Open Access
REVIEW
Curcumin in inflammatory bowel diseases: Cellular targets and molecular mechanisms
1 Rede Nordeste de Biotecnologia (RENORBIO), Instituto de química e Biotecnologia, Universidade Federal de Alagoas (UFAL), Maceió, 57072-900, Brasil
2 Programa de Pós-graduação em Nutrição (PPGNUT), Faculdade de Nutrição (FANUT), Universidade Federal de Alagoas (UFAL), Maceió, 57072-900, Brasil
3 Programa de Pós-Graduação em Química e Biotecnologia (PPGQB), Instituto de química e Biotecnologia, Universidade Federal de Alagoas (UFAL), Maceió, 57072-900, Brasil
4 Colegiado de Nutrição, Universidade de Pernambuco (UPE), Campus Petrolina, Pernambuco, 56328-900, Brasil
5 Programa de Pós-Graduação em Ciências da SaÚde (PPGCS), Instituto de Ciências da SaÚde, Universidade Federal de Alagoas (UFAL), Maceió, 57072-900, Brasil
6 Programa de Pós-graduação em Ciências Médicas (PPGCM), Faculdade de Medicina, Universidade Federal de Alagoas (UFAL), Maceió, 57072-900, Brasil
* Corresponding Author: FABIANA ANDRÉA MOURA. Email:
# The two first authors contributed equally to this review
BIOCELL 2023, 47(11), 2547-2566. https://doi.org/10.32604/biocell.2023.043253
Received 27 June 2023; Accepted 08 September 2023; Issue published 27 November 2023
Abstract
Curcumin, a natural product, has exhibited promising effects in both animal models and clinical trials, interacting with a multitude of factors linked to Inflammatory Bowel Disease (IBD). These factors encompass cytokines, oxidative stress-associated enzymes, and modulation of the intestinal microbiota. Notably, curcumin has demonstrated therapeutic potential in animal models of colitis, wherein it exerts a negative regulatory influence on pivotal signaling pathways such as PI3/Akt, JAK/STAT, and β-catenin. Moreover, it inhibits the expression of pro-inflammatory enzymes and co-stimulatory molecules (including RANKL, ICAM-1, CD205, CD256, TLR4, among others), while curbing immune cell chemotaxis, thereby attenuating the characteristic neutrophil infiltration observed in IBD. Another facet of curcumin’s action involves its modulation of the intestinal microbiota. Notably, the microbiota itself contributes to beneficial biotrans formations of curcumin, thereby enhancing its effectiveness in IBD treatment. On a clinical front, curcumin has demonstrated the ability to induce clinical and/or endoscopic remission without any reported toxic effects. Hence, curcumin warrants consideration as an adjunctive therapy in IBD management. Subsequent clinical investigations should concentrate on meticulously evaluating curcumin’s impact on these precise therapeutic targets.
Graphic Abstract
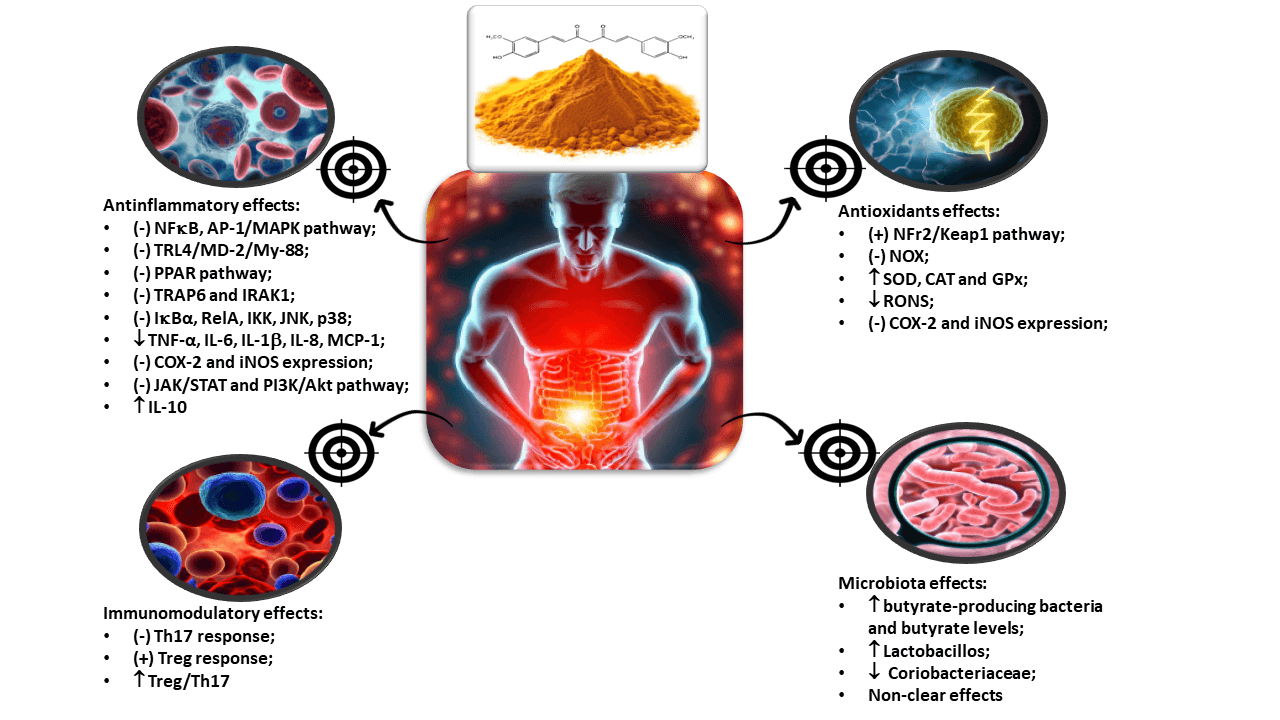
Keywords
Abbreviation List
AP-1 | Activator Protein 1 |
ARE | Antioxidant Response Element |
ATG5 | Autophagy-Related 5 |
BCL-2 | B Cell Lymphoma 2 |
CAT | Catalase |
CRP | C-Reactive Protein |
CD | Crohn’s Disease |
C. Longa | Curcuma Longa |
CUR | Curcumin |
COX | Cyclooxygenases |
DAMP | Damage-Associated Molecular Patterns |
DNA | Deoxyribonucleic Acid |
Nrf2 | Erythroid Nuclear Factor 2 |
γGCL | Gamma-Glutamylcysteine Ligase |
Gpx | Glutathione Peroxidase |
GSH | Glutathione Reduced |
GR | Glutathione Reductase |
GST | Glutathione S-Transferase |
HO-1 | Heme-Oxygenase 1 |
IBD | Inflammatory Bowel Disease |
IFNγ | Interferon-Gamma |
IL | Interleukin |
JAK | Janus Kinases |
JNK | Jun N-Terminal Kinase |
Keap1 | Kelch-Like ECH-Associated Protein |
LPS | Lipopolysaccharide |
LOX | Lipoxygenase |
MDA | Malondialdehyde |
MGO | Methylglyoxal |
MAPK | Mitogen-Activated Protein Kinases |
MCP-1 | Monocyte Chemotactic Protein 1 |
MDR1 | Multidrug Resistance Protein 1 |
MPO | Myeloperoxidase |
NOS | Nitric Oxide Synthase |
Nox | Nitrogen Oxid |
NLRP3 | NOD-Like Receptor Pyrin Domain-Containing 3 |
NF-κB | Nuclear Factor Kappa-B |
PAMP | Pathogen-Associated Molecular Patterns |
Prx | Peroxidase |
PI3K | Phosphatidylinositol-3-Kinase |
PXR | Pregnane X Receptor |
PPAR | Proliferator-Activated Receptor-Gamma |
NQQ1 | Quinone Oxidoreductase 1 |
RNS | Reactive Nitrogen Species |
ROS | Reactive Oxygen Species |
RONS | Reactive Species of Oxygen and Nitrogen |
RIP | Receptor-Interacting Serine/Threonine Kinase |
NADPH | Reduced Nicotinamide Adenine Dinucleotide Phosphate |
SCFAS | Short-Chain Fatty Acids |
STAT | Signal Transducer and Activator of Transcription Proteins |
Srxn1 | Sulfiredoxin |
SOD | Superoxide Dismutase |
Th | T-Helper Cells |
TLRs | Toll-Like Receptors |
TGF-β1 | Transforming Growth Factor β–1 |
TNF-α | Tumor Necrosis Factor—α |
UC | Ulcerative Colitis |
XO | Xanthine Oxidase |
Complementary alternative medicine employs non-traditional therapies provided by professionals extending beyond Western allopathic medicine (Duff et al., 2018). In managing inflammatory bowel disease (IBD), a chronic gastrointestinal inflammatory disorder, alternative or complementary approaches encompass bioactive components from food, psychotherapeutic practices, and hypnosis (Mijan and Lim, 2018; Torres et al., 2019).
These approaches are adopted by 30%–56% of IBD patients, given potential adverse effects of conventional drug treatments (aminosalicylates, corticosteroids, antibiotics, immunomodulators, and biological therapy targeting tumor necrosis factor α—TNF-α) (Schmidt et al., 2010, Torres et al., 2019). Prolonged use of these drugs, associated with the chronicity of the disease, can cause adverse effects, many of them directly affect Gut Intestinal Transit (GIT), leading to dependence and therapeutic failure (Hvas et al., 2018).
Curcuminoids, which include curcumin, demethoxycurcumin, and bisdemethoxycurcumin (Fig. 1), are polyphenols found in the plant species Curcuma longa (C. longa) which is being used for at least 2500 years in traditional Asia and Indian medicine as a food additive, and/or a dye, and/or a condiment (Kocaadam and Sanlier, 2017; Akaberi et al., 2021).
Figure 1: Chemical structures of Curcumidoids present in Curcuma longa.
Studies in animal models have shown that curcuminoids, particularly curcumin, have efficacy in reducing IBD activity (Altinel et al., 2020; Wei et al., 2021) through their antioxidant and anti-inflammatory modulation effects (Cooney et al., 2016; Kocaadam and Sanlier, 2017). In addition, C. longa polyphenols are part of the group of selected phytochemicals studied as a complementary therapy for IBD in randomized clinical trials (Sugimoto et al., 2020; Banerjee et al., 2021). However, unlike studies in animal models, clinical trials have focused more heavily on the anti-colitis action of curcumin and have not given as much attention to its effects on redox and/or anti-inflammatory biochemical markers.
A recent review on curcumin delves into its potential adverse effects, including gallstone formation, moderate anemia, and elevated lipid peroxidation. This underscores the fact that not all mechanisms and cellular targets of this polyphenol are fully understood (Karthikeyan et al., 2021). Another crucial point highlighted in the review conducted by Mazieiro et al. (2018) is the imperative for further clinical trials focusing on curcumin’s impact on IBD. This is primarily due to the lack of knowledge regarding safe and efficacious dosage levels, a challenge compounded by its low bioavailability (Mazieiro et al., 2018).
On this context, this review aims to discuss the effects and mechanisms of action of curcuminoids as an alternative therapy for IBD.
Immunological pathophysiology of IBD
IBD is a chronic disorder marked by periods of remission and symptom exacerbation. IBD comprises Crohn’s disease (CD), which can affect any part of the gastrointestinal tract, and ulcerative colitis (UC), primarily occurring in the rectum, ascending colon, or both (Mahdipour et al., 2019). Globally, IBD exhibits a widespread presence, with a consistent occurrence in developed regions such as Western Europe, the United States, and Canada, and a significant rise in incidence and prevalence in newly industrialized regions (Kaplan et al., 2019). While seldom fatal, severe cases can result in infections, surgeries, and colorectal cancer (Brunet et al., 2018). This disease substantially impacts patients’ quality of life due to its recurrent, chronic symptoms, often emerging during periods of high productivity (Sairenji et al., 2017).
The pathophysiology of IBD requires further investigation, though recent evidence highlights its multifactorial nature, involving external environmental factors, intestinal microbiota, individual genetic predisposition, and immune responses. Inflammation and redox imbalance play crucial roles at the molecular level in the initiation and progression of this disease (Moura et al., 2015).
External contributors to IBD encompass factors like smoking, stress, medication use, and dietary composition. Genetic susceptibility evidence underscores around 240 loci heightening IBD risk, with approximately 30 being common between CD and UC. Gene alterations linked to maintaining intestinal homeostasis, such as epithelial barrier function, immunity, and cellular autophagy, are observed. Intestinal microbiota’s influence on immunological factors is significant, and dysbiosis—favoring pathogenic over commensal bacteria—can lead to metabolic changes impacting gastrointestinal development. Immunological dysregulation, marked by exaggerated immune responses and uncontrolled inflammation, contributes to IBD (Guan, 2019).
A key IBD pathophysiological mechanism involves immune response dysregulation against antigens from luminal content, which normally triggers controlled inflammation. In genetically susceptible hosts, such as in IBD, this homeostatic balance breaks, resulting in persistent inflammation (Lim and Hanauer, 2004). Inflammatory cascade activation involves cell types like macrophages and dendritic cells, stimulating CD4+ T lymphocyte activation and differentiation. These differentiate into T-helper cells (Th) 1, 2, and 17, secreting various cytokines. Th-1 cells release interferon-gamma (IFNγ) and interleukin (IL)-2, IL-12, and IL-18, while Th-2 cells release IL-4, IL-5, IL-6, IL-10, and IL-13 (Piechota-Polanczyk and Fichna, 2014). Thus, the IBD pro-inflammatory signaling relies on the cytokine network and interactions (Ansari et al., 2021).
IL-6 and Tumor Necrosis Factor-alpha (TNF-α) are critical mediators of chronic intestinal inflammation. TNF-α, produced by phagocytes, drives intestinal inflammation, influencing various intestinal cells. TNF-α effects include macrophage activation, enhanced T cell response, adhesion molecule expression, granuloma formation, and neutrophil recruitment. TNF-α can also regulate other inflammatory mediators like IL-6 and IL-1β, amplifying the initial inflammatory cascade (Cukovic-Cavka et al., 2013).
In IBD, oxidative stress, an imbalance favoring oxidants over antioxidants, leads to redox signaling disruption, molecular damage, and apoptosis, along with promoting carcinogenesis. Reactive species interactions with cellular components cause injury and functional impairment, increasing permeability and chronic inflammation in the intestinal mucosa (Balmus et al., 2016). Endogenously generated reactive oxygen species (ROS) and reactive nitrogen species (RNS) involve enzymes like peroxidase (POD), xanthine oxidase (XO), lipoxygenase (LOX), glucose oxidase, myeloperoxidase (MPO), nitric oxide synthase (NOS), cyclooxygenases (COX), and the NOX complex (Martins et al., 2021). XO, particularly active in the intestinal mucosa, contributes to ROS generation and tissue damage. These enzymes play roles in pathological intestinal processes (Beaugerie and Itzkowitz, 2015).
Numerous cell mediators change during IBD. Erythroid nuclear factor 2 (Nrf2), involved in antioxidant and anti-inflammatory responses, has its activity inhibited in IBD. Protein 53 gene (p53) mutations in IBD may indicate early cancerous cell changes in UC patients. Nuclear factor kappa B (NFκB), a transcription factor regulating many pro-inflammatory cytokines and chemokines, has increased expression in IBD (Moura et al., 2020).
The clinical course of IBD is profoundly influenced by shifts in the intestinal microbiota composition. Numerous studies have documented substantial differences between the gut microbiota of IBD patients and healthy individuals, particularly in terms of microbial diversity and the relative abundance of specific bacteria (Ni et al., 2017). Characterization studies of IBD patient microbiota reveal a widespread reduction in biodiversity, coupled with diminished levels of specific taxa like Firmicutes, Bacteroidetes, Lactobacillus, and Eubacterium (Mentella et al., 2020).
The various ways in which the host is impacted by microbial metabolite production are pivotal to IBD pathogenesis. A microbial metabolic pathway with relevance to IBD is the production of short-chain fatty acids (SCFAs) through the fermentation of non-digestible carbohydrates. SCFAs play a crucial role in immune regulation and metabolic homeostasis. Their loss or reduction could potentially disrupt host-microbe interactions, which are essential for intestinal mucosa and immune homeostasis (Ni et al., 2017; Lee and Chang, 2021).
Although intestinal dysbiosis is undeniably associated with IBD, discerning whether these alterations are a cause or consequence of the disease remains challenging. While these changes might not be the primary drivers of IBD development, they do contribute to disease progression by exacerbating and perpetuating the immune-inflammatory process (Wu et al., 2023). This, in turn, leads to tissue damage, impaired wound healing, complications like fibrosis and strictures, and the formation of fistulas and abscesses. Moreover, the decline or even eradication of key commensal microbiota necessary for immune system restoration and intestinal homeostasis further compounds the issue (Quaglio et al., 2022; Yang and Li, 2022).
Integral to the dynamics of IBD is the engagement of innate immunity—the body’s initial defense line—which recognizes invasive pathogens through Toll-like receptors (TLRs). These receptors respond to damage-associated and pathogen-associated molecular patterns (DAMPs and PAMPs), instigating immune responses (Li and Chang, 2021).
Curcumin chemical features and bioavailability
The effects of C. longa may be attributed to curcuminoids, including curcumin, demethoxycurcumin, bisdemethoxycurcumin, and cyclocurcumin, present in approximate proportions of 70%, 17%, 3%, and 10%, respectively (Trujillo et al., 2013; Stanic, 2017; Li and Chang, 2021).
Curcumin has a structure consisting of two methoxy-and two hydroxyl-substituted benzene rings linked to an α,β-unsaturated β-diketone. The other curcuminoids, demethoxycurcumin (4-hydroxycinnamoyl-(4-hydroxy-3-methoxycinnamoyl) methane) and bis-demethoxycurcumin (bis-(4-hydroxy cinnamoyl) methane) (Fig. 1), have curcumin-like structures and differ by the presence or absence of the methoxy groups. Most studies suggest that curcumin is the most active component of C. longa, demonstrating significant anti-inflammatory, antioxidant, anti-tumor, anti-coagulant, antimicrobial, hypoglycemic, neuroprotective, cardioprotective, and other activities in experimental models and clinical trials (Peng et al., 2021).
The β-diketone moiety exhibits keto-enolic tautomerism and cis-trans isomerism (Fig. 2), which can exist in different proportions depending on the medium (Slika and Patra, 2020). In media with acidic and neutral pH values (ranging between 3 and 7), the keto-enolic balance tends to shift towards the enol form. This is because, in addition to the molecule presenting greater planarity, there is an intramolecular hydrogen bond that contributes to the stability of the carbon chain. In the keto form, the substance becomes able to donate hydrogen with ease, since it presents an electron-deficient methylene due to the electrophilic vicinal carbonyl groups. This ability to donate hydrogen atoms is likely the mechanism behind the antioxidant properties of curcumin (Sueth-Santiago et al., 2015). The presence of two phenolic groups can enhance the biological activities of this substance (Slika and Patra, 2020).
Figure 2: (I-A): Enol (CUR-ENOL)-keto (CUR-KETO) tautomerism of curcumin (CUR), along with the organic functions related to some of the biological activities and chemical properties of CUR. (II) Some pathways related to metabolism of CUR. (B) Conjugation, generating CURG and CURS; (C) Representative compound from the auto-oxidation of CUR; (D) Reduction; (E) Some products resulting from degradation (cleavage) of CUR. Adapted from Schneider et al. (2015).
Curcumin has reduced bioavailability, as the compound is absorbed in a precarious manner, rapidly metabolized, and eliminated from the body (Liu et al., 2016) (Fig. 2). The reduced solubility in an aqueous environment contributes to this process (Prasad et al., 2014). Some studies have evaluated serum curcumin levels after administration by different routes (Yang et al., 2007; Sun et al., 2013; Cheng et al., 2019). While oral administration resulted in reduced serum concentrations in animal models (Yang et al., 2007) and humans (Cheng et al., 2019), intravenous administration in rats resulted in higher serum concentrations (Sun et al., 2013). These studies point to the influence of the route of administration on the bioavailability of this polyphenol (Prasad et al., 2014). Curcumin is metabolized in two phases—reduction and conjugation—resulting in different metabolites (Kunnumakkara et al., 2017; Kotha and Luthria, 2019) (Fig. 2).
Curcumin metabolism unfolds in the liver, intestine, and gut microbiota. Its limited stability under physiological conditions leads to reactions like solvolysis (resulting in 90% degradation), photodegradation, and self-oxidation, producing ferulic acid, ferulic aldehyde, vanillin, vanillic acid, and others (Dei Cas and Ghidoni, 2019) (Fig. 2).
Upon absorption, curcumin undergoes first and second-pass metabolisms in the liver and intestine, mainly through reduction (phase I) and conjugation (phase II). Phase I involves di-, tetra-, hexa-, and octahydrocurcumin formation. In phase II, curcumin and phase I metabolites conjugate with monoglucuronide/sulfate, forming sulfate or glucuronide of curcumin/di-, tetra-, hexa-, and octahydrocurcumin (Anand et al., 2007). Curcumin and metabolites from the first pathway can undergo further conjugation, forming di-glucuronides, tetra-, hexa-, and octahydrocurcumin, and di-, tetra-, hexa-, and octahydrocurcumin sulfates (Nelson et al., 2017).
Curcumin’s pharmacological effects stem from its modulation of various targets and molecular pathways. It curtails inflammation by inhibiting the nuclear factor kappa-B (NF-κB) pathway via binding to peroxisome proliferator-activated receptor-gamma (PPAR) and TLRs. Additionally, it negatively regulates activator protein 1 (AP-1), mitogen-activated protein kinases (MAPK), and p53 pathways (Zhang et al., 2019). NOD-like receptor pyrin domain-containing 3 (NLRP3), involved in cytokine maturation via caspase-1, is directly compromised by curcumin through assembly prevention and indirectly via NFκB blockade (Hasanzadeh et al., 2020).
Curcumin also exhibits effects on cell death that do not rely on caspases (necroptosis), which is inherently linked to increased inflammatory activity. In an in vitro model involving intestinal epithelial cells (human colon cancer HT-29 cells), curcumin inhibited the activity of receptor-interacting serine/threonine kinase (RIP) 1. As a result, the activation of NFκB and MAPKs was suppressed, leading to cellular survival (Zhong et al., 2023).
Curcumin’s effects encompass inhibition of signaling pathways, proteins, and enzymes like Janus kinases/signal transducer and activator of transcription proteins (JAK/STAT), phosphatidylinositol-3-kinase/protein kinase B (PI3K/Akt), jun n-terminal kinase (JNK), COX, and transforming growth factor β–1 (TGF-β1). This leads to diminished cytokines and chemokines with inflammatory properties, including TNF-α, IL-1β, IL-6, IL-8, and monocyte chemotactic protein 1 (MCP-1) (Ashrafizadeh et al., 2020). C-reactive protein (CRP) and TNF-α, inflammatory mediators, are also reduced by curcumin (Alizadeh et al., 2018). Moreover, curcumin curbs inflammation by acting on immune cells, inhibiting the IL-23/Th17 pathway, maintaining Treg/Th17 balance (Peng et al., 2021).
Beyond its anti-inflammatory effects, curcumin lessens oxidative stress by inducing the Nrf2-Kelch-like ECH-associated protein (Keap1) signaling pathway (Fig. 3F), triggering antioxidant defense mechanisms that shield cells from ROS-induced oxidative damage. It also directly reacts with reduced nicotinamide adenine dinucleotide phosphate (NADPH) oxidase and others (Fig. 3B), elevating antioxidant enzyme levels like catalase (CAT), superoxide dismutase (SOD), and glutathione peroxidase (GPx) (Lin et al., 2019) (Fig. 3). Both mechanisms (antioxidant and anti-inflammatory) are interconnected.
Figure 3: Direct and indirect antioxidant effects of Curcumin (CUR). (A) Metal chelation, especially Zn, Cu, Fe; (B) protein-binding, through Michael addition, mainly of oxidant enzymes (lipoxygenase—LOX—and LOX-1, nitric oxide synthase—NOS, myeloperoxidase—MPO, nitrogen oxid—NOx, and others), responsible for generating of reactive species of oxygen and nitrogen (RONS), thus regulating indirectly oxidative stress; (C) direct scavenging of RONS and some carbonyl derivatives (MGO—methylglyoxal); (D) organic moieties responsible for radical, ionic and molecular species capture; (E) mitochondrial protection; (F) generation of antioxidant enzymes (peroxidases—PrX, superoxide dismutase—SOD, catalase—CAT, glutathione peroxidase—GPx, glutathione reductase—GR, heme-oxygenase 1—HO-1, glutathione S-transferase—GST, quinone oxidoreductase 1—NQQ1, gamma-glutamylcysteine ligase—γGCL, sulfiredoxin—Srxn1, and thioredoxin—TrX) through nuclear translocation of erythroid nuclear factor 2/antioxidant response element (Nrf2/ARE); (G) inhibition of nuclear factor kappa-B (NF-κB), decreasing oxidative stress. Adapted from Trujillo et al. (2014), Schneider et al. (2015), Sueth-Santiago et al. (2015), Sun et al. (2016), Costantino et al. (2022).
Curcumin’s distinctive chemical structure (Figs. 1 and 2)—the phenolic hydroxyl groups acting as a hydrogen donor, the α,β-unsaturated carbonyl group as a Michael acceptor, and the adjacent methoxy groups conferring metal-chelating ability (Fig. 3)—underlies its biological properties. These reactive sites enable curcumin’s interaction with biomolecules, modulating proteins, enzymes, and transcription factors, leading to anti-inflammatory, antioxidant, and anti-cancer effects. The methoxy group is proposed as the preferred site for hydrogen donation due to weakened carbon-hydrogen bonds. The behavior as a Michael reaction acceptor (Fig. 3B) reactions occur between the β-unsaturated β-diketone and nucleophiles (-OH, -SH, -SeH, aminoacids residues), contributing to its antioxidant action (Fig. 3A), through deactivation of some oxidant enzymes. Curcumin’s metal-chelating ability (Fig. 3A) is harnessed for various therapeutic purposes (Garcia-Nino and Pedraza-Chaverri, 2014; Wanninger et al., 2015) including Alzheimer’s disease, arthritis, cancer, neurological, metabolic, cardiovascular diseases, psoriasis, COVID-19, and inflammatory disorders (Patel et al., 2020; Peng et al., 2021).
The therapeutic use of turmeric/curcuminoid in humans is considered safe, as evidenced by studies involving high doses (Ryan et al., 2013). Adverse events are rare and include dermatitis and platelet activation suppression, although the latter is not fully understood. Given its intrinsic relation to the dose, as demonstrated by Rukoyatkina et al. (2021) in their in vitro study, high concentrations of this polyphenol (50 µM) reduced platelet viability but in low doses (5 µM), it potentiates platelet autophagy (Rukoyatkina et al., 2021).
In a recent review, the role of curcumin as an anti-thrombotic agent was discussed in the context of conditions involving thromboembolism, atherothrombosis, leukemia, and cardiovascular diseases. This review suggests the need for conducting clinical trials to evaluate the therapeutic potential of this bioactive compound (Hussain et al., 2022). Furthermore, important questions such as the combination of this therapy with natural products and conventional drugs, whether pro-thrombotic or anti-thrombotic, need to be further explored to better understand the safety of curcumin in these situations.
A population-level study in India found curcumin intake up to 100 mg/day safe and associated with reduced cancer prevalence (Jutooru et al., 2010) FAO/WHO Joint Expert Committee on Food Additives (JECFA) considers 4 to 10 g of turmeric daily safe for healthy adults (Kocaadam and Sanlier, 2017).
Apart from curcumin extract, turmeric oil has also been subject to testing in human trials (Table 1). Doses of 0.6 mL/d, administered three times a day for one month, and 1 mL/d, divided into three doses over two months, were assessed for their impact on haemoglobin, blood counts, liver and kidney functions, bleeding and clotting time and serum electrolytes. Over a 12-week observation period, no discernible alterations were noted in heart rate, blood pressure, tolerability, body weight, or signs and symptoms, reaffirming its safety in humans (Joshi et al., 2003).
The assessment of curcumin toxicity finds more comprehensive exploration through animal models. In a study, a dosage equivalent to 20 times the therapeutic recommendation for humans was administered to treat arthritis in female rats, both orally and intraperitoneally. Intriguingly, oral administration exhibited no discernible toxicity, albeit with modest protective effects. In contrast, intraperitoneal administration significantly escalated morbidity and mortality among the animals (Funk et al., 2010). Conversely, a dose of curcuminoids powder extract 25 times higher than the safe dosage (250 mg/kg body weight/day for 6 months) administered orally induced elevated serum levels of alkaline phosphatase and liver weight, but had no effect on hematological parameters or other liver enzymes (Chavalittumrong et al., 2002).
However, in recent years, concerns regarding curcumin’s hepatotoxicity have come to light (Lukefahr et al., 2018; Sohal et al., 2021). These case reports have triggered discussions regarding the quality of this polyphenol available in commercial outlets, as well as the formulations aimed at enhancing its bioavailability (Bucchini, 2019; Girme et al., 2020). In light of these emerging hepatotoxicity questions, Pancholi et al. (2021) evaluated a new curcumin formulation, the curcumin-galactomannoside complex (CGM), in healthy volunteers at a dosage of 1000 mg per day (∼380 mg curcuminoids). Following a 90-day intervention, the authors concluded that CGM was safe, as no discernible alterations in hepatic markers were identified (Pancholi et al., 2021).
According to Qiu et al. (2016), the potential mechanism underlying the hepatotoxic action of curcumin in mega-doses could involve the pronounced overexpression of ROS and IL-6, alongside a reduction in SOD and glutathione-S-transferase levels. This proposed mechanism suggests that the excessive intake of curcumin might disrupt the delicate balance between oxidative stress and antioxidant defenses within the liver. The overproduction of reactive oxygen species and the subsequent elevation of pro-inflammatory IL-6 could contribute to cellular damage and inflammation. At the same time, the diminished activity of SOD and Glutathione S-transferase (GST) enzymes, might compromise the liver’s ability to counteract the damaging effects of oxidative stress (Qiu et al., 2016).
Special physiological situations, such as pregnancy and lactation, lack studies on safe dosages of curcumin. Recent reviews have explored the potential applications of this polyphenol in pregnancy-related conditions, such as preeclampsia, gestational diabetes, intrauterine growth, and more. However, all these scenarios have been tested only in vitro or in animal models, yielding favorable results. Nonetheless, no human studies have assessed the safety and effectiveness, making impractical to recommend this compound during high-risk pregnancies or otherwise (Filardi et al., 2020; Ghaneifar et al., 2020; Tossetta et al., 2021).
Regarding the impact of curcumin on breastfeeding, studies are even scarcer. Kobayashi et al. (2021) evaluated the influence of curcumin on milk production in lactating mammary epithelial cells (MECs) and observed a downregulation in milk production without triggering increased inflammatory activity. This phenomenon could be beneficial in certain situations where reducing breast milk production is desired, such as in cases of galactorrhea and hyperprolactinemia (Kobayashi et al., 2021).
Topical use of curcumin was tested by Afshariani et al. (2014) for the treatment of mastitis in breastfeeding women. In this randomized clinical trial, curcumin was found to successfully decrease markers of lactational mastitis, including pain, breast tension, and erythema within 72 h of administration, without any side effects. Similarly, oral use of turmeric in combination with fenugreek and ginger (3 capsules, 3 times/day for 4 weeks—100 mg turmeric per capsule) was investigated by Bumrungpert et al. (2018) in a randomized clinical trial. This combination exhibited promising effects within 2 weeks (49% increase in breast milk volume), and by 4 weeks, an increase of 103% was observed, all without any adverse effects. While curcumin shows potential in breastfeeding support, the available literature lacks sufficient information to confirm a safe dosage (Bumrungpert et al., 2018).
Therefore, in both pregnancy and breastfeeding contexts, the use of curcumin remains a subject that requires further rigorous investigation to ascertain its safety and efficacy, especially in human populations.
Due to its hydrophobicity, curcumin is insoluble in physiological media, where it rapidly degrades or self-oxidizes, impairing its absorption (Fig. 2). Furthermore, in the bloodstream, it is immediately metabolized in part by the action of glycoprotein-P, a transmembrane protein that depends on adenosine triphosphate (ATP) and leads to the efflux of curcumin, as well as by enzymes of first-pass metabolism in the liver and small intestine, which reduce it to its metabolites di-, tetra-, hexa-, and octa-hydrocurcumin (Fig. 2). When passing through second-pass metabolism, curcumin and its products are conjugated to glucuronide and sulfate, forming inactive products (Dei Cas and Ghidoni, 2019; Ipar et al., 2019), However, some studies show that curcumin metabolites exhibit biological activity (Heger et al., 2014; Edwards et al., 2017). The low bioavailability, rapid degradation, and physical-chemical instability of curcumin have been attributed to the β-diketone portion of the molecule (Peng et al., 2021).
Numerous studies have evaluated and demonstrated a wide variability in the systemic distribution of curcumin. In healthy individuals who received doses of curcumin ranging from 0.5 to 12 g/d, serum levels of this curcuminoid were only noticeable in volunteers who received doses higher than 4 g/d (Lao et al., 2006). In patients with pancreatic cancer who supplemented with 8 g/d of curcumin, only their conjugated metabolic forms were detected in plasma (Chen et al., 2020). Conversely, the concentration of curcumin in colonic tissue was found to be higher than the plasma levels of this polyphenol after supplementation with doses ranging from 3 to 66 g of curcumin per day (Garcea et al., 2005) and with a dose of 3.25 g (Irving et al., 2013), suggesting the potential pharmacological significance of the large intestine for curcumin’s effects.
Efforts to augment curcumin’s bioavailability have led to diverse formulations modifying its solubility, circulation time, permeability, and metabolic stability. These encompass associations with adjuvants, polymers, nanoparticles, liposomes, micelles, phospholipids, metal complexation, and derivatives/analogues (Prasad et al., 2014; Kotha and Luthria, 2019). Recently, nanotechnology has been explored as a drug delivery system for therapeutic agents with bioavailability issues, such as curcumin. Examples of nanoparticles used include poly (butyl-cyanoacrylate) (PBCA), poly (lactide-co-glycolide) (PLGA), chitosan, albumin, and solid lipids (Slika and Patra, 2020).
Among the compounds investigated for adjuvant therapy, piperine, a component of black pepper (Piper nigrum), stands out. Piperine has been shown to prevent the degradation of curcumin and increase its concentration in aqueous solution by interacting with and inhibiting P-glycoprotein and glucuronidation in the liver and intestine, improving its bioavailability by up to 2000% (Singh et al., 2013; Hewlings and Kalman, 2017; Ipar et al., 2019). When 2 g of curcumin was administered to healthy adults, low plasma levels (0.006 ± 0.005 μg/mL, within 1 h) were observed. However, when this dosage was combined with 20 mg of piperine, the concentrations increased significantly (0.18 ± 0.16 μg/mL, up to 0.75 h) (Shoba et al., 1998). Some studies have demonstrated the positive effects of this formulation in certain pathophysiological conditions, including metabolic syndrome, chronic pulmonary complications, and type II diabetes mellitus (Panahi et al., 2015, 2016, 2018).
As mentioned, curcumin is a promising candidate for the treatment of IBD and has been shown to exert anti-inflammatory and antioxidant actions (Figs. 2 and 3) through the modulation of multiple targets and pathways involved in the pathophysiology of these diseases. The interruption of intestinal homeostasis and dysregulation of cytokine pathways, as discussed earlier, contribute to the inflammatory responses observed in IBD (Friedrich et al., 2019). Additionally, the oxidative stress-induced damage to cellular components further exacerbates tissue injury (Moura et al., 2020). On this context, curcumin’s ability to target and modulate these cellular mechanisms and cytokine networks provides a rationale for its therapeutic potential in IBD. By exerting its anti-inflammatory and antioxidant effects, curcumin may help restore intestinal homeostasis and mitigate the pathophysiological processes underlying IBD.
Evidence in the literature suggests that the TLR4/NF-κB/AP-1 signaling pathways are overactivated IBD. Both in vitro and in vivo studies have consistently reported that curcumin reduces the levels of pro-inflammatory cytokines, such as TNF-α, IL-6, IL-1β, and IL-8, by inhibiting these pathways, thereby improving intestinal inflammation in patients with IBD (Zhao et al., 2016; Wang et al., 2018; Burge et al., 2019). Curcumin exerts its effects by suppressing NFκB translocation, inhibiting MAPK phosphorylation, and reducing the expression of inflammatory mediators and reactive oxygen and nitrogen species (RONS). This modulation also impairs the activity of dendritic cells and macrophages that induce Th1 and Th17 responses. Animal models of induced colitis have further demonstrated the efficacy of curcumin in attenuating colonic injury, inflammation markers, lipid peroxidation, and cell death through modulation of the p38 MAPK and TLR4-MyD88/NFκB pathways (Lubbad et al., 2009; Topcu-Tarladacalisir et al., 2013; Li et al., 2015). Additionally, curcumin promotes the expansion of regulatory T cells (Treg) and increases the levels of anti-inflammatory products, such as IL-10 and TGF-β (Zeng et al., 2013; Wang et al., 2018).
The action of curcumin on NF-κB, coupled with its inhibition of the Notch-1 gene, confers to this compound an action that goes beyond anti-inflammatory effects, encompassing inhibition of angiogenesis and tumorigenesis. This multifaceted action is supported by numerous in vitro studies (Wang et al., 2006; Li et al., 2012; Zhdanovskaya et al., 2022).
An important consequence of curcumin’s action on NF-κB and TNF-α is the improvement in insulin resistance, leading to a subsequent reduction in glycemic levels. Several in vivo studies conducted both in animal models (Zou et al., 2021; Lee et al., 2022; Zhong et al., 2022) and in humans (Thota et al., 2018; Marton et al., 2021) have consistently confirmed the hypoglycemic potential of this bioactive compound. In addition to the previously mentioned NF-κB and TNF-α inhibition, it is suggested that curcumin’s action includes the suppression of Notch‑1 signaling and the modulation of intestinal microbiota (Zhao et al., 2018; Zhong et al., 2022).
TNF-α, produced by phagocytes, monocytes, and T cells, has multiple effects on various intestinal cells, including cell proliferation and differentiation. This cytokine can stimulate the production of IL-1, IL-6, and fibroblasts, as well as the expression of other molecules (Guan, 2019). Excessive cytokine production may disrupt the functionality and integrity of the intestinal barrier by inducing apoptosis in epithelial cells (Friedrich et al., 2019). IL-6 is produced by macrophages, T cells in the lamina propria (Mudter and Neurath, 2007), as well as epithelial and mesenchymal cells (Ansari et al., 2021). These latter two cell types play a crucial role in the healing process by recruiting polymorphonuclear leukocytes and macrophages. IL-6 also prevents apoptosis of T cells (Zmora et al., 2017) and its elevated levels have been associated with increased inflammatory activity, as indicated by elevated levels of fecal calprotectin (Bourgonje et al., 2018) or disease activity (Slowinska-Solnica et al., 2021).
IL-10, a critical anti-inflammatory cytokine, is produced by activated innate or adaptive immune system cells, primarily macrophages (Ip et al., 2017). This cytokine regulates the production of pro-inflammatory cytokines and modulates the genetic expression of co-stimulatory molecules and chemokines. Mice genetically modified to lack IL-10 or the IL-10 receptor beta (IL-10Rβ) spontaneously develop enterocolitis (Wei et al., 2020). Therefore, the stimulatory effect of curcumin on IL-10 may provide a protective effect against inflammation. Other cytokines, such as IL-4, IL-10, and IL-13, also appear to be molecular targets of curcumin, with their expression or levels being reduced by this curcuminoid (Ukil et al., 2003; Jian et al., 2005; Gopu et al., 2015; Zhang et al., 2016; Zhao et al., 2017; Kesharwani et al., 2018; Wei et al., 2021).
Additionally, the administration of curcumin has demonstrated therapeutic potential by negatively regulating various signaling pathways (PI3/Akt, JAK/STAT, and β-catenin), inhibiting the expression of COX-2, iNOS, and co-stimulatory molecules (RANKL, ICAM-1, CD205, CD256, TLR4, among others), and suppressing immune cell chemotaxis, including the infiltration of neutrophils into inflamed regions (Zhao et al., 2016; Wang et al., 2018; Karthikeyan et al., 2021). These effects contribute to the reduction of tissue damage. Several studies have supported these findings, including a model of 2,4,6-trinitrobenzenesulfonic acid (TNBS)-induced colitis, where curcumin decreased the expression of pro-inflammatory cytokines (TNF-α and IFN-γ) by inhibiting COX-2 expression (Jiang et al., 2006). The therapeutic effects of curcuminoids were also observed in other experimental models, attributed to the blockade of the STAT3 pathway (Liu et al., 2013).
COX is an enzyme involved in the endogenous generation of ROS, while COX-2 is specifically associated with the formation of ulcers (Balmus et al., 2016). Ulcer formation is closely linked to the development of precancerous features in the colon (Tian et al., 2017). On the other hand, inducible nitric oxide synthetase (iNOS) is an enzyme highly expressed in inflammatory cells in response to immune system stimuli and cytokines. The iNOS catalyzes the production of nitric oxide (•NO) from L-arginine, which is normally produced under physiological conditions. However, in cases of IBD, where there is an excessive production of •NO, various cell types, including epithelial ones, can be affected, leading to vasodilation, formation of edema, and alteration of mucosal permeability (Kamalian et al., 2020). Studies using cell and animal models of colitis have indicated that curcumin treatment significantly reduces iNOS activity and tissue levels of •NO (Camacho-Barquero et al., 2007; Venkataranganna et al., 2007; Mouzaoui et al., 2020).
In addition, curcumin’s protective effect is also attributed to its ability to inhibit NLRP-3 inflammasome activation and reduce IL-1β production, thereby resulting in improvements in weight loss, disease activity index, and colon length (Gong et al., 2018; Yue et al., 2019). Although the precise role of IL-1β in intestinal inflammation remains uncertain, it should be noted that patients treated with IL-1β receptor inhibitors did not show a favorable response (Mao et al., 2018). Nonetheless, experimental studies have demonstrated elevated levels of this cytokine in colitis models (Liu et al., 2013; Gopu et al., 2015).
In IBD, histologically characteristic features include the infiltration and accumulation of immune cells, such as neutrophils and monocytes, which release MPO (Altinel et al., 2020). This enzyme is associated with both the inflammatory process and oxidative mechanisms in the inflamed colon, including the generation of ROS such as hypochlorous acid (HOCl) through halogenation cycles or peroxidase (Chami et al., 2018). Curcumin has been shown to inhibit MPO activity (Salh et al., 2003; Ukil et al., 2003; Deguchi et al., 2007; Venkataranganna et al., 2007; Lubbad et al., 2009; Liu et al., 2013; Zeng et al., 2013; Gopu et al., 2015; Bastaki et al., 2016; Yildirim et al., 2016; Zhang et al., 2016; Altinel et al., 2020), suggesting its potential antioxidant and anti-inflammatory effects, which may potentially reduce immune cell infiltration and mucosal changes caused by intestinal inflammation.
In a murine model of colitis, curcumin was able to normalize the expression of autophagy-associated genes (Beclin-1, LC-3II, autophagy-related 5—ATG5, B cell lymphoma 2—BCL-2), leading to disease improvement. Additionally, it reduced the formation of autophagosomes in colonocytes and the levels of IL-17, IL-6, and TNF-α, promoting anti-inflammatory effects, as well as restoring IL-10 expression (Yue et al., 2019).
Due to its antioxidant action, curcumin has been found to be effective in combating oxidative stress present in IBD. Several experimental studies have demonstrated that this polyphenol significantly reduces levels of malondialdehyde (MDA), HOCl, hydrogen peroxide (H2O2), and •NO, while increasing the activity of SOD and other phase II enzymes involved in xenobiotic detoxification processes mediated by the Nrf2 pathway, which is known to regulate antioxidant responses (Mouzaoui et al., 2012, 2020; Goulart et al., 2021; Lucas et al., 2021) (Fig. 3).
Another therapeutic target of curcumin is the pregnane X receptor (PXR), a nuclear receptor that regulates inflammatory responses, glucose metabolism, and protects normal cells against carcinogenesis. Curcumin appears to activate the human PXR and significantly reduce the histological signs of colonic inflammation through the induction of multidrug resistance protein 1 (MDR1) expression. MDR1 is involved in the transport of various xenobiotics, toxins, as well as physiological substrates (Niu et al., 2022).
As previously noted, oxidative stress plays a decisive role in the pathophysiology of IBD, leading to cellular and molecular damage in various components such as membrane lipids, proteins, and deoxyribonucleic acid (DNA) in mitochondria and nuclei, ultimately resulting in tissue injury (Tian et al., 2017; Dudzinska et al., 2018). The inhibition of lipid peroxidation by curcumin is crucial as it prevents the accumulation of MDA, a metabolite generated during lipid peroxidation. This process is significant because lipid peroxidation not only disrupts the cellular environment’s metabolism and balance but also results in the production of harmful end products that can damage protein complexes (Alemany-Cosme et al., 2021). The distinct antioxidant mechanisms of curcumin can be visualized in Fig. 3.
In animal models of colitis, supplementation with curcumin has been shown to increase or normalize the activity of SOD, CAT, and/or GPx enzymes in colonic tissue and/or serum, compared to groups without supplementation (Topcu-Tarladacalisir et al., 2013; Gopu et al., 2015). Furthermore, curcumin has been observed to elevate serum or tissue glutathione reduced (GSH) levels (Gopu et al., 2015; Bastaki et al., 2016). These findings indicate that apart from its direct effects on pro-oxidant enzymes and reactive species, curcumin can alleviate oxidative stress by stimulating the production of both enzymatic and non-enzymatic antioxidant complexes.
The effects and molecular targets of curcumin in IBD are listed in Table 2.
As discussed previously, oxidative stress and inflammation play crucial roles in initiating, progressing, and sustaining tissue damage that leads to intestinal barrier dysfunction and increased permeability. This dysfunction results in the loss of immune tolerance to microbiota, making the organism susceptible to attacks by various antigens from the lumen, primarily bacteria and their products such as lipopolysaccharide (LPS) (Pasternak et al., 2011). In this regard, curcumin and its metabolites generated in the gut exhibit biological activity and promote beneficial effects on the intestinal microbiota.
Several animal models have demonstrated positive effects on the microbiota in induced colitis, such as increased abundance of butyrate-producing bacteria and fecal butyrate levels (Ohno et al., 2017), as well as increased relative abundance of Lactobacillus and decreased Coriobacteriaceae order (McFadden et al., 2015). These effects suggest two distinct influences of curcumin on the microbiota: firstly, curcumin modulates microbiota composition, and secondly, microbiota causes biotransformation of curcumin (Scazzocchio et al., 2020).
However, human studies in this area are still limited and have presented conflicting results. In a double-blind, randomized, placebo-controlled pilot study, Peterson et al. (2018) compared the administration of 1000 mg of C, longa + 1.25 mg black pepper or 1000 mg of curcumin + 1.25 mg black pepper vs. placebo. According to the authors, both turmeric and curcumin altered the gut microbiota in a highly similar manner in healthy subjects. Nevertheless, there were some concerns regarding the study, including the small number of subjects evaluated (3 placebo, 6 turmeric, and 5 curcumin), the absence of a controlled diet group, and the potential for piperine to promote the growth of certain gut microbiota (Peterson et al., 2018).
More recently, Lopresti et al. (2021) conducted a double-blind, randomized, placebo-controlled study to test the efficacy of a curcumin extract in adults with self-reported digestive complaints. After 8 weeks of treatment (500 mg curcumin extract vs. placebo), post-stool samples were available for 50 participants (25 per group). The study did not observe a significant effect on small intestinal bacterial overgrowth (SIBO) and intestinal microbial profile. However, the authors suggest conducting more detailed microbial analyses to examine the effects of curcumin on intestinal barrier function, inflammation, neurotransmitter activity, and visceral sensitivity (Lopresti et al., 2021).
In a review by Jabczyk et al. (2021), discussing the impact of curcumin on the microbiota, the authors state that the benefits of curcumin on the microbiota depend on its dietary and supplementary intake, an individual’s capacity for metabolism, and the biodiversity of their microbiota. Consequently, the influence of curcumin on the intestinal microbiota remains unclear. On this context, curcumin metabolites may exhibit higher bioactivity than the parent compound (Jabczyk et al., 2021).
The anti-colitis efficacy of curcumin can be attributed to its multitarget action, which includes the modulation of inflammation and oxidative stress markers, regulation of microbiota and intestinal immune function, and improvement of mucosal barrier dysfunction, among other mechanisms. Experimental studies have demonstrated improvements in disease activity, macroscopic and histological evaluations of the colon, and a reduction in animal mortality (Ohno et al., 2017; Yang et al., 2018).
Another significant aspect of curcumin’s action revolves around its interaction with Escherichia coli, a bacterium abundantly found within the human microbiota. Notably, a substantial proportion of ingested curcumin reaches the cecum and colon regions. Here, it undergoes a conversion process facilitated by the enzyme NADPH reductase, which is present in human fecal E. coli. This enzyme orchestrates the stepwise reduction of the four double bonds within curcumin’s heptadiene-3,5-dione structure, resulting in the formation of dihydrocurcumin and subsequently tetrahydrocurcumin (Hassaninasab et al., 2011).
Numerous studies have demonstrated that the use of curcumin as an adjuvant agent is safe and effective in the treatment of IBD. These studies have reported beneficial effects on clinical symptoms, endoscopic remission, and reduction of certain inflammation markers (Burge et al., 2019; Cunha Neto et al., 2019; Fallahi et al., 2021).
Sugimoto et al. (2020) conducted pioneering research on the therapeutic use of curcumin for the treatment of mild to moderate CD. A total of 30 patients from five independent medical centers were enrolled in the study and received Theracurmin®, an orally administered formulation containing 360 mg/day of curcumin, or a placebo for a duration of 12 weeks. It is important to note that Theracurmin® has a significantly higher absorption rate compared to curcumin powder, being 27 times more bioavailable. The effects of the treatment were assessed based on clinical, endoscopic, healing, and laboratory parameters. The study’s findings revealed significant clinical and endoscopic improvements, reduced disease activity, and anal healing in the treatment group. The endoscopic remission rate was 15% in the curcumin group, while no remission was observed in the placebo group. Importantly, no serious adverse effects were reported, indicating the safety of Theracurmin® as a treatment option for CD (Sugimoto et al., 2020).
The number of clinical trials investigating curcumin has been increasing, leading to the conduction of several meta-analyses, including a recent one conducted by our research group (Alves et al., 2022). However, despite the reported positive effects, several critical factors and the absence of evaluation of more robust markers may limit our conclusions, as can be seen below.
Similarly, a randomized, double-blind, placebo-controlled study demonstrated that the administration of 1500 mg of curcumin for 8 weeks, in conjunction with pharmacological therapy, resulted in improved clinical parameters and reduced levels of CRP and erythrocyte sedimentation rate in patients with mild to moderate ulcerative colitis (UC), without any changes in TNF-α levels (Sadeghi et al., 2020).
Masoodi et al. (2018) investigated the use of curcuminoid nanomicelles (80 mg, orally three times a day) for 4 weeks in patients with mild to moderate ulcerative colitis. Due to its better bioavailability, curcuminoid nanomicelles were used. Although improvements in clinical variables were observed, the study did not include endoscopic investigation or evaluation of other important laboratory and inflammatory markers (Masoodi et al., 2018). Sadeghi et al. (2020) conducted a study with 70 patients using 1500 mg/day of curcumin as adjunctive therapy. The intervention group showed clinical remission, improved disease activity, and reduced inflammatory markers compared to the control group, indicating a positive response. However, the specific drug and dosage used in each group were not described (Sadeghi et al., 2020).
Banerjee et al. (2021) evaluated a novel bioenhanced hydrophilic curcumin compound (BEC) as adjuvant therapy in ulcerative colitis patients. Treatment with BEC induced clinical and endoscopic remission and showed long-term efficacy without adverse effects. However, the study could have included additional inflammatory markers for a more robust analysis (Banerjee et al., 2021). Similarly, the study conducted by Lang et al. (2015) in patients with mild to moderately active ulcerative colitis, using 5-aminosalicylic acid (5-ASA) at an optimized dose, demonstrated that curcumin supplementation (3 g/day) for 4 weeks improved clinical response and induced remission in patients with ulcerative colitis, but did not affect CRP and hemoglobin levels. The study lacked evaluation of other inflammatory markers and had a short intervention time (Lang et al., 2015).
Interesting results were also reported by Hanai et al. (2006), in their multicenter study that demonstrated that oral administration of curcumin (2 g/day) as an adjuvant to sulfasalazine/mesalamine (1–3 g/day) for 6 months promoted clinical and endoscopic improvement in ulcerative colitis patients in remission. However, the study did not include patients with mild to moderate disease activity (Hanai et al., 2006). Also used as adjuvant therapy for 8 weeks, Kedia et al. (2017), reported that oral use of curcumin (450 mg/day) as was not effective in inducing clinical and endoscopic remission in patients with mild to moderate ulcerative colitis, possibly due to the low dosage used and limitations in sample size (Kedia et al., 2017).
Lastly, Singla et al. (2014) evaluated for 8 weeks the effects of the standardized preparation of C. longa extract (NCB-02), by enema, associated with 5-ASA, in patients with mild to moderate ulcerative colitis. Treatment with NCB-02 was effective in inducing clinical remission and endoscopic improvement, but the study had significant losses during the research period (Singla et al., 2014).
In general, most randomized clinical trials have reported satisfactory and promising outcomes with the use of curcumin as an adjunctive therapy for IBD (Grammatikopoulou et al., 2018; Alves et al., 2022). However, the significant variability among studies, including variations in dosage, formulation type, route of administration, intervention duration, and limited sample sizes, restricts its applicability in clinical practice. Therefore, there is a need for standardization in clinical studies with respect to these variables to establish more effective therapeutic approaches.
It is evident, considering the information presented, that the biological characteristics of curcumin, particularly its effects on various therapeutic targets, underscore its potential as an adjunct therapy for IBD. This warrants discussion by medical societies in their guidelines to provide scientific guidance for healthcare professionals involved in the care of these patients. Notably, curcumin has demonstrated effects on multiple targets related to inflammation, oxidative stress, and the gut microbiota. For instance, it has been shown to modulate key pro-inflammatory cytokines such as TNF-α, IL-6, IL-10, and IL-1β, reducing the inflammatory burden in IBD patients. Additionally, curcumin exerts its antioxidant effects by modulating the activity of enzymes involved in oxidative stress, such as superoxide dismutase SOD and CAT. Moreover, curcumin has shown potential in modulating the gut microbiota composition, promoting a balanced microbial environment and influencing the gut-brain axis, as it can be visualized in the graphical abstract.
To comprehensively explore curcumin’s potential, future clinical trials should incorporate these mechanisms. Robust biomarkers can illuminate its intricate cellular pathways. By evaluating cytokine profiles, oxidative stress markers, and gut microbiota dynamics, researchers can elucidate curcumin’s therapeutic potential in IBD. This holistic approach enriches the foundation for evidence-based medical decisions.
However, prescribing curcumin demands caution. Factors like origin, dosage, and ongoing monitoring to detect early alterations, especially in hepatic markers should be considered. Such vigilance ensures patient safety and outcomes. Furthermore, as curcumin’s therapeutic potential evolves with new formulations, robust studies are imperative. Evaluating efficacy and safety of these novel formulations will expand our understanding and guide curcumin’s integration into medical practice.
Acknowledgement: None.
Funding Statement: The authors gratefully acknowledge the financial support of the CNPq (Conselho Nacional de Desenvolvimento Científico e Tecnológico) (435704/2018-4), INCT-Bioanalítica (Instituto Nacional de Ciências e Tecnologia em Bioanalítica) (465389/2014-7), CAPES/RENORBIO/PROAP (Coorde-nação de Aperfeiçoamento de Pessoal de Nível Superior), and FAPEAL/PPSUS (Fundação de Amparo à Pesquisa do Estado de Alagoas/Programa Pesquisa para o SUS) (60030-00879).
Author Contributions: Conceptualization, data curation and methodology, MOFG and FAM; investigation, MCA, ASPM, ORPA, MOFG and FAM; writing—original draft preparation ASPM, MCA, FOSC; writing—review and editing, MCA, ASPM, MOFG and FAM. All authors have read and agreed to the published version of the manuscript.
Availability of Data and Materials: The datasets generated during and/or analysed during the current study are available from the corresponding author on reasonable request.
Ethics Approval: Not applicable.
Conflicts of Interest: The authors declare that they have no conflicts of interest to report regarding the present study.
References
Afshariani R, Farhadi P, Ghaffarpasand F, Roozbeh J (2014). Effectiveness of topical curcumin for treatment of mastitis in breastfeeding women: A randomized, double-blind, placebo-controlled clinical trial. Oman Medical Journal 29: 330–334. https://doi.org/10.5001/omj.2014.89 [Google Scholar] [PubMed] [CrossRef]
Akaberi M, Sahebkar A, Emami SA (2021). Turmeric and curcumin: From traditional to modern medicine. Advances in Experimental Medicine and Biology 1291: 15–39. https://doi.org/10.1007/978-3-030-56153-6 [Google Scholar] [CrossRef]
Alemany-Cosme E, Saez-Gonzalez E, Moret I, Mateos B, Iborra M, Nos P, Sandoval J, Beltran B (2021). Oxidative stress in the pathogenesis of crohn’s disease and the interconnection with immunological response, microbiota, external environmental factors, and epigenetics. Antioxidants 10: 64. https://doi.org/10.3390/antiox10010064 [Google Scholar] [PubMed] [CrossRef]
Alizadeh F, Javadi M, Karami AA, Gholaminejad F, Kavianpour M, Haghighian HK (2018). Curcumin nanomicelle improves semen parameters, oxidative stress, inflammatory biomarkers, and reproductive hormones in infertile men: A randomized clinical trial. Phytotherapy Research 32: 514–521. https://doi.org/10.1002/ptr.5998 [Google Scholar] [PubMed] [CrossRef]
Altinel Y, Yalcin S, Ercan G, Yavuz E, Ercetin C, Gulcicek OB, Celik A, Ozkaya G, Uzun H (2020). The efficacy of curcumin on PDGF expression and NF-kappa B pathway: TNBS-induced colitis. Ulusal Travma ve Acil Cerrahi Dergisi 26: 663–670. https://doi.org/10.14744/tjtes.2019.45570 [Google Scholar] [PubMed] [CrossRef]
Alves MDC, Santos MO, Bueno NB, AraÚjo ORPd, Goulart MOF, Moura FA (2022). Efficacy of oral consumption of curcumin/for symptom improvement in inflammatory bowel disease: A systematic review of animal models and a meta-analysis of randomized clinical trials. BIOCELL 46: 2015–2047. https://doi.org/10.32604/biocell.2022.020407 [Google Scholar] [CrossRef]
Anand P, Kunnumakkara AB, Newman RA, Aggarwal BB (2007). Bioavailability of curcumin: Problems and promises. Molecular Pharmaceutics 4: 807–818. https://doi.org/10.1021/mp700113r [Google Scholar] [PubMed] [CrossRef]
Ansari MN, Rehman NU, Karim A, Soliman GA, Ganaie MA, Raish M, Hamad AM (2021). Role of oxidative stress and inflammatory cytokines (TNF-α and IL-6) in acetic acid-induced ulcerative colitis in rats: Ameliorated by Otostegia fruticosa. Life 11: 195. https://doi.org/10.3390/life11030195 [Google Scholar] [PubMed] [CrossRef]
Ashrafizadeh M, Rafiei H, Mohammadinejad R, Afshar EG, Farkhondeh T, Samarghandian S (2020). Potential therapeutic effects of curcumin mediated by JAK/STAT signaling pathway: A review. Phytotherapy Research 34: 1745–1760. https://doi.org/10.1002/ptr.6642 [Google Scholar] [PubMed] [CrossRef]
Balmus IM, Ciobica A, Trifan A, Stanciu C (2016). The implications of oxidative stress and antioxidant therapies in inflammatory bowel disease: Clinical aspects and animal models. Saudi Journal of Gastroenterology 22: 3–17. https://doi.org/10.4103/1319-3767.173753 [Google Scholar] [PubMed] [CrossRef]
Banerjee R, Pal P, Penmetsa A, Kathi P, Girish G, Goren I, Reddy DN (2021). Novel bioenhanced curcumin with mesalamine for induction of clinical and endoscopic remission in mild-to-moderate ulcerative colitis: A randomized double-blind placebo-controlled pilot study. Journal Clinical Gastroenterology 55: 702–708. https://doi.org/10.1097/MCG.0000000000001416 [Google Scholar] [PubMed] [CrossRef]
Bastaki SM, Al Ahmed MM, Al Zaabi A, Amir N, Adeghate E (2016). Effect of turmeric on colon histology, body weight, ulcer, IL-23, MPO and glutathione in acetic-acid-induced inflammatory bowel disease in rats. BMC Complementary and Alternative Medicine 16: 72. https://doi.org/10.1186/s12906-016-1057-5 [Google Scholar] [PubMed] [CrossRef]
Beaugerie L, Itzkowitz SH (2015). Cancers complicating inflammatory bowel disease. New England Journal of Medicine 372: 1441–1452. https://doi.org/10.1056/NEJMra1403718 [Google Scholar] [PubMed] [CrossRef]
Bourgonje AR, von Martels JZH, de Vos P, Faber KN, Dijkstra G (2018). Increased fecal calprotectin levels in Crohn’s disease correlate with elevated serum Th1- and Th17-associated cytokines. PLoS One 13: e0193202. https://doi.org/10.1371/journal.pone.0193202 [Google Scholar] [PubMed] [CrossRef]
Brunet E, Roig-Ramos C, Vela E, Clèries M, Melcarne L, Villòria A, Pontes C, Calvet X (2018). Prevalence, incidence and mortality of inflammatory bowel disease in Catalonia. A population-based analysis. Annals of Medicine 50: 613–619. https://doi.org/10.1080/07853890.2018.1523550 [Google Scholar] [PubMed] [CrossRef]
Bucchini L (2019). The 2019 curcumin crisis in Italy: What we know so far, and early lessons. Planta Medica 85: 1401–0. https://doi.org/10.1055/s-0039-3399667 [Google Scholar] [CrossRef]
Bumrungpert A, Somboonpanyakul P, Pavadhgul P, Thaninthranon S (2018). Effects of fenugreek, ginger, and turmeric supplementation on human milk volume and nutrient content in breastfeeding mothers: A randomized double-blind controlled trial. Breastfeeding Medicine 13: 645–650. https://doi.org/10.1089/bfm.2018.0159 [Google Scholar] [PubMed] [CrossRef]
Burge K, Gunasekaran A, Eckert J, Chaaban H (2019). Curcumin and intestinal inflammatory diseases: Molecular mechanisms of protection. International Journal of Molecular Science 20: 1912. https://doi.org/10.3390/ijms20081912 [Google Scholar] [PubMed] [CrossRef]
Camacho-Barquero L, Villegas I, Sanchez-Calvo JM, Talero E, Sanchez-Fidalgo S, Motilva V, Alarcon de la Lastra C (2007). Curcumin, a Curcuma longa constituent, acts on MAPK p38 pathway modulating COX-2 and iNOS expression in chronic experimental colitis. International Immunopharmacology 7: 333–342. https://doi.org/10.1016/j.intimp.2006.11.006 [Google Scholar] [PubMed] [CrossRef]
Chami B, Martin NJJ, Dennis JM, Witting PK (2018). Myeloperoxidase in the inflamed colon: A novel target for treating inflammatory bowel disease. Archives of Biochemistry and Biophysics 645: 61–71. https://doi.org/10.1016/j.abb.2018.03.012 [Google Scholar] [PubMed] [CrossRef]
Chavalittumrong P, Chivapat S, Punyamong S, Chuthaputti A, Phisalaphong C (2002). Chronic toxicity study of curcuminoids in rats. Songklanakarin Journal Science and Technollogy 24: 633–647. [Google Scholar]
Chen Y, Lu Y, Lee RJ, Xiang G (2020). Nano encapsulated curcumin: And its potential for biomedical applications. International Journal of Nanomedicine 15: 3099–3120. https://doi.org/10.2147/IJN.S210320 [Google Scholar] [PubMed] [CrossRef]
Cheng AL, Hsu CH, Lin JK, Hsu MM, Ho YF et al. (2001). Phase I clinical trial of curcumin, a chemopreventive agent, in patients with high-risk or pre-malignant lesions. Anticancer Research 21: 2895–2900. [Google Scholar] [PubMed]
Cheng D, Li W, Wang L, Lin T, Poiani G, Wassef A, Hudlikar R, Ondar PL, Brunetti L, Kong AN (2019). Pharmacokinetics, pharmacodynamics, and PKPD modeling of curcumin in regulating antioxidant and epigenetic gene expression in healthy human volunteers. Molecular Pharmacology 16: 1881–1889. https://doi.org/10.1021/acs.molpharmaceut.8b01246 [Google Scholar] [PubMed] [CrossRef]
Cong Y, Wang L, Konrad A, Schoeb T, Elson CO (2009). Curcumin induces the tolerogenic dendritic cell that promotes differentiation of intestine-protective regulatory T cells. European Journal of Immunology 39: 3134–3146. https://doi.org/10.1002/eji.200939052 [Google Scholar] [PubMed] [CrossRef]
Cooney JM, Barnett MP, Dommels YE, Brewster D, Butts CA, McNabb WC, Laing WA, Roy NC (2016). A combined omics approach to evaluate the effects of dietary curcumin on colon inflammation in the Mdr1a-/- mouse model of inflammatory bowel disease. The Journal of Nutritional Biochemistry 27: 181–192. https://doi.org/10.1016/j.jnutbio.2015.08.030 [Google Scholar] [PubMed] [CrossRef]
Costantino M, Corno C, Colombo D, Perego P (2022). Curcumin and related compounds in cancer cells: New avenues for old molecules. Frontiers in Pharmacology 13: 889816. https://doi.org/10.3389/fphar.2022.889816 [Google Scholar] [PubMed] [CrossRef]
Cukovic-Cavka S, Vucelic B, Urek MC, Brinar M, Turk N (2013). The role of anti-TNF therapy in ulcerative colitis. Acta Medica Croatica 67: 171–177. [Google Scholar] [PubMed]
Cunha Neto F, Marton LT, de Marqui SV, Lima TA, Barbalho SM (2019). Curcuminoids from Curcuma longa: New adjuvants for the treatment of crohn’s disease and ulcerative colitis? Critical Reviews in Food Science and Nutrition 59: 2136–2143. https://doi.org/10.1080/10408398.2018.1456403 [Google Scholar] [PubMed] [CrossRef]
Deguchi Y, Andoh A, Inatomi O, Yagi Y, Bamba S, Araki Y, Hata K, Tsujikawa T, Fujiyama Y (2007). Curcumin prevents the development of dextran sulfate Sodium (DSS)-induced experimental colitis. Digestive Diseases and Sciences 52: 2993–2998. https://doi.org/10.1007/s10620-006-9138-9 [Google Scholar] [PubMed] [CrossRef]
Dei Cas M, Ghidoni R (2019). Dietary Curcumin: Correlation between bioavailability and health potential. Nutrients 11: 2147. https://doi.org/10.3390/nu11092147 [Google Scholar] [PubMed] [CrossRef]
Dhillon N, Aggarwal BB, Newman RA, Wolff RA, Kunnumakkara AB, Abbruzzese JL, Ng CS, Badmaev V, Kurzrock R (2008). Phase II trial of curcumin in patients with advanced pancreatic cancer. Clinical Cancer Research 14: 4491–4499. https://doi.org/10.1158/1078-0432.CCR-08-0024 [Google Scholar] [PubMed] [CrossRef]
Dudzinska E, Gryzinska M, Ognik K, Gil-Kulik P, Kocki J (2018). Oxidative stress and effect of treatment on the oxidation product decomposition processes in IBD. Oxidative Mededicine and Cellular Longevity 2018: 7918261. https://doi.org/10.1155/2018/7918261 [Google Scholar] [PubMed] [CrossRef]
Duff W, Haskey N, Potter G, Alcorn J, Hunter P, Fowler S (2018). Non-pharmacological therapies for inflammatory bowel disease: Recommendations for self-care and physician guidance. World Journal of Gastroenterology 24: 3055–3070. https://doi.org/10.3748/wjg.v24.i28.3055 [Google Scholar] [PubMed] [CrossRef]
Edwards RL, Luis PB, Varuzza PV, Joseph AI, Presley SH, Chaturvedi R, Schneider C (2017). The anti-inflammatory activity of curcumin is mediated by its oxidative metabolites. Journal of Biological Chemistry 292: 21243–21252. https://doi.org/10.1074/jbc.RA117.000123 [Google Scholar] [PubMed] [CrossRef]
Epelbaum R, Schaffer M, Vizel B, Badmaev V, Bar-Sela G (2010). Curcumin and gemcitabine in patients with advanced pancreatic cancer. Nutriton and Cancer 62: 1137–1141. https://doi.org/10.1080/01635581.2010.513802 [Google Scholar] [PubMed] [CrossRef]
Fallahi F, Borran S, Ashrafizadeh M, Zarrabi A, Pourhanifeh MH, Khaksary Mahabady M, Sahebkar A, Mirzaei H (2021). Curcumin and inflammatory bowel diseases: From in vitro studies to clinical trials. Molecular Immunology 130: 20–30. https://doi.org/10.1016/j.molimm.2020.11.016 [Google Scholar] [PubMed] [CrossRef]
Filardi T, Vari R, Ferretti E, Zicari A, Morano S, Santangelo C (2020). Curcumin: Could this compound be useful in pregnancy and pregnancy-related complications? Nutrients 12: 3179. https://doi.org/10.3390/nu12103179 [Google Scholar] [PubMed] [CrossRef]
Friedrich M, Pohin M, Powrie F (2019). Cytokine networks in the pathophysiology of inflammatory bowel disease. Immunity 50: 992–1006. https://doi.org/10.1016/j.immuni.2019.03.017 [Google Scholar] [PubMed] [CrossRef]
Funk JL, Frye JB, Oyarzo JN, Zhang H, Timmermann BN (2010). Anti-arthritic effects and toxicity of the essential oils of turmeric (Curcuma longa L.). Journal of Agricultural and Food Chemistry 58: 842–849. https://doi.org/10.1021/jf9027206 [Google Scholar] [PubMed] [CrossRef]
Garcea G, Berry DP, Jones DJ, Singh R, Dennison AR, Farmer PB, Sharma RA, Steward WP, Gescher AJ (2005). Consumption of the putative chemopreventive agent curcumin by cancer patients: Assessment of curcumin levels in the colorectum and their pharmacodynamic consequences. Cancer, Epidemiol Biomarkers & Prevention 14: 120–125. [Google Scholar]
Garcia-Nino WR, Pedraza-Chaverri J (2014). Protective effect of curcumin against heavy metals-induced liver damage. Food and Chemical Toxicology 69: 182–201. https://doi.org/10.1016/j.fct.2014.04.016 [Google Scholar] [PubMed] [CrossRef]
Ghaneifar Z, Yousefi Z, Tajik F, Nikfar B, Ghalibafan F, Abdollahi E, Momtazi-Borojeni AA (2020). The potential therapeutic effects of curcumin on pregnancy complications: Novel insights into reproductive medicine. IUBMB Life 72: 2572–2583. https://doi.org/10.1002/iub.2399 [Google Scholar] [PubMed] [CrossRef]
Girme A, Saste G, Balasubramaniam AK, Pawar S, Ghule C, Hingorani L (2020). Assessment of Curcuma longa extract for adulteration with synthetic curcumin by analytical investigations. Journal of Pharmaceutical and Biomedical Analysis 191: 113603. https://doi.org/10.1016/j.jpba.2020.113603 [Google Scholar] [PubMed] [CrossRef]
Gong Z, Zhao S, Zhou J, Yan J, Wang L, Du X, Li H, Chen Y, Cai W, Wu J (2018). Curcumin alleviates DSS-induced colitis via inhibiting NLRP3 inflammsome activation and IL-1β production. Molecullar Immunology 104: 11–19. https://doi.org/10.1016/j.molimm.2018.09.004 [Google Scholar] [PubMed] [CrossRef]
Gopu B, Dileep R, Rani MU, Kumar CS, Kumar MV, Reddy AG (2015). Protective role of curcumin and flunixin against acetic acid-induced inflammatory bowel disease via modulating inflammatory mediators and cytokine profile in rats. Journal of Environmental Pathology, Toxicology and Oncology 34: 309–320. https://doi.org/10.1615/JEnvironPatholToxicolOncol.2015013049 [Google Scholar] [PubMed] [CrossRef]
Goulart RA, Barbalho SM, Lima VM, Souza GA, Matias JN et al. (2021). Effects of the use of curcumin on ulcerative colitis and crohn’s disease: A systematic review. Journal of Medicinal Food 24: 675–685. https://doi.org/10.1089/jmf.2020.0129 [Google Scholar] [PubMed] [CrossRef]
Grammatikopoulou MG, Gkiouras K, Theodoridis X, Asteriou E, Forbes A, Bogdanos DP (2018). Oral adjuvant curcumin therapy for attaining clinical remission in ulcerative colitis: A systematic review and meta-analysis of randomized controlled trials. Nutrients 10: 1737. https://doi.org/10.3390/nu10111737 [Google Scholar] [PubMed] [CrossRef]
Guan Q (2019). A comprehensive review and update on the pathogenesis of inflammatory bowel disease. Journal of Immunology Research 2019: 7247238. https://doi.org/10.1155/2019/7247238 [Google Scholar] [PubMed] [CrossRef]
Hanai H, Iida T, Takeuchi K, Watanabe F, Maruyama Y et al. (2006). Curcumin maintenance therapy for ulcerative colitis: Randomized, multicenter, double-blind, placebo-controlled trial. Clinical Gastroenterology and Hepatology 4: 1502–1506. https://doi.org/10.1016/j.cgh.2006.08.008 [Google Scholar] [PubMed] [CrossRef]
Hasanzadeh S, Read MI, Bland AR, Majeed M, Jamialahmadi T, Sahebkar A (2020). Curcumin: An inflammasome silencer. Pharmacolological Research 159: 104921. https://doi.org/10.1016/j.phrs.2020.104921 [Google Scholar] [PubMed] [CrossRef]
Hassaninasab A, Hashimoto Y, Tomita-Yokotani K, Kobayashi M (2011). Discovery of the curcumin metabolic pathway involving a unique enzyme in an intestinal microorganism. Proceedings of the National Academy of Sciences of the United States of America 108: 6615–6620. https://doi.org/10.1073/pnas.1016217108 [Google Scholar] [PubMed] [CrossRef]
Heger M, van Golen RF, Broekgaarden M, Michel MC (2014). The molecular basis for the pharmacokinetics and pharmacodynamics of curcumin and its metabolites in relation to cancer. Pharmacological Reviews 66: 222–307. https://doi.org/10.1124/pr.110.004044 [Google Scholar] [PubMed] [CrossRef]
Hewlings SJ, Kalman DS (2017). Curcumin: A review of its effects on human health. Foods 6: 92. https://doi.org/10.3390/foods6100092 [Google Scholar] [PubMed] [CrossRef]
Hussain Y, Abdullah, Khan F, Alsharif KF, Alzahrani KJ, Saso L, Khan H (2022). Regulatory effects of curcumin on platelets: An update and future directions. Biomedicines 10: 3180. https://doi.org/10.3390/biomedicines10123180 [Google Scholar] [PubMed] [CrossRef]
Hvas CL, Bendix M, Dige A, Dahlerup JF, Agnholt J (2018). Current, experimental, and future treatments in inflammatory bowel disease: A clinical review. Immunopharmacology and Immunotoxicology 40: 446–460. https://doi.org/10.1080/08923973.2018.1469144 [Google Scholar] [PubMed] [CrossRef]
IP WKE, Hoshi N, Shouval DS, Snapper S, Medzhitov R (2017). Anti-inflammatory effect of IL-10 mediated by metabolic reprogramming of macrophages. Science 356: 513–519. https://doi.org/10.1126/science.aal3535 [Google Scholar] [PubMed] [CrossRef]
Ipar VS, Dsouza A, Devarajan PV (2019). Enhancing curcumin oral bioavailability through nanoformulations. European Journal of Drug Metabolism and Pharmacokinetics 44: 459–480. https://doi.org/10.1007/s13318-019-00545-z [Google Scholar] [PubMed] [CrossRef]
Irving GR, Howells LM, Sale S, Kralj-Hans I, Atkin WS et al. (2013). Prolonged biologically active colonic tissue levels of curcumin achieved after oral administration—A clinical pilot study including assessment of patient acceptability. Cancer Prevention Research 6: 119–128. https://doi.org/10.1158/1940-6207.CAPR-12-0281 [Google Scholar] [PubMed] [CrossRef]
Jabczyk M, Nowak J, Hudzik B, Zubelewicz-Szkodzinska B (2021). Curcumin and its potential impact on microbiota. Nutrients 13: 2004. https://doi.org/10.3390/nu13062004 [Google Scholar] [PubMed] [CrossRef]
Jian YT, Mai GF, Wang JD, Zhang YL, Luo RC, Fang YX (2005). Preventive and therapeutic effects of NF-kappaB inhibitor curcumin in rats colitis induced by trinitrobenzene sulfonic acid. World Journal of Gastroenterology 11: 1747–1752. https://doi.org/10.3748/wjg.v11.i12.1747 [Google Scholar] [PubMed] [CrossRef]
Jiang H, Deng CS, Zhang M, Xia J (2006). Curcumin-attenuated trinitrobenzene sulphonic acid induces chronic colitis by inhibiting expression of cyclooxygenase-2. World Journal of Gastroenterology 12: 3848–3853. https://doi.org/10.3748/wjg.v12.i24.3848 [Google Scholar] [PubMed] [CrossRef]
Joshi J, Ghaisas S, Vaidya A, Vaidya R, Kamat DV, Bhagwat AN (2003). Early human safety study of turmeric oil (Curcuma longa oil) administered orally in healthy volunteers. Journal of the Association of Physicians of India 51: 1055–1060. [Google Scholar] [PubMed]
Jutooru I, Chadalapaka G, Lei P, Safe S (2010). Inhibition of NFκB and pancreatic cancer cell and tumor growth by curcumin is dependent on specificity protein down-regulation. Journal of Biological Chemistry 285: 25332–25344. https://doi.org/10.1074/jbc.M109.095240 [Google Scholar] [PubMed] [CrossRef]
Kamalian A, Sohrabi Asl M, Dolatshahi M, Afshari K, Shamshiri S, Momeni Roudsari N, Momtaz S, Rahimi R, Abdollahi M, Abdolghaffari AH (2020). Interventions of natural and synthetic agents in inflammatory bowel disease, modulation of nitric oxide pathways. World Journal of Gastroenterology 26: 3365–3400. https://doi.org/10.3748/wjg.v26.i24.3365 [Google Scholar] [PubMed] [CrossRef]
Kaplan GG, Bernstein CN, Coward S, Bitton A, Murthy SK, Nguyen GC, Lee K, Cooke-Lauder J, Benchimol EI (2019). The impact of inflammatory bowel disease in Canada 2018: Epidemiology. Journal of the Canadian Association of Gastroenterology 2: S6–S16. https://doi.org/10.1093/jcag/gwy054 [Google Scholar] [PubMed] [CrossRef]
Karthikeyan A, Young KN, Moniruzzaman M, Beyene AM, Do K, Kalaiselvi S, Min T (2021). Curcumin and its modified formulations on inflammatory bowel disease (IBDThe story so far and future outlook. Pharmaceutics 13: 484. https://doi.org/10.3390/pharmaceutics13040484 [Google Scholar] [PubMed] [CrossRef]
Kedia S, Bhatia V, Thareja S, Garg S, Mouli VP, Bopanna S, Tiwari V, Makharia G, Ahuja V (2017). Low dose oral curcumin is not effective in induction of remission in mild to moderate ulcerative colitis: Results from a randomized double blind placebo controlled trial. World Journal of Gastrointestinal Pharmacology and Therapeutics 8: 147–154. https://doi.org/10.4292/wjgpt.v8.i2.147 [Google Scholar] [PubMed] [CrossRef]
Kesharwani SS, Ahmad R, Bakkari MA, Rajput MKS, Dachineni RC, Valiveti K, Kapur S, Jayarama Bhat G, Singh AB, Tummala H (2018). Site-directed non-covalent polymer-drug complexes for inflammatory bowel disease (IBDFormulation development, characterization and pharmacological evaluation. Journal of Controlled Release 290: 165–179. https://doi.org/10.1016/j.jconrel.2018.08.004 [Google Scholar] [PubMed] [CrossRef]
Kobayashi K, Tsugami Y, Suzuki N, Suzuki T, Nishimura T (2021). Suppressive effects of curcumin on milk production without inflammatory responses in lactating mammary epithelial cells. Phytomedicine 80: 153360. https://doi.org/10.1016/j.phymed.2020.153360 [Google Scholar] [PubMed] [CrossRef]
Kocaadam B, Sanlier N (2017). Curcumin, an active component of turmeric (Curcuma longaand its effects on health. Critical Reviews in Food Science and Nutrition 57: 2889–2895. https://doi.org/10.1080/10408398.2015.1077195 [Google Scholar] [PubMed] [CrossRef]
Kotha RR, Luthria DL (2019). Curcumin: Biological, pharmaceutical, nutraceutical, and analytical aspects. Molecules 24: 2930. https://doi.org/10.3390/molecules24162930 [Google Scholar] [PubMed] [CrossRef]
Kunnumakkara AB, Bordoloi D, Padmavathi G, Monisha J, Roy NK, Prasad S, Aggarwal BB (2017). Curcumin, the golden nutraceutical: Multitargeting for multiple chronic diseases. British Journal of Pharmacology 174: 1325–1348. https://doi.org/10.1111/bph.13621 [Google Scholar] [PubMed] [CrossRef]
Lang A, Salomon N, Wu JC, Kopylov U, Lahat A et al. (2015). Curcumin in combination with mesalamine induces remission in patients with mild-to-moderate ulcerative colitis in a randomized controlled trial. Clinical Gastroenterology and Hepatology 13: 1444–1449. E.1. https://doi.org/10.1016/j.cgh.2015.02.019 [Google Scholar] [PubMed] [CrossRef]
Lao CD, Ruffin MTT, Normolle D, Heath DD, Murray SI, Bailey JM, Boggs ME, Crowell J, Rock CL, Brenner DE (2006). Dose escalation of a curcuminoid formulation. BMC Complementary Medicine and Therapies 6: 10. https://doi.org/10.1186/1472-6882-6-10 [Google Scholar] [PubMed] [CrossRef]
Lee SJ, Chandrasekran P, Mazucanti CH, O’Connell JF, Egan JM, Kim Y (2022). Dietary curcumin restores insulin homeostasis in diet-induced obese aged mice. Aging 14: 225–239. https://doi.org/10.18632/aging.203821 [Google Scholar] [PubMed] [CrossRef]
Lee M, Chang EB (2021). Inflammatory bowel diseases (IBD) and the microbiome-searching the crime scene for clues. Gastroenterology 160: 524–537. https://doi.org/10.1053/j.gastro.2020.09.056 [Google Scholar] [PubMed] [CrossRef]
Li P, Chang M (2021). Roles of PRR-mediated signaling pathways in the regulation of oxidative stress and inflammatory diseases. International Journal of Molecular Sciences 22: 7688. https://doi.org/10.3390/ijms22147688 [Google Scholar] [PubMed] [CrossRef]
Li CP, Li JH, He SY, Chen O, Shi L (2015). Effect of curcumin on p38MAPK expression in DSS-induced murine ulcerative colitis. Genetics and Molecular Research 14: 3450–3458. https://doi.org/10.4238/2015.April.15.8 [Google Scholar] [PubMed] [CrossRef]
Li Q, Zhai W, Jiang Q, Huang R, Liu L, Dai J, Gong W, Du S, Wu Q (2015). Curcumin-piperine mixtures in self-microemulsifying drug delivery system for ulcerative colitis therapy. International Journal of Pharmaceutics 490: 22–31. https://doi.org/10.1016/j.ijpharm.2015.05.008 [Google Scholar] [PubMed] [CrossRef]
Li Y, Zhang J, Ma D, Zhang L, Si M, Yin H, Li J (2012). Curcumin inhibits proliferation and invasion of osteosarcoma cells through inactivation of Notch-1 signaling. The FEBS Journal 279: 2247–2259. https://doi.org/10.1111/j.1742-4658.2012.08607.x [Google Scholar] [PubMed] [CrossRef]
Lim WC, Hanauer SB (2004). Emerging biologic therapies in inflammatory bowel disease. Reviews in Gastroenterological Disorders 4: 66–85. [Google Scholar] [PubMed]
Lin X, Bai D, Wei Z, Zhang Y, Huang Y, Deng H, Huang X (2019). Curcumin attenuates oxidative stress in RAW264.7 cells by increasing the activity of antioxidant enzymes and activating the Nrf2-Keap1 pathway. PLoS One 14: e0216711. https://doi.org/10.1371/journal.pone.0216711 [Google Scholar] [PubMed] [CrossRef]
Liu L, Liu YL, Liu GX, Chen X, Yang K, Yang YX, Xie Q, Gan HK, Huang XL, Gan HT (2013). Curcumin ameliorates dextran sulfate sodium-induced experimental colitis by blocking STAT3 signaling pathway. International Immunopharmacology 17: 314–320. https://doi.org/10.1016/j.intimp.2013.06.020 [Google Scholar] [PubMed] [CrossRef]
Liu W, Zhai Y, Heng X, Che FY, Chen W, Sun D, Zhai G (2016). Oral bioavailability of curcumin: Problems and advancements. Journal of Drug Targeting 24: 694–702. https://doi.org/10.3109/1061186X.2016.1157883 [Google Scholar] [PubMed] [CrossRef]
Lopresti AL, Smith SJ, Rea A, Michel S (2021). Efficacy of a curcumin extract (Curcugen) on gastrointestinal symptoms and intestinal microbiota in adults with self-reported digestive complaints: A randomised, double-blind, placebo-controlled study. BMC Complementary Medicine and Therapies 21: 40. https://doi.org/10.1186/s12906-021-03220-6 [Google Scholar] [PubMed] [CrossRef]
Lubbad A, Oriowo MA, Khan I (2009). Curcumin attenuates inflammation through inhibition of TLR-4 receptor in experimental colitis. Molecular and Cellular Biochemistry 322: 127–135. https://doi.org/10.1007/s11010-008-9949-4 [Google Scholar] [PubMed] [CrossRef]
Lucas M, Freitas M, Xavier JA, Moura FA, Goulart MOF, Ribeiro D, Fernandes E (2021). The scavenging effect of curcumin, piperine and their combination against physiological relevant reactive pro-oxidant species using in vitro non-cellular and cellular models. Chemical Papers 75: 5269–5277. https://doi.org/10.1007/s11696-021-01710-y [Google Scholar] [CrossRef]
Lukefahr AL, McEvoy S, Alfafara C, Funk JL (2018). Drug-induced autoimmune hepatitis associated with turmeric dietary supplement use. BMJ Case Reports 2018. https://doi.org/10.1136/bcr-2018-224611 [Google Scholar] [PubMed] [CrossRef]
Mahdipour M, Shafaghi A, Mansour-Ghanaei F, Hojati A, Joukar F, Mavaddati S (2019). Fecal calprotectin role in diagnosis of ulcerative colitis and treatment follow-up. Journal of Coloproctology 39: 115–120. https://doi.org/10.1016/j.jcol.2018.10.012 [Google Scholar] [CrossRef]
Mao L, Kitani A, Strober W, Fuss IJ (2018). The role of NLRP3 and IL-1beta in the pathogenesis of inflammatory bowel disease. Frontiers in Immunology 9: 2566. https://doi.org/10.3389/fimmu.2018.02566 [Google Scholar] [PubMed] [CrossRef]
Martins ASDP, Campos SBG, Goulart MOF, Moura FA (2021). Extraintestinal manifestations of inflammatory bowel disease, nitroxidative stress and dysbiosis: What is the link between them? BIOCELL 45: 461–481. https://doi.org/10.32604/biocell.2021.014332 [Google Scholar] [CrossRef]
Marton LT, Pescinini ESLM, Camargo MEC, Barbalho SM, Haber J, Sinatora RV, Detregiachi CRP, Girio RJS, Buchaim DV, Bueno PCS (2021). The effects of curcumin on diabetes mellitus: A systematic review. Frontiers in Endocrinology 12: 669448. https://doi.org/10.3389/fendo.2021.669448 [Google Scholar] [PubMed] [CrossRef]
Masoodi M, Mahdiabadi MA, Mokhtare M, Agah S, Kashani AHF, Rezadoost AM, Sabzikarian M, Talebi A, Sahebkar A (2018). The efficacy of curcuminoids in improvement of ulcerative colitis symptoms and patients’ self-reported well-being: A randomized double-blind controlled trial. Journal of Cellular Biochemistry 119: 9552–9559. https://doi.org/10.1002/jcb.27273 [Google Scholar] [PubMed] [CrossRef]
Mazieiro R, Frizon RR, Barbalho SM, Goulart RA (2018). Is curcumin a possibility to treat inflammatory bowel diseases? Journal of Medicinal Food 21: 1077–1085. https://doi.org/10.1089/jmf.2017.0146 [Google Scholar] [PubMed] [CrossRef]
McFadden RM, Larmonier CB, Shehab KW, Midura-Kiela M, Ramalingam R et al. (2015). The role of curcumin in modulating colonic microbiota during colitis and colon cancer prevention. Inflammatory Bowel Diseases 21: 2483–2494. https://doi.org/10.1097/MIB.0000000000000522 [Google Scholar] [PubMed] [CrossRef]
Mentella MC, Scaldaferri F, Pizzoferrato M, Gasbarrini A, Miggiano GAD (2020). Nutrition, IBD and gut microbiota: A review. Nutrients 12: 944. https://doi.org/10.3390/nu12040944 [Google Scholar] [PubMed] [CrossRef]
Mijan MA, Lim BO (2018). Diets, functional foods, and nutraceuticals as alternative therapies for inflammatory bowel disease: Present status and future trends. World Journal of Gastroenterology 24: 2673–2685. https://doi.org/10.3748/wjg.v24.i25.2673 [Google Scholar] [PubMed] [CrossRef]
Moura FA, de Andrade KQ, dos Santos JC, Araujo OR, Goulart MO (2015). Antioxidant therapy for treatment of inflammatory bowel disease: Does it work? Redox Biology 6: 617–639. https://doi.org/10.1016/j.redox.2015.10.006 [Google Scholar] [PubMed] [CrossRef]
Moura FA, Goulart MOF, Campos SBG, da Paz Martins AS (2020). The close interplay of nitro-oxidative stress, advanced glycation end products and inflammation in inflammatory bowel diseases. Current Medicinal Chemistry 27: 2059–2076. https://doi.org/10.2174/0929867325666180904115633 [Google Scholar] [PubMed] [CrossRef]
Mouzaoui S, Banerjee S, Djerdjouri B (2020). Low-dose curcumin reduced TNBS-associated mucin depleted foci in mice by scavenging superoxide anion and lipid peroxides, rebalancing matrix NO synthase and aconitase activities, and recoupling mitochondria. Inflammopharmacology 28: 949–965. https://doi.org/10.1007/s10787-019-00684-4 [Google Scholar] [PubMed] [CrossRef]
Mouzaoui S, Rahim I, Djerdjouri B (2012). Aminoguanidine and curcumin attenuated tumor necrosis factor (TNF)-α-induced oxidative stress, colitis and hepatotoxicity in mice. International Immunopharmacology 12: 302–311. https://doi.org/10.1016/j.intimp.2011.10.010 [Google Scholar] [PubMed] [CrossRef]
Mudter J, Neurath MF (2007). IL-6 signaling in inflammatory bowel disease: Pathophysiological role and clinical relevance. Inflammatory Bowel Diseases 13: 1016–1023. https://doi.org/10.1002/ibd.20148 [Google Scholar] [PubMed] [CrossRef]
Nelson KM, Dahlin JL, Bisson J, Graham J, Pauli GF, Walters MA (2017). The essential medicinal chemistry of curcumin. Journal of Medicinal Chemistry 60: 1620–1637. https://doi.org/10.1021/acs.jmedchem.6b00975 [Google Scholar] [PubMed] [CrossRef]
Ni J, Wu GD, Albenberg L, Tomov VT (2017). Gut microbiota and IBD: Causation or correlation? Nature Reviews Gastroenterology & Hepatology 14: 573–584. https://doi.org/10.1038/nrgastro.2017.88 [Google Scholar] [PubMed] [CrossRef]
Niu X, Wu T, Li G, Gu X, Tian Y, Cui H (2022). Insights into the critical role of the PXR in preventing carcinogenesis and chemotherapeutic drug resistance. International Journal of Biological Sciences 18: 742–759. https://doi.org/10.7150/ijbs.68724 [Google Scholar] [PubMed] [CrossRef]
Ohno M, Nishida A, Sugitani Y, Nishino K, Inatomi O, Sugimoto M, Kawahara M, Andoh A (2017). Nanoparticle curcumin ameliorates experimental colitis via modulation of gut microbiota and induction of regulatory T cells. PLoS One 12: e0185999. https://doi.org/10.1371/journal.pone.0185999 [Google Scholar] [PubMed] [CrossRef]
Panahi Y, Ghanei M, Hajhashemi A, Sahebkar A (2016). Effects of curcuminoids-piperine combination on systemic oxidative stress, clinical symptoms and quality of life in subjects with chronic pulmonary complications due to sulfur mustard: A randomized controlled trial. Journal of Dietary Supplements 13: 93–105. https://doi.org/10.3109/19390211.2014.952865 [Google Scholar] [PubMed] [CrossRef]
Panahi Y, Hosseini MS, Khalili N, Naimi E, Majeed M, Sahebkar A (2015). Antioxidant and anti-inflammatory effects of curcuminoid-piperine combination in subjects with metabolic syndrome: A randomized controlled trial and an updated meta-analysis. Clinical Nutrition 34: 1101–1108. https://doi.org/10.1016/j.clnu.2014.12.019 [Google Scholar] [PubMed] [CrossRef]
Panahi Y, Khalili N, Sahebi E, Namazi S, Simental-Mendia LE, Majeed M, Sahebkar A (2018). Effects of curcuminoids plus piperine on glycemic, hepatic and inflammatory biomarkers in patients with type 2 diabetes mellitus: A randomized double-blind placebo-controlled trial. Drug Research 68: 403–409. https://doi.org/10.1055/s-0044-101752 [Google Scholar] [PubMed] [CrossRef]
Pancholi V, Smina TP, Kunnumakkara AB, Maliakel B, Krishnakumar IM (2021). Safety assessment of a highly bioavailable curcumin-galactomannoside complex (CurQfen) in healthy volunteers, with a special reference to the recent hepatotoxic reports of curcumin supplements: A 90-days prospective study. Toxicology Reports 8: 1255–1264. https://doi.org/10.1016/j.toxrep.2021.06.008 [Google Scholar] [PubMed] [CrossRef]
Pasternak BA, Mello SD, Jurickova HanII, Willson X, Flick T et al. (2011). Lipopolysaccharide exposure is linked to activation of the acute phase response and growth failure in pediatric crohn’s disease and murine colitis. Inflammatory Bowel Diseases 16: 856–869. https://doi.org/10.1002/ibd.21132 [Google Scholar] [PubMed] [CrossRef]
Patel SS, Acharya A, Ray RS, Agrawal R, Raghuwanshi R, Jain P (2020). Cellular and molecular mechanisms of curcumin in prevention and treatment of disease. Critical Reviews in Food Science and Nutrition 60: 887–939. https://doi.org/10.1080/10408398.2018.1552244 [Google Scholar] [PubMed] [CrossRef]
Peng Y, Ao M, Dong B, Jiang Y, Yu L, Chen Z, Hu C, Xu R (2021). Anti-inflammatory effects of curcumin in the inflammatory diseases: Status, limitations and countermeasures. Drug Design, Development and Therapy 15: 4503–4525. https://doi.org/10.2147/DDDT.S327378 [Google Scholar] [PubMed] [CrossRef]
Peterson CT, Vaughn AR, Sharma V, Chopra D, Mills PJ, Peterson SN, Sivamani RK (2018). Effects of turmeric and curcumin dietary supplementation on human gut microbiota: A double-blind, randomized, placebo-controlled pilot study. Journal of Evidence-Based Integrative Medicine 23: 2515690X1879072. https://doi.org/10.1177/2515690X18790725 [Google Scholar] [PubMed] [CrossRef]
Piechota-Polanczyk A, Fichna J (2014). Review article: The role of oxidative stress in pathogenesis and treatment of inflammatory bowel diseases. Naunyn-Schmiedeberg’s Archives of Pharmacology 387: 605–620. https://doi.org/10.1007/s00210-014-0985-1 [Google Scholar] [PubMed] [CrossRef]
Prasad S, Tyagi AK, Aggarwal BB (2014). Recent developments in delivery, bioavailability, absorption and metabolism of curcumin: The golden pigment from golden spice. Cancer Research and Treatment 46: 2–18. https://doi.org/10.4143/crt.2014.46.1.2 [Google Scholar] [PubMed] [CrossRef]
Qiu P, Man S, Li J, Liu J, Zhang L, Yu P, Gao W (2016). Overdose intake of curcumin initiates the unbalanced state of bodies. Journal of Agricultural and Food Chemistry 64: 2765–2771. https://doi.org/10.1021/acs.jafc.6b00053 [Google Scholar] [PubMed] [CrossRef]
Quaglio AEV, Grillo TG, de Oliveira ECS, Di Stasi LC, Sassaki LY (2022). Gut microbiota, inflammatory bowel disease and colorectal cancer. World Journal of Gastroenterology 28: 4053–4060. https://doi.org/10.3748/wjg.v28.i30.4053 [Google Scholar] [PubMed] [CrossRef]
Rukoyatkina N, Shpakova V, Sudnitsyna J, Panteleev M, Makhoul S, Gambaryan S, Jurk K (2021). Curcumin at low doses potentiates and at high doses inhibits ABT-737-induced platelet apoptosis. International Journal of Molecular Sciences 22: 5405. https://doi.org/10.3390/ijms22105405 [Google Scholar] [PubMed] [CrossRef]
Ryan JL, Heckler CE, Ling M, Katz A, Williams JP, Pentland AP, Morrow GR (2013). Curcumin for radiation dermatitis: A randomized, double-blind, placebo-controlled clinical trial of thirty breast cancer patients. Radiation Research 180: 34–43. https://doi.org/10.1667/RR3255.1 [Google Scholar] [PubMed] [CrossRef]
Sadeghi N, Mansoori A, Shayesteh A, Hashemi SJ (2020). The effect of curcumin supplementation on clinical outcomes and inflammatory markers in patients with ulcerative colitis. Phytotherapy Research 34: 1123–1133. https://doi.org/10.1002/ptr.6581 [Google Scholar] [PubMed] [CrossRef]
Sairenji T, Collins KL, Evans DV (2017). An update on inflammatory bowel disease. Primary Care 44: 673–692. https://doi.org/10.1016/j.pop.2017.07.010 [Google Scholar] [PubMed] [CrossRef]
Salh B, Assi K, Templeman V, Parhar K, Owen D, Gomez-Munoz A, Jacobson K (2003). Curcumin attenuates DNB-induced murine colitis. American Journal of Physiology Gastrointestinal and Liver Physiology 285: G235–G243. https://doi.org/10.1152/ajpgi.00449.2002 [Google Scholar] [PubMed] [CrossRef]
Scazzocchio B, Minghetti L, D’Archivio M (2020). Interaction between gut microbiota and curcumin: A new key of understanding for the health effects of curcumin. Nutrients 12: 2499. https://doi.org/10.3390/nu12092499 [Google Scholar] [PubMed] [CrossRef]
Schmidt C, Bielecki C, Felber J, Stallmach A (2010). Surveillance strategies in inflammatory bowel disease. Minerva Gastroenterologica e Dietologica 56: 189–201. [Google Scholar] [PubMed]
Schneider C, Gordon ON, Edwards RL, Luis PB (2015). Degradation of curcumin: From mechanism to biological implications. Journal of Agricultural and Food Chemistry 63: 7606–7614. https://doi.org/10.1021/acs.jafc.5b00244 [Google Scholar] [PubMed] [CrossRef]
Sharma RA, Euden SA, Platton SL, Cooke DN, Shafayat A et al. (2004). Phase I clinical trial of oral curcumin: Biomarkers of systemic activity and compliance. Clinical Cancer Research 10: 6847–6854. https://doi.org/10.1158/1078-0432.CCR-04-0744 [Google Scholar] [PubMed] [CrossRef]
Shoba G, Joy D, Joseph T, Majeed M, Rajendran R, Srinivas PS (1998). Influence of piperine on the pharmacokinetics of curcumin in animals and human volunteers. Planta Medica 64: 353–356. https://doi.org/10.1055/s-2006-957450 [Google Scholar] [PubMed] [CrossRef]
Singh DV, Godbole MM, Misra K (2013). A plausible explanation for enhanced bioavailability of P-gp substrates in presence of piperine: Simulation for next generation of P-gp inhibitors. Journal of Molecular Modeling 19: 227–238. https://doi.org/10.1007/s00894-012-1535-8 [Google Scholar] [PubMed] [CrossRef]
Singla V, Pratap Mouli V, Garg SK, Rai T, Choudhury BN et al. (2014). Induction with NCB-02 (curcumin) enema for mild-to-moderate distal ulcerative colitis—A randomized, placebo-controlled, pilot study. Journal of Crohn’s and Colitis 8: 208–214. https://doi.org/10.1016/j.crohns.2013.08.006 [Google Scholar] [PubMed] [CrossRef]
Slika L, Patra D (2020). A short review on chemical properties, stability and nano-technological advances for curcumin delivery. Expert Opinion on Drug Delivery 17: 61–75. https://doi.org/10.1080/17425247.2020.1702644 [Google Scholar] [PubMed] [CrossRef]
Slowinska-Solnica K, Pawlica-Gosiewska D, Gawlik K, Owczarek D, Cibor D, Pocztar H, Mach T, Solnica B (2021). Serum inflammatory markers in the diagnosis and assessment of Crohn’s disease activity. Archives of Medical Science 17: 252–257. https://doi.org/10.5114/aoms/130842 [Google Scholar] [PubMed] [CrossRef]
Sohal A, Alhankawi D, Sandhu S, Chintanaboina J (2021). Turmeric-induced hepatotoxicity: Report of 2 cases. International Medical Case Reports Journal 14: 849–852. https://doi.org/10.2147/IMCRJ.S333342 [Google Scholar] [PubMed] [CrossRef]
Stanic Z (2017). Curcumin, a compound from natural sources, a true scientific challenge—A review. Plant Foods for Human Nutrition 72: 1–12. https://doi.org/10.1007/s11130-016-0590-1 [Google Scholar] [PubMed] [CrossRef]
Sueth-Santiago V, Mendes-Silva GP, Decoté-Ricardo D, Lima MEF (2015). Curcumina, o pó dourado do açafrão-da-terra: Introspecções sobre química e atividades biológicas. Química Nova 38: 538–552. https://doi.org/10.5935/0100-4042.20150035 [Google Scholar] [CrossRef]
Sugimoto K, Ikeya K, Bamba S, Andoh A, Yamasaki H et al. (2020). Highly bioavailable curcumin derivative ameliorates crohn’s disease symptoms: A randomized, double-blind, multicenter study. Journal of Crohn’s and Colitis 14: 1693–1701. https://doi.org/10.1093/ecco-jcc/jjaa097 [Google Scholar] [PubMed] [CrossRef]
Sun J, Bi C, Chan HM, Sun S, Zhang Q, Zheng Y (2013). Curcumin-loaded solid lipid nanoparticles have prolonged in vitro antitumour activity, cellular uptake and improved in vivo bioavailability. Colloids and Surfaces B: Biointerfaces 111: 367–375. https://doi.org/10.1016/j.colsurfb.2013.06.032 [Google Scholar] [PubMed] [CrossRef]
Sun YP, Gu JF, Tan XB, Wang CF, Jia XB, Feng L, Liu JP (2016). Curcumin inhibits advanced glycation end product-induced oxidative stress and inflammatory responses in endothelial cell damage via trapping methylglyoxal. Molecular Medicine Reports 13: 1475–1486. https://doi.org/10.3892/mmr.2015.4725 [Google Scholar] [PubMed] [CrossRef]
Thota RN, Dias CB, Abbott KA, Acharya SH, Garg ML (2018). Curcumin alleviates postprandial glycaemic response in healthy subjects: A cross-over, randomized controlled study. Scientific Reports 8: 13679. https://doi.org/10.1038/s41598-018-32032-x [Google Scholar] [PubMed] [CrossRef]
Tian T, Wang Z, Zhang J (2017). Pathomechanisms of oxidative stress in inflammatory bowel disease and potential antioxidant therapies. Oxidative Medicene and Cellular Longevity 2017: 4535194. https://doi.org/10.1155/2017/4535194 [Google Scholar] [PubMed] [CrossRef]
Topcu-Tarladacalisir Y, Akpolat M, Uz YH, Kizilay G, Sapmaz-Metin M, Cerkezkayabekir A, Omurlu IK (2013). Effects of curcumin on apoptosis and oxidoinflammatory regulation in a rat model of acetic acid-induced colitis: The roles of c-Jun N-terminal kinase and p38 mitogen-activated protein kinase. Journal of Medicinal Food 16: 296–305. https://doi.org/10.1089/jmf.2012.2550 [Google Scholar] [PubMed] [CrossRef]
Torres J, Ellul P, Langhorst J, Mikocka-Walus A, Barreiro-de Acosta M et al. (2019). European crohn’s and colitis organisation topical review on complementary medicine and psychotherapy in inflammatory bowel disease. Journal of Crohn’s and Colitis 13: 673–685e. https://doi.org/10.1093/ecco-jcc/jjz051 [Google Scholar] [PubMed] [CrossRef]
Tossetta G, Fantone S, Giannubilo SR, Marzioni D (2021). The multifaced actions of curcumin in pregnancy outcome. Antioxidants 10: 126. https://doi.org/10.3390/antiox10010126 [Google Scholar] [PubMed] [CrossRef]
Trujillo J, Chirino YI, Molina-Jijon E, Anderica-Romero AC, Tapia E, Pedraza-Chaverri J (2013). Renoprotective effect of the antioxidant curcumin: Recent findings. Redox Biology 1: 448–456. https://doi.org/10.1016/j.redox.2013.09.003 [Google Scholar] [PubMed] [CrossRef]
Trujillo J, Granados-Castro LF, Zazueta C, Anderica-Romero AC, Chirino YI, Pedraza-Chaverri J (2014). Mitochondria as a target in the therapeutic properties of curcumin. Archiv der Pharmazie 347: 873–884. https://doi.org/10.1002/ardp.201400266 [Google Scholar] [PubMed] [CrossRef]
Ukil A, Maity S, Karmakar S, Datta N, Vedasiromoni JR, Das PK (2003). Curcumin, the major component of food flavour turmeric, reduces mucosal injury in trinitrobenzene sulphonic acid-induced colitis. British Journal of Pharmacology 139: 209–218. https://doi.org/10.1038/sj.bjp.0705241 [Google Scholar] [PubMed] [CrossRef]
Venkataranganna MV, Rafiq M, Gopumadhavan S, Peer G, Babu UV, Mitra SK (2007). NCB-02 (standardized Curcumin preparation) protects dinitrochlorobenzene-induced colitis through down-regulation of NFkappa-B and iNOS. World Journal of Gastroenterology 13: 1103–1107. https://doi.org/10.3748/wjg.v13.i7.1103 [Google Scholar] [PubMed] [CrossRef]
Wang Y, Tang Q, Duan P, Yang L (2018). Curcumin as a therapeutic agent for blocking NF-kappaB activation in ulcerative colitis. Immunopharmacology and Immunotoxicology 40: 476–482. https://doi.org/10.1080/08923973.2018.1469145 [Google Scholar] [PubMed] [CrossRef]
Wang Z, Zhang Y, Banerjee S, Li Y, Sarkar FH (2006). Notch-1 down-regulation by curcumin is associated with the inhibition of cell growth and the induction of apoptosis in pancreatic cancer cells. Cancer 106: 2503–2513. https://doi.org/10.1002/cncr.21904 [Google Scholar] [PubMed] [CrossRef]
Wanninger S, Lorenz V, Subhan A, Edelmann FT (2015). Metal complexes of curcumin—synthetic strategies, structures and medicinal applications. Chemical Society Reviews journal 44: 4986–5002. https://doi.org/10.1039/C5CS00088B [Google Scholar] [PubMed] [CrossRef]
Wei HX, Wang B, Li B (2020). IL-10 and IL-22 in mucosal immunity: Driving protection and pathology. Frontiers in Immunology 11: 1315. https://doi.org/10.3389/fimmu.2020.01315 [Google Scholar] [PubMed] [CrossRef]
Wei C, Wang JY, Xiong F, Wu BH, Luo MH et al. (2021). Curcumin ameliorates DSSinduced colitis in mice by regulating the Treg/Th17 signaling pathway. Molecular Medicine Reports 23: 34. https://doi.org/10.3892/mmr.2020.11672 [Google Scholar] [PubMed] [CrossRef]
Wu R, Xiong R, Li Y, Chen J, Yan R (2023). Gut microbiome, metabolome, host immunity associated with inflammatory bowel disease and intervention of fecal microbiota transplantation. Journal of Autoimmunity 31: 103062. https://doi.org/10.1016/j.jaut.2023.103062 [Google Scholar] [PubMed] [CrossRef]
Yang ZY, Li M (2022). The role of gut microbiota dysbiosis in the occurrence and development of inflammatory bowel disease and its prevention and control strategies. Zhonghua Yu Fang Yi Xue Za Zhi 56: 1175–1181 (In Chinese). https://doi.org/10.3760/cma.j.cn112150-20211008-00936 [Google Scholar] [PubMed] [CrossRef]
Yang KY, Lin LC, Tseng TY, Wang SC, Tsai TH (2007). Oral bioavailability of curcumin in rat and the herbal analysis from Curcuma longa by LC-MS/MS. Journal of Chromatography B: Analytical Technologies in the Biomedical and Life Sciences 853: 183–189. https://doi.org/10.1016/j.jchromb.2007.03.010 [Google Scholar] [PubMed] [CrossRef]
Yang JY, Zhong X, Kim SJ, Kim DH, Kim HS, Lee JS, Yum HW, Lee J, Na HK, Surh YJ (2018). Comparative effects of curcumin and tetrahydrocurcumin on dextran sulfate sodium-induced colitis and inflammatory signaling in mice. Journal of Cancer Prevention 23: 18–24. https://doi.org/10.15430/JCP.2018.23.1.18 [Google Scholar] [PubMed] [CrossRef]
Yildirim H, Sunay FB, Sinan S, Kockar F (2016). In vivo effects of curcumin on the paraoxonase, carbonic anhydrase, glucose-6-phosphate dehydrogenase and beta-glucosidase enzyme activities in dextran sulphate sodium-induced ulcerative colitis mice. Journal of Enzyme Inhibition and Medicinal Chemistry 31: 1583–1590. https://doi.org/10.3109/14756366.2016.1158173 [Google Scholar] [PubMed] [CrossRef]
Yue W, Liu Y, Li X, Lv L, Huang J, Liu J (2019). Curcumin ameliorates dextran sulfate sodium-induced colitis in mice via regulation of autophagy and intestinal immunity. The Turkish Journal of Gastroenterology 30: 290–298. https://doi.org/10.5152/tjg.2019.18342 [Google Scholar] [PubMed] [CrossRef]
Zeng Z, Zhan L, Liao H, Chen L, Lv X (2013). Curcumin improves TNBS-induced colitis in rats by inhibiting IL-27 expression via the TLR4/NF-κB signaling pathway. Planta Medical 79: 102–109. https://doi.org/10.1055/s-0032-1328057 [Google Scholar] [PubMed] [CrossRef]
Zhang X, Wu J, Ye B, Wang Q, Xie X, Shen H (2016). Protective effect of curcumin on TNBS-induced intestinal inflammation is mediated through the JAK/STAT pathway. BMC Complementary Alternative Medicine 16: 299. https://doi.org/10.1186/s12906-016-1273-z [Google Scholar] [PubMed] [CrossRef]
Zhang J, Zheng Y, Luo Y, Du Y, Zhang X, Fu J (2019). Curcumin inhibits LPS-induced neuroinflammation by promoting microglial M2 polarization via TREM2/TLR4/NF-kappaB pathways in BV2 cells. Molecular Immunology 116: 29–37. https://doi.org/10.1016/j.molimm.2019.09.020 [Google Scholar] [PubMed] [CrossRef]
Zhao HM, Han F, Xu R, Huang XY, Cheng SM et al. (2017). Therapeutic effect of curcumin on experimental colitis mediated by inhibiting CD8+CD11c+ cells. World Journal of Gastroenterology 23: 1804–1815. https://doi.org/10.3748/wjg.v23.i10.1804 [Google Scholar] [PubMed] [CrossRef]
Zhao NJ, Liao MJ, Wu JJ, Chu KX (2018). Curcumin suppresses Notch‐1 signaling: Improvements in fatty liver and insulin resistance in rats. Molecular Medicine Reports 17: 819–826. https://doi.org/10.3892/mmr.2017.7980 [Google Scholar] [PubMed] [CrossRef]
Zhao HM, Xu R, Huang XY, Cheng SM, Huang MF, Yue HY, Wang X, Zou Y, Lu AP, Liu DY (2016). Curcumin improves regulatory T cells in gut-associated lymphoid tissue of colitis mice. World Jounal of Gastroenterology 22: 5374–5383. https://doi.org/10.3748/wjg.v22.i23.5374 [Google Scholar] [PubMed] [CrossRef]
Zhdanovskaya N, Lazzari S, Caprioglio D, Firrincieli M, Maioli C et al. (2022). Identification of a novel curcumin derivative influencing notch pathway and DNA damage as a potential therapeutic agent in T-ALL. Cancers 14: 5772. https://doi.org/10.3390/cancers14235772 [Google Scholar] [PubMed] [CrossRef]
Zhong Y, Tu Y, Ma Q, Chen L, Zhang W, Lu X, Yang S, Wang Z, Zhang L (2023). Curcumin alleviates experimental colitis in mice by suppressing necroptosis of intestinal epithelial cells. Frontiers in Pharmacology 14: 1170637. https://doi.org/10.3389/fphar.2023.1170637 [Google Scholar] [PubMed] [CrossRef]
Zhong Y, Xiao Y, Gao J, Zheng Z, Zhang Z, Yao L, Li D (2022). Curcumin improves insulin sensitivity in high-fat diet-fed mice through gut microbiota. Nutrition & Metabolism 19: 76. https://doi.org/10.1186/s12986-022-00712-1 [Google Scholar] [PubMed] [CrossRef]
Zmora N, Levy M, Pevsner-Fishcer M, Elinav E (2017). Inflammasomes and intestinal inflammation. Mucosal Immunology 10: 865–883. https://doi.org/10.1038/mi.2017.19 [Google Scholar] [PubMed] [CrossRef]
Zou T, Li S, Wang B, Wang Z, Liu Y, You J (2021). Curcumin improves insulin sensitivity and increases energy expenditure in high-fat-diet-induced obese mice associated with activation of FNDC5/irisin. Nutrition 90: 111263. https://doi.org/10.1016/j.nut.2021.111263 [Google Scholar] [PubMed] [CrossRef]
Cite This Article
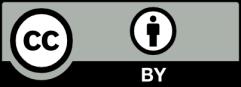
This work is licensed under a Creative Commons Attribution 4.0 International License , which permits unrestricted use, distribution, and reproduction in any medium, provided the original work is properly cited.