-inducible factor-1α. The Journal of Biological Chemistry 279: 53365–53373. [Google Scholar]
Zhang X, Li T, Liu S, Xu Y, Meng M et al. (2020). β-glucan from Lentinus edodes inhibits breast cancer progression via the Nur77/HIF-1α axis. Bioscience Reports 40: BSR20201006. [Google Scholar]
Zhang C, Sun Y, Guo Y, Xu J, Zhao H (2023). JMJD1C promotes smooth muscle cell proliferation by activating glycolysis in pulmonary arterial hypertension. Cell Death Discovery 9: 98. [Google Scholar]
Cite This Article
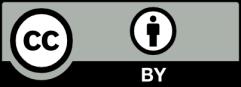