Open Access
ARTICLE
MBD2 promotes Th2 differentiation in ovalbumin-induced CD4+ T cells
1 Department of Respiratory and Critical Care Medicine, Affiliated Hospital of Guilin Medical University, Guilin, 541001, China
2 Laboratory of Respiratory Disease, Affiliated Hospital of Guilin Medical University, Guilin, 541001, China
* Corresponding Author: LIBING MA. Email:
# Co-first authors: Qilu Pan and Yan Jiang
BIOCELL 2023, 47(11), 2495-2502. https://doi.org/10.32604/biocell.2023.042617
Received 06 June 2023; Accepted 20 September 2023; Issue published 27 November 2023
Abstract
Introduction: Allergen-specific CD4+ T cells play a central role in autoimmune disorders, allergies and asthma, with Th2-type immunity being the typical functional response of CD4+ T cells. This study aimed to investigate the role of MBD2 in regulating Th2 cell differentiation. Methods: Splenic mononuclear cells were extracted from C57BL/6 mice, and CD4+ T cells were isolated using magnetic beads and confirmed through flow cytometry. Lentivirus was employed to construct MBD2-silenced CD4+ T cells. In vitro experiments were performed to treat splenogenic mononuclear cells and CD4+ T cells with Ovalbumin (OVA), and Th2 cell ratios and IL-4 levels were assessed using flow cytometry and ELISA. Results: The purity of the isolated CD4+ T cells was 95.73%, confirming successful isolation of primary CD4+ T cells. Compared to the control group, the Th2 cell ratio exhibited an increase in the Th2-induced group. Treatment with 5-Aza (concentrations, 1–100 μM) promoted Th2 cell differentiation and increased IL-4 levels. Notably, when combined with Th2 induction and 10 μM 5-Aza treatment, silencing MBD2 further amplified Th2 cell ratios and elevated IL-4 levels in cell supernatants. Furthermore, OVA (concentration, 200 μg/mL) induced the differentiation of CD4+ T cells into Th2 cells and increased IL-4 secretion. Interestingly, silencing MBD2 significantly increased the Th2 cell ratio and IL-4 levels in OVA-treated CD4+ T cells. Conclusion: In summary, OVA promoted CD4+ T cell differentiation into Th2 cells and enhanced IL-4 levels. MBD2 was identified as a mediator of Th2 cell differentiation in splenic-derived CD4+ T cells, influenced by OVA or 5-Aza treatment.Keywords
Upon activation, naïve CD4+ T cells differentiate into distinct T-helper (Th) subsets, each producing a unique subset of cytokines that play pivotal roles in the immune response against various infections and are also implicated in autoimmune conditions, allergies and asthma (Zhu, 2018). CD4+ T helper cells are essential components of adaptive immune responses to pathogens and are critical for maintaining host immune homeostasis (Yang et al., 2020). Cytokines generated by antigen-presenting cells and other innate immune cells can influence the effector programs of CD4+ T cells, including Th1, Th2 and Th17 cells (Ruterbusch et al., 2020). In asthma, the maturation and function of CD4+ T cells are characterized by Th2/Th17 type immunity (Pacholewska et al., 2017). Therefore, elucidating the mechanism of Th2 cell differentiation in CD4+ T cells could provide significant insight for improving the treatment of autoimmune disorders, allergies and asthma.
Early-life malnutrition triggers hypomethylation of the Th2 cytokine site in CD4+ T cells through the mechanistic target, resulting in cell activation, proliferation, Th2 skew, and increased susceptibility to experimental asthma (Chen et al., 2019). Research has indicated that low concentrations of MBD2 may enhance IL-4 activity (Decramer et al., 2008). Th2 cells are known for producing cytokines such as IL-4, IL-5 and IL-13, among which IL-4’s capacity to drive Th2 cell development is pivotal in allergic asthma (Zeng et al., 2018). Notably, high exposure to PM2.5 in the month preceding a visit is independently linked to methylation of the IL-4 CpG24 locus (Prunicki et al., 2021). In addition, it has been shown that reduced DNA methylation in the IL-4 promoter region can intensify Th2-based immune response by naïve HFD CD4+ T cells (Lin et al., 2022). Therefore, the modulation of Th2 differentiation holds promise for exploring innovative approaches in the treatment of autoimmune disorders, allergies and asthma.
In allergic asthma, specific the airway and participate in both innate and adaptive immune responses within the airway (Ling and Luster, 2016). Early-life exposure to exogenous substances that bind to aryl hydrocarbon receptors (AhR) has been shown to induce DNA methylation bias and functional alterations in CD4+ T cells (Burke et al., 2021). At the same time, Th2 cells play a role in promoting IgE-mediated sensitization, airway hyperresponsiveness, and eosinophilia (Muehling et al., 2017). However, the precise role of the DNA methylation inhibitor, 5-Aza-2′-deoxycytidine (5-Aza), in the differentiation of Th2 immune cells into CD4+ T cells in respiratory diseases remains unclear.
Methyl-CpG-binding domain protein 2 (MBD2) interprets the information encoded within DNA methylomes by binding to methylated CpG DNA, thereby exerting regulatory control over target gene expression at the transcriptional level (Yue et al., 2022). Notably, in patients with severe asthma, MBD2 exhibits a negative correlation with Th2 cells (Chen et al., 2021). Furthermore, in the absence of MBD2, dendritic cells display a significantly impaired capacity to initiate a Th2 immune response against parasitic worms or allergens (Cook et al., 2015). Thus, we hypothesized that MBD2 might play a role in mediating the differentiation of Th2 cells in asthma, although the specific mechanisms remain unclear. Therefore, in this study, we aimed to investigate the influence of MBD2 on the differentiation of Th2 cells in CD4+ T cells induced by 5-Aza (Brand et al., 2012) or OVA (Lertnimitphun et al., 2021) to increase our understanding on asthma and propose novel insights that could be used to improve treatment outcomes.
Spleen-derived mononuclear cells and CD4+ T cells were extracted from male C57BL/6 mice, as described in our previous research (Jiang et al., 2023), and splenogenic mononuclear cells were categorized into the following groups: Control, 50, 100, and 200 μg/mL OVA (323–339, Invivogen, France).
CD4+ T cells, with or without 200 μg/mL OVA treatment, were divided into wild-type, sh-NC, and sh-MBD2 groups. The construction of MBD2-silenced CD4+ T cells was carried out in accordance with our previous study (Jiang et al., 2023).
Based on these designated groups, Th2-induced differentiation and 5-Aza treatment were performed, and the groups were divided into control, Th2-induced, 5-Aza, and Th2+5-Aza groups. The cells were incubated with 2 μg/mL anti-CD3 (100340, Biolegend, San Diego, California, USA) + 2 μg/mL anti-CD28 (102116, Biolegend, San Diego, California, USA) in control. Th2-induction was incubated with 2 μg/mL anti-CD3 + 2 μg/mL anti-CD28 + 10 ng/mL anti-IL-12 (505307, Biolegend, San Diego, California, USA) + 10 μg/mL IL-4 (214-14-500, peprotech, Cranberry, New Jersey, USA) and treated for 4 days. Moreover, 5-Aza (1, 10 and 100 μM, ab142744, Abcam, Cambridge, UK) were performed half 1 h before the Th2 induction.
Cells from each group were suspended and stimulated with a stimulation cocktail, following which the cells were washed and centrifuged with 0.5% BSA-PBS (1 mL). Then, 500 μL of cell fixation buffer was added to fix the cells, and the cell precipitate was resuscitated with 1 × membrane-breaking buffer (1 mL), followed by resuspension. Each cell group was treated and thoroughly mixed with CD4-APC antibody (0.5 μL, 17-0042-82, ebioscience, San Diego, California, USA), CD4-APC antibody (0.5 μL, 17-0042-82, ebioscience, San Diego, California, USA) or IL-4-FITC antibody (2 μL, 53-7041-82, ebioscience, San Diego, California, USA). After incubation, staining tubes and single staining tubes were prepared, and the cells were washed and resuspended in 0.5% BSA-PBS for flow cytometry (A00-1-1102, Beckman, Pasadena, California, USA) detection.
For IL-4 level analysis, cell culture supernatant samples were centrifuged at 1,000 g at 2°C–8°C for 15 min, and the resulting supernatant was used for detection. The level of IL-4 was analyzed using an IL-4 assay kit (KE10010, Proteintech, Chicago, USA) and a multifunctional enzyme label analyzer (MB-530, HEALES, Shenzhen, China).
Data analysis was performed using GraphPad Prism 8.0 software (San Diego, California, USA). Measurement data are presented as mean ± standard deviation. Normality and homogeneity of variance tests were conducted to ensure data met the assumptions of the analysis. For comparisons between two groups, an unpaired t-test was used, while one-way analysis of variance (ANOVA) or repeated measures ANOVA was used comparing multiple groups. Tukey’s post-hoc test was performed when appropriate. p < 0.05 was used to determine statistical significance.
Purity identification of mouse CD4+ T cells after positive sorting
Flow cytometry analysis confirmed the purity of CD4+ T cells to be 95.73% (Fig. 1), demonstrating the successful isolation of primary CD4+ T cells derived from mouse spleen, thereby allowing us to proceed with subsequent experiments.
Figure 1: Flow cytometry for identifying the purity of CD4+ T cells.
5-Aza induced the differentiation of Th2 cell and IL-4 secretion
Following Th2-induction, we observed an increase in the Th2 cell ratio (Figs. 2A and 2B). Moreover, 5-Aza treatment demonstrated a dose-dependent promotion of Th2 cell differentiation (Figs. 2A and 2B) and enhanced IL-4 secretion (Fig. 2C). Based on these findings, we selected 10 μM 5-Aza for subsequent experiments, demonstrating that 5-Aza can induce Th2 differentiation from CD4+ T cells.
Figure 2: 5-Aza induced Th2 differentiation from CD4+ T cells. (A) and (B) Th2 cell ratio. (C) IL-4 level. *p < 0.05 vs. normal; #p < 0.05 vs. Th2-induced. n = 3.
MBD2 affected the differentiation of Th2 cells
Silencing MBD2 promoted Th2 differentiation from CD4+ T cells under the influence of both 10 μM 5-Aza and Th2 induction (Figs. 3A and 3B) and also increased IL-4 levels in the cell supernatant when subjected to 10 μM 5-Aza and Th2 induction (Fig. 3C). These findings indicate that silencing MBD2 could induce Th2 cell differentiation.
Figure 3: MBD2-mediated Th2 cell differentiation. (A) and (B) Th2 cells proportion. (C) IL-4 level. *p < 0.05 vs. sh-NC in Control, Th2-induced, 5-Aza, or Th2+5-Aza groups. n = 3.
OVA promoted Th2 cell differentiation and the secretion of IL-4
The proportion of Th2 cells significantly increased with increasing OVA doses (Figs. 4A and 4B). Furthermore, our analysis of secreted factors from splenogenic mononuclear cells exposed to OVA treatment revealed a substantial increase in IL-4 levels within the OVA treatment group compared to the control group (Fig. 4C). Importantly, the highest observed Th2 cell ratio and IL-4 levels were in the 200 μg/mL OVA group compared to the other groups (Fig. 4C). These findings demonstrate that OVA treatment promotes Th2 cell differentiation and increases IL-4 secretion.
Figure 4: Different doses of OVA induced Th2 cell differentiation. (A) and (B) Ratio of Th2 cells. (C) IL-4 level. #p < 0.05 vs. Control. n = 3.
Silencing of MBD2 promoted the role of OVA on Th2 cell differentiation
No significant change was observed in the ratio of Th2 cells and IL-4 levels between the wild-type and sh-NC groups (Figs. 5A–5C), but a significant increase was observed in both the ratio of Th2 cells and the IL-4 levels in the sh-MBD2 group (Figs. 5A–5C), suggesting that silencing MBD2 promotes Th2 cell differentiation and enhances IL-4 levels induced by OVA.
Figure 5: Silencing of MBD2 promotes the action of OVA on Th2 cell differentiation. (A) and (B) Th2 cell proportion. (C) IL-4 levels. #p < 0.05 vs. sh-NC. n = 3.
The addition of various cytokine combinations to induce initial CD4+ T cell activation in vitro is a valuable strategy for assessing the development of specific CD4+ T helper subpopulations (Flaherty and Reynolds, 2015). Immature CD4+ T lymphocytes differentiate into highly allergic T helper 2 cells, inducing the expression of interleukin IL-4, inhibiting the expression of interferon IFN-γ, and accompanied by uniform DNA methylation of these gene promoters (Tang et al., 2012). 5-Aza serves as a DNA methyltransferase inhibitor, enhancing the function and stability of regulatory T cells (Varanasi et al., 2017). Although treatment with varying concentrations of 5-Aza (1–100 μM) has been shown to promote Th2 cell differentiation and elevate IL-4 levels and DNA methylation is known to play a role in Th2 cell differentiation, the precise mechanisms in asthma warrant further investigation.
CD4+ T-helper (Th) cells are pivotal for maintaining immune homeostasis and host defense, and their dysregulation contributes to the development of autoimmune diseases critical for the development of various autoimmune diseases (Pawlak et al., 2020). This intricate process involves the activation of signal transducers, transcriptional activators, and master regulatory transcription factors that guide the function of each distinct helper T lymphocyte subtype (Chalmin et al., 2018). Notably, 5-Aza-2’-deoxycytidine-mediated demethylation was shown to enhance the differentiation of naïve T cells into regulatory T cells (Tregs) in chronic HBV infection (Fang et al., 2021). Our findings indicate that silencing MBD2 facilitates Th2 cell differentiation in CD4+ T cells and enhances IL-4 levels in cell supernatants, especially when combined with 10 μM 5-Aza and Th2 induction.
Research has demonstrated that low-dose 5-Aza (1–10 μM) promotes the generation and differentiation of regulatory T (Treg) cells and enhances their immune-suppressive function (Han et al., 2021). Similarly, human CD4+ CD25− T cells treated with low-dose 5-Aza-2’-deoxycytidine (5 μM) exhibit reduced proliferation without inhibitory effects (Kehrmann et al., 2014). These findings align with our use of a 10 μM 5-Aza dosage in this present study. Further, animal experiments also support the benefits of low-dose 5-Aza in immune regulation. For instance, short-term pretreatment with 5-Aza (0.15 mg/kg) significantly inhibits disease activity in experimental autoimmune encephalomyelitis mice and improves inflammatory responses in the central nervous system (Chan et al., 2014). Low-dose (0.15 mg/kg) 5-Aza significantly inhibits FoxP3 methylation, rebalances the Treg/Th17 ratio, and mitigates liver damage and inflammation in mice with primary biliary cholangitis (Jiang et al., 2021). Taken together, our study highlights that low-dose 5-Aza (10 μM) effectively promotes Th2 cell differentiation and modulates the immune system.
Identifying biomarkers for allergic asthma holds great potential for disease monitoring, early diagnosis, and treatment (Breiteneder et al., 2020). CD4+ T cells expressing chemoreceptor homologous molecules, such as CRTH2, play a pivotal role in determining Th2 cell subsets (Palikhe et al., 2016). These cells are identified as allergen-specific central memory Th2 cells, and an increased presence of circulating CD4+ CRTH2+ T cells has been observed to be a distinct feature of severe asthma (Palikhe et al., 2016; Shrestha Palikhe et al., 2021). OVA-related sensitization and Th2 immune response are crucial in mediating asthma (Lin et al., 2022). Our study demonstrates that a high dose of OVA induces an increase in Th2 cells and the secretion of the cytokine IL-4, highlighting the significance of OVA in the immune response associated with allergic asthma.
MBD2 expression was found to be elevated in peripheral blood CD4+ T cells from individuals with asthma (Jia et al., 2017). Asthma is characterized by abnormal accumulation, differentiation, or function of memory CD4+ T cells that produce Th2 cells (Seumois et al., 2014). Notably, macrophage-specific knockout of MBD2 has been shown to protect mice from OVA-induced allergic airway inflammation and inhibit M2 procedures (Wu et al., 2022). In our study, MBD2 silencing was found to significantly increase the ratio of Th2 cells and the levels of IL-4 in OVA-induced CD4+ T cells, providing evidence that MBD2 promotes Th2 cell differentiation from CD4+ T cells induced by OVA.
It is important to acknowledge that our research only confirmed the expression of MBD2 in OVA-induced CD4+ T cells and its role in promoting Th2 cell differentiation in cell experiments. However, due to limitations in experimental funding and technology at our institution, we were unable to generate or acquire a sufficient number of MBD2 knockout mice for in vivo experiments, underscoring the need for further investigation in the future.
In conclusion, this study shows that OVA can induce Th2 cell differentiation and increase the IL-4 levels in CD4+ T cells and suggests that MBD2 plays a key role in modulating IL-4 levels by mediating the differentiation of Th2 cells in splenic-derived CD4+ T cells in response to OVA or 5-Aza treatment.
Acknowledgement: The authors would like to thank the Affiliated Hospital of Guilin Medical University for their technical assistance.
Funding Statement: This work was supported by grants from the National Natural Science Foundation of China (Nos. 81760009 and 81560007).
Author Contributions: The authors confirm contribution to the paper as follows: Q. Pan: Writing-original draft; Conceptualization. Y. Jiang: Writing-original draft; Methodology. L. Li, X. Du, Q. Han, F. Ling, R. Li, S. Chu, L. Mai, and J. Huang: Data curation; Formal analysis; Visualization. L. Ma: Design; Writing-review & editing; Project administration. All authors reviewed the results and approved the final version of the manuscript.
Availability of Data and Materials: All data generated or analyzed during this study are included in this article.
Ethics Approval: This experiment was approved by the ethics committee of the Affiliated Hospital of Guilin Medical University (No. 201703190). All methods were carried out in accordance with relevant guidelines and regulations.
Conflicts of Interest: The authors declare that they have no conflicts of interest to report regarding the present study.
References
Brand S, Kesper DA, Teich R, Kilic-Niebergall E, Pinkenburg O, Bothur E, Lohoff M, Garn H, Pfefferle PI, Renz H (2012). DNA methylation of TH1/TH2 cytokine genes affects sensitization and progress of experimental asthma. The Journal of Allergy and Clinical Immunology 129: 1602–1610.e1606. [Google Scholar] [PubMed]
Breiteneder H, Peng YQ, Agache I, Diamant Z, Eiwegger T et al. (2020). Biomarkers for diagnosis and prediction of therapy responses in allergic diseases and asthma. Allergy 75: 3039–3068. [Google Scholar] [PubMed]
Burke CG, Myers JR, Post CM, Boulé LA, Lawrence BP (2021). DNA methylation patterns in CD4+ T cells of naïve and influenza a virus-infected mice developmentally exposed to an aryl hydrocarbon receptor ligand. Environmental Health Perspectives 129: 17007. [Google Scholar]
Chalmin F, Humblin E, Ghiringhelli F, Végran F (2018). Transcriptional programs underlying CD4 T cell differentiation and functions. International Review of Cell and Molecular Biology 341: 1–61. [Google Scholar] [PubMed]
Chan MW, Chang CB, Tung CH, Sun J, Suen JL, Wu SF (2014). Low-dose 5-aza-2'-deoxycytidine pretreatment inhibits experimental autoimmune encephalomyelitis by induction of regulatory T cells. Molecular Medicine 20: 248–256. [Google Scholar] [PubMed]
Chen X, Lin H, Yang D, Xu W, Liu G, Liu X, Sheng J, Huang H (2019). Early-life undernutrition reprograms CD4+ T-cell glycolysis and epigenetics to facilitate asthma. The Journal of Allergy and Clinical Immunology 143: 2038-2051.e2012. [Google Scholar]
Chen Z, Yuan Y, He Y, Wasti B, Duan W et al. (2021). MBD2 as a potential novel biomarker for identifying severe asthma with different endotypes. Frontiers in Medicine 8: 693605. [Google Scholar] [PubMed]
Cook PC, Owen H, Deaton AM, Borger JG, Brown SL et al. (2015). A dominant role for the methyl-CpG-binding protein Mbd2 in controlling Th2 induction by dendritic cells. Nature Communications 6: 6920. [Google Scholar] [PubMed]
Decramer M, Rennard S, Troosters T, Mapel DW, Giardino N, Mannino D, Wouters E, Sethi S, Cooper CB (2008). COPD as a lung disease with systemic consequences—clinical impact, mechanisms, and potential for early intervention. COPD: Journal of Chronic Obstructive Pulmonary Disease 5: 235–256. [Google Scholar] [PubMed]
Fang Y, Yuan XD, Liu HH, Xiang L, Chen LM, Fan YC, Gao S, Wang K (2021). 5-Aza-2′-deoxycytidine may enhance the frequency of T regulatory cells from CD4+ naïve T cells isolated from the peripheral blood of patients with chronic HBV infection. Expert Review of Clinical Immunology 17: 177–185. [Google Scholar] [PubMed]
Flaherty S, Reynolds JM (2015). Mouse naïve CD+ T cell isolation and in vitro differentiation into T cell subsets. JoVE Journal. https://doi.org/10.3791/52739 [Google Scholar] [PubMed] [CrossRef]
Han P, Hou Y, Zhao Y, Liu Y, Yu T et al. (2021). Low-dose decitabine modulates T-cell homeostasis and restores immune tolerance in immune thrombocytopenia. Blood 138: 674–688. [Google Scholar] [PubMed]
Jia A, Wang Y, Sun W, Xiao B, Wei Y et al. (2017). MBD2 regulates Th17 cell differentiation and experimental severe asthma by affecting IRF4 expression. Mediators of Inflammation 2017: 6249685. [Google Scholar] [PubMed]
Jiang Y, Li L, Pan Q, Du X, Han Q et al. (2023). Methyl-cpg-binding domain protein 2 silencing inhibits Th17 differentiation of CD4+T cells induced by ovalbumin. Iranian Journal of Immunology: IJI 20: 45–56. [Google Scholar] [PubMed]
Jiang T, Zhang HW, Wen YP, Yin YS, Yang LH et al. (2021). 5-Aza-2-deoxycytidine alleviates the progression of primary biliary cholangitis by suppressing the FoxP3 methylation and promoting the Treg/Th17 balance. International Immunopharmacology 96: 107820. [Google Scholar] [PubMed]
Kehrmann J, Tatura R, Zeschnigk M, Probst-Kepper M, Geffers R, Steinmann J, Buer J (2014). Impact of 5-aza-2′-deoxycytidine and epigallocatechin-3-gallate for induction of human regulatory T cells. Immunology 142: 384–395. [Google Scholar] [PubMed]
Lertnimitphun P, Zhang W, Fu W, Yang B, Zheng C et al. (2021). Safranal alleviated OVA-induced asthma model and inhibits mast cell activation. Frontiers in Immunology 12: 585595. [Google Scholar] [PubMed]
Lin H, Zhao Y, Zhu Y, Li C, Xu W, Chen X, Huang H, Jin L (2022). Maternal high-fat diet aggravates allergic asthma in offspring via modulating CD4+ T-cell differentiation. Nutrients 14: 2508. [Google Scholar] [PubMed]
Ling MF, Luster AD (2016). Allergen-specific CD4+ T cells in human asthma. Annals of the American Thoracic Society 13 Suppl 1: S25–S30. [Google Scholar] [PubMed]
Muehling LM, Lawrence MG, Woodfolk JA (2017). Pathogenic CD4+ T cells in patients with asthma. The Journal of Allergy and Clinical Immunology 140: 1523–1540. [Google Scholar] [PubMed]
Pacholewska A, Kraft MF, Gerber V, Jagannathan V (2017). Differential expression of serum microRNAs supports CD4+ T cell differentiation into Th2/Th17 cells in severe equine asthma. Genes 8: 383. [Google Scholar] [PubMed]
Palikhe NS, Laratta C, Nahirney D, Vethanayagam D, Bhutani M, Vliagoftis H, Cameron L (2016). Elevated levels of circulating CD4+ CRTh2+ T cells characterize severe asthma. Clinical and Experimental Allergy 46: 825–836. [Google Scholar] [PubMed]
Pawlak M, Ho AW, Kuchroo VK (2020). Cytokines and transcription factors in the differentiation of CD4+ T helper cell subsets and induction of tissue inflammation and autoimmunity. Current Opinion in Immunology 67: 57–67. [Google Scholar] [PubMed]
Prunicki M, Cauwenberghs N, Lee J, Zhou X, Movassagh H et al. (2021). Air pollution exposure is linked with methylation of immunoregulatory genes, altered immune cell profiles, and increased blood pressure in children. Scientific Reports 11: 4067. [Google Scholar] [PubMed]
Ruterbusch M, Pruner KB, Shehata L, Pepper M (2020). In vivo CD4+ T cell differentiation and function: Revisiting the Th1/Th2 paradigm. Annual Review of Immunology 38: 705–725. [Google Scholar] [PubMed]
Seumois G, Chavez L, Gerasimova A, Lienhard M, Omran N et al. (2014). Epigenomic analysis of primary human T cells reveals enhancers associated with TH2 memory cell differentiation and asthma susceptibility. Nature Immunology 15: 777–788. [Google Scholar] [PubMed]
Shrestha Palikhe N, Wu Y, Konrad E, Gandhi VD, Rowe BH, Vliagoftis H, Cameron L (2021). Th2 cell markers in peripheral blood increase during an acute asthma exacerbation. Allergy 76: 281–290. [Google Scholar] [PubMed]
Tang WY, Levin L, Talaska G, Cheung YY, Herbstman J, Tang D, Miller RL, Perera F, Ho SM (2012). Maternal exposure to polycyclic aromatic hydrocarbons and 5′-CpG methylation of interferon-γ in cord white blood cells. Environmental Health Perspectives 120: 1195–1200. [Google Scholar] [PubMed]
Varanasi SK, Reddy PBJ, Bhela S, Jaggi U, Gimenez F, Rouse BT (2017). Azacytidine treatment inhibits the progression of herpes stromal keratitis by enhancing regulatory T cell function. Journal of Virology 91: e02367. [Google Scholar] [PubMed]
Wu GR, Zhou M, Wang Y, Zhou Q, Zhang L et al. (2022). Blockade of Mbd2 by siRNA-loaded liposomes protects mice against OVA-induced allergic airway inflammation via repressing M2 macrophage production. Frontiers in Immunology 13: 930103. [Google Scholar] [PubMed]
Yang W, Chen X, Hu H (2020). CD4+ T-cell differentiation in vitro. Methods in Molecular Biology 2111: 91–99. [Google Scholar] [PubMed]
Yue T, Sun F, Wang F, Yang C, Luo J et al. (2022). MBD2 acts as a repressor to maintain the homeostasis of the Th1 program in type 1 diabetes by regulating the STAT1-IFN-γ axis. Cell Death and Differentiation 29: 218–229. [Google Scholar] [PubMed]
Zeng Z, Li M, Chen J, Li Q, Ning Q, Zhao J, Xu Y, Xie J, Yu J (2018). Reduced MBD2 expression enhances airway inflammation in bronchial epithelium in COPD. International Journal of Chronic Obstructive Pulmonary Disease 13: 703–715. [Google Scholar] [PubMed]
Zhu J (2018). T helper cell differentiation, heterogeneity, and plasticity. Cold Spring Harbor Perspectives in Biology 10: a030338. [Google Scholar] [PubMed]
Cite This Article
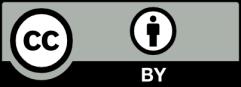