Open Access
REVIEW
Pharmacological effects of denervated muscle atrophy due to metabolic imbalance in different periods
1 Department of Prenatal Screening and Diagnosis Center, Affiliated Maternity and Child Health Care Hospital of Nantong University, Nantong, 226001, China
2 Nantong Institute of Genetics and Reproductive Medicine, Affiliated Maternity and Child Health Care Hospital of Nantong University, Nantong, 226001, China
3 Department of Emergency Medicine, Affiliated Maternity and Child Health Care Hospital of Nantong University, Nantong, 226001, China
4 Medical School of Nantong University, Nantong University, Nantong, 226001, China
5 School of Life Sciences, Nantong University, Nantong, 226001, China
* Corresponding Authors: HAIBO ZHANG. Email: ; ZHENYU ZHANG. Email:
BIOCELL 2023, 47(11), 2351-2359. https://doi.org/10.32604/biocell.2023.031043
Received 11 May 2023; Accepted 25 August 2023; Issue published 27 November 2023
Abstract
Denervation-induced skeletal muscle atrophy can potentially cause the decline in the quality of life of patients and an increased risk of mortality. Complex pathophysiological mechanisms with dynamic alterations have been documented in skeletal muscle atrophy resulting from innervation loss. Hence, an in-depth comprehension of the key mechanisms and molecules governing skeletal muscle atrophy at varying stages, along with targeted treatment and protection, becomes essential for effective atrophy management. Our preliminary research categorizes the skeletal muscle atrophy process into four stages using microarray analysis. This review extensively discusses the pathways and molecules potentially implicated in regulating the four stages of denervation and muscle atrophy. Notably, drugs targeting the reactivare oxygen species stage and the inflammation stage assume critical roles. Timely intervention during the initial atrophy stages can expedite protection against skeletal muscle atrophy. Additionally, pharmaceutical intervention in the ubiquitin-proteasome pathway associated with atrophy and autophagy lysosomes can effectively slow down skeletal muscle atrophy. Key molecules within this stage encompass MuRF1, MAFbx, LC3II, p62/SQSTM1, etc. This review also compiles a profile of drugs with protective effects against skeletal muscle atrophy at distinct post-denervation stages, thereby augmenting the evidence base for denervation-induced skeletal muscle atrophy treatment.Keywords
As a pivotal effector organ within the peripheral nervous system, the structural integrity and functional preservation of skeletal muscle are subject to control and regulation by the nervous system. Peripheral nerve injury arising from acute trauma results in the loss of innervation from skeletal muscle. This initiates a sequence of pathological changes, including reduced muscle fiber cross-sectional area, myofibril degradation with destruction of sarcomeres, diminished contraction speed, and fibrosis, ultimately culminating atrophy (Dumitru et al., 2018). The regeneration rate of peripheral nerves is slow after injury. As a result, the target muscles of severe patients become irreversibly atrophied before being re-innervated by the nerves. This can even lead to disabling in the patients, bringing a heavy burden to the family and society (Gu et al., 2011). Therefore, a series of problems in target muscle repair and functional reconstruction after peripheral nerve injury could be resolved by exploring novel and effective methods for the treatment of skeletal muscle atrophy.
Skeletal muscle atrophies upon innervation loss due to perturbations in the balance between protein synthesis and degradation. Notably, protein degradation pathways become activated, while protein synthesis pathways are repressed, constituting the underlying cause of skeletal muscle atrophy. Effective alleviation of skeletal muscle atrophy is promoted by inhibition of protein degradation and stimulation of protein synthesis (Lang et al., 2017; Li et al., 2017; Cui et al., 2019). Several molecules are involved in the process of denervated muscular atrophy, and the mechanism of regulating denervated muscular atrophy is also extremely complicated. Recent years have witnessed many studies on denervated muscular atrophy at home and abroad, but most of these studies have focused on a single event, a single gene or protein (Castets et al., 2019; Janice Sanchez et al., 2019). A more comprehensive understanding of the denervated skeletal muscle atrophy process is attainable by elucidating pivotal regulators and therapeutic targets. Shen et al. (2019) employed microarray analysis to scrutinize target muscles at varying post-denervation time points, revealing that the 28-day period following a neural injury can be segmented into four distinct transcriptional phases, demarcated by three nodal transitions (Shen et al., 2019). These phases encompass the oxidative stress stage, inflammation stage, atrophy stage, and atrophic fibrosis stage. Consistent with previous research, the phenotype of skeletal muscle atrophy manifests between 36 h and 3 days, aligning with the aforementioned atrophy period (Shen et al., 2019; Qiu et al., 2021). Consequently, these research findings facilitate more optimal utilization of diverse methods for denervated skeletal muscle atrophy treatment. Several treatment modalities are currently proposed, encompassing electrical stimulation (Dow et al., 2005; Tamaki et al., 2017) and noncoding RNA (He et al., 2016; Li et al., 2017; Hitachi et al., 2019). Furthermore, extensive utilization of various food and drug extracts is evident in the treatment of denervated skeletal muscle atrophy.
The enhanced medical application of traditional Chinese medicine (TCM) is attributed to its multifaceted functionalities and its facile interaction with substances within the human body. Substantial strides have been made in studying the prevention and treatment of skeletal muscle atrophy using various drugs. Noteworthy achievements include effective myotube atrophy management through triptolide, an ingredient isolated from ancient Chinese herbal medicine. This agent triggers IRS-1 degradation and activates the FOXO3 pathway activation (Wang et al., 2020). Furthermore, withaferin A (WFA), a natural protein-specific binding waveform, mitigates nuclear factor kappa B (NF-κB)-mediated pro-inflammatory signaling, thereby alleviating cancer cachexia-induced skeletal muscle atrophy (Straughn and Kakar, 2019). This article highlights distinct stages of skeletal muscle atrophy, protein synthesis pathways, and other pathways. It systematically summarizes the role of food and drug extracts and other effects on the treatment of skeletal muscle atrophy, which can provide a reference for the clinical management of denervated skeletal muscle atrophy.
Drugs that Play a Protective Role by Inhibiting Protein Degradation
Inhibition of reactive oxygen species
An earlier study involving rats subjected to neural exchange suggested that heightened mitochondrial permeability transitions and increased apoptosis result in elevated mitochondrial ROS production, signifying mitochondrial dysfunction following denervation (Adhihetty et al., 2007). Reactive oxygen species (ROS) are accountable for maintaining the homeostasis of various physiological processes. A mounting body of evidence posits oxidative stress as a crucial regulator of muscle wasting (Jackman and Kandarian, 2004; Powers et al., 2005). Meanwhile, an initial stage in denervated skeletal muscle exposes aberrant ROS production. Thus, curbing excessive ROS production can ameliorate skeletal muscle atrophy. Compared to a control group, a delay in muscle atrophy mediated by ROS inhibition was reported by Kim et al. (2018). On injecting Oenothera odorata root extract (EVP) in both H2O2-treated C2C12 myoblasts and sciatic-denervated mice (Kim et al., 2018). They probed deeper into the mechanistic underpinnings of how reactive oxygen species oversee skeletal muscle atrophy. EVP suppressed superoxide dismutase 1 (SOD1) expression and augmented HSP70 expression in H2O2-treated C2C12 myoblasts and sciatic-neutralized mice. In addition, EVP regulates apoptotic signals, including caspase-3, B-cell lymphoma protein 2 (Bcl-2), Bcl-2-associated X (Bax), and ceramides. Administration of recombinant heat shock protein 70 delays peripheral muscle denervation in the SOD1 (G93A) mouse model of amyotrophic lateral sclerosis (Gifondorwa et al., 2012). O’Leary and Hood have previously shown that seven days of muscle disuse increases the expression of Beclin-1, as well as LC3-II, a known component of autophagy (O’Leary and Hood, 2009). These studies provide evidence for EVP’s protective effect and regulatory mechanism on denervation muscle atrophy. The glutathione content and malondialdehyde (MDA) levels are considered indicators of oxidative stress and were assayed in the denervated gastrocnemius muscle. As a free radical scavenger, adding vitamin E can restore glutathione levels and reduce MDA levels, further confirming the conclusion that oxidative stress accelerates muscle atrophy (Demiryurek and Babul, 2004). Indeed, increased oxidative damage after denervation resulted in increased ROS production, decreased surface hydrophobicity, and decreased enzymatic activities of glyceraldehyde-3-phosphate dehydrogenase and creatine kinase, while increased mitochondrial ROS may also play a signaling role (Pierce et al., 2006). Another compelling candidate for ROS-mediated signaling is NF-κB. The involvement of NF-κB in muscle atrophy is recognized. The inhibitor of apoptosis 1 (cIAP1) protein, a positive regulator of NF-κB signaling, exhibited upregulation in denervated muscles compared to non-denervated controls 14 days post-denervation. Genetic or pharmacological inhibition of cIAP1 curtailed canonical NF-κB signaling, thus attenuating denervation-induced muscle atrophy (Lala-Tabbert et al., 2019). Furthermore, mitochondrial ROS has been demonstrated to upregulate the expression of ubiquitin ligase, atrogin-1/MAFbx, potentially contributing to atrophy through enhanced protein degradation by the 26S proteasome system (Li et al., 2005). We found that pyrroloquinoline quinone (PQQ)-mediated reduction in the expression of reactive oxygen species and inflammatory factors results in a decrease in the expression of MuRF1 and MAFbx, ultimately improving denervated skeletal muscle atrophy (Qiu et al., 2018; Ma et al., 2019). Kuo from Taiwan revealed PQQ impairs denervation-induced skeletal muscle atrophy by activating PGC-1α and integrating mitochondrial electron transport chain complexes (Kuo et al., 2015). Previous research by our team reported a reduction of denervation-induced oxidative stress and inflammation mediated by salidroside, inhibiting muscle proteolysis and ultimately alleviating muscle atrophy caused by denervation (Huang et al., 2019).
Inhibition of pro-inflammatory factors
Apigenin is a natural plant flavonoid acclaimed for its anti-obesity, anti-inflammatory, antioxidant, and anti-cancer attributes. Apigenin was attributed to dose-dependent inhibition of collagenase activity implicated in rheumatoid arthritis (RA) and the suppression of lipopolysaccharide (LPS)-induced nitric oxide (NO) production in RAW 264.7 macrophage cells (Lee et al., 2007). In denervated gastrocnemius and soleus muscles, apigenin treatment impeded the upregulation of tumor necrosis factor alpha (TNF-α) and interleukin (IL)-6 expression (Choi et al., 2018). Furthermore, Shiota et al. (2015) reported that apigenin exerted inhibitory effects on atrogin-1/MAFbx expression and curbed the reduction in myotube diameter induced by LPS stimulation in the C2C12 murine cell line (Shiota et al., 2015).
Ficus carica L. (FCL.), a flowering plant, contains flavonoids, psoralen, and bergapten, renowned for their antioxidant, anti-inflammatory, and anti-apoptotic characteristics. They are also implicated in quelling IL-1β and IL-6 production in atrophic muscles, attenuating muscle inflammation by inhibiting nuclear factor NF-κB activation (Dai et al., 2020). A study by Aarti Yadav et al. identified quercetin as a dietary antioxidant flavonoid that curbed inflammation in myotubes, fully reinstating TNF and an a-induced reduction of myotube diameter (Kim et al., 2018). This reflects muscle morphology at the cellular level. Quercetin improves motor function and muscle mass in aged people (Yadav et al., 2022). These reported effects of flavonoids are in other skeletal muscle atrophy models rather than in denervation-induced skeletal muscle atrophy. However, apigenin, functioning as a bioactive flavone, augmented the fiber-cross sectional area by 0.1% in the gastrocnemius muscle of sciatic denervated mice over 2 weeks. The enhanced cross-sectional area was substantiated by diminished levels of TNF-α in the gastrocnemius and IL-6 in the soleus muscle (Choi et al., 2018). Buyang Huangwu Tang (BYHWT), a classic Chinese medicine formula, ameliorated the inflammatory response in denervation-dependent skeletal muscle atrophy rat models (Zhou et al., 2020). Wu et al. (2019) found that negative regulation of pro-inflammatory cytokine mediated by salidroside attenuates denervation-induced skeletal muscle atrophy. The discernible pathway involves the inflammation caused by denervation in skeletal muscle, leading to the generation of inflammatory cytokines, particularly IL-6. This, in turn, augments the phosphorylation of STAT3 and the expression of SOCS3, which consequently triggers the activation of the proteolytic pathway in the muscle. Salidroside curbed muscle proteolysis and muscle atrophy by tempering the inflammatory response triggered by innervation. This likely transpired through the inactivation of the STAT3/SOCS3 pathway (Wu et al., 2019).
Regulation of ubiquitin-proteasome and autophagy-lysosome pathway
Activation of proteolytic metabolism and inhibition of protein synthesis pathway are the primary causes of skeletal muscle atrophy following denervation. The proteolytic pathway chiefly encompasses the ubiquitin-proteasome system and the autophagy-lysosome system. Clenbuterol (CLE) is a beta-adrenergic receptor stimulant employed in treating muscle spasms and asthma (Bohorov et al., 1987; Pairet et al., 1997). In vivo and in vitro treatment with CLE was found to prohibit the transcriptional upregulation of atrophy-related Ub ligases (i.e., MAFbx and MuRF1) in denervated soleus muscles (Goncalves et al., 2012). MAFbx overexpression led to a reduction in cultured muscle cell line size, indicating accelerated protein catabolism. Conversely, MAFbx gene depletion impeded nerve transection-induced muscle loss (Bodine et al., 2001). Nandrolone decelerates denervation atrophy by repressing MAFbx and MuRF1 (Zhao et al., 2008). The results showed that Nandrolone inhibited the expression of MAFbx and MuRF1 in subacutely denervated muscles. Reduced MAFbx and MuRF1 expression 35 and 56 days after denervation, suggesting that protection against denervation atrophy is time-related. Furthermore, no decrease in MAFbx or MuRF1 expression was observed at 3, 7, 14, or 31 days when nandrolone failed to prevent denervation atrophy at these time points. This underscores a mechanistic association between lowered MAFbx or MuRF1 levels and attenuated atrophy. Irisin is a 112 amino acid glycosylated protein hormone formed by the proteolysis of FNDC5 (Schumacher et al., 2013). The pivotal role of irisin in rescuing skeletal muscle atrophy was substantiated by the significant increase in the denervated muscle mass following irisin injection. Estimating the protein levels of both MAFbx and MuRF1, it was observed that injection of irisin resulted in a marked reduction in MAFbx and MuRF1 protein levels in denervated muscle. These findings advocate that irisin treatment curbed the expression of key indicators of skeletal muscle wasting during denervation-induced muscle atrophy (Reza et al., 2017).
Phenolic compounds derived from plants deliver diverse health benefits, mitigating the risk of cardiovascular disease and cancer (Kris-Etherton et al., 2002). Moreover, the protective impact of phenolic compounds from olive oil on muscle atrophy has been validated. These compounds can also alleviate the insulin resistance of skeletal muscle induced by a high-fat diet (Fujiwara et al., 2017; Szychlinska et al., 2019). In terms of alkylresorcinols (ARs) in denervated muscle atrophy, dietary alkylresorcinol supplementation was reported to thwart muscle atrophy by restraining the expression of the related genes of the ubiquitin-proteasome and autophagy-lysosomal pathway (Hiramoto et al., 2018). This study demonstrated that AR ingestion prevented denervation-induced hindlimb muscle weight and muscle fiber size reductions. However, ubiquitin ligase and autophagy-related gene expression related to muscle proteolysis were somewhat higher in denervated mice on an ARs-supplemented diet (D-AR) compared to those on a normal diet (D-ND). Furthermore, the abundance of autophagy marker p62 was significantly higher in D-AR than in D-ND. Fish oil rich in n-3 polyunsaturated fatty acids, including eicosapentaenoic acid and docosahexaenoic acid, is known for maintaining muscle mass. However, numerous studies have underscored fish oil’s positive role in various skeletal muscle atrophy models, such as cancer cachexia (Whitehouse et al., 2001), acute starvation (Whitehouse and Tisdale, 2001), sepsis (Khal and Tisdale, 2008), arthritis (Castillero et al., 2009), hind-limb immobilization (You et al., 2010). Komiya et al. (2019) documented that fish oil regulates ubiquitin ligase transcriptional levels through the TNF-α signaling pathway but not the FOXO1 pathway. Nevertheless, dietary fish oil intake has demonstrated no significant effect on skeletal muscle mass loss induced by sciatic nerve denervation (Komiya et al., 2019). Furthermore, BYHWT treatment is also associated with decreased levels of skeletal muscle atrophy-specific molecules MAFbx and MuRF1. This finding aligns with the outcomes of inflammatory response and motor endplate alterations following BYHWT treatment (Zhou et al., 2020). NeuroHeal, a new neuroprotective drug for peripheral nerve injury, discovered using artificial intelligence is based on a combination of two approved drugs, acamprosate and ribavirin, which facilitate its preparation for clinical application. Decreased levels of MAFbX and MuRF1 proteins were evident in the NeuroHeal group in denervated muscles. Denervation-induced autophagy activation characterized by LC3II and p62/SQSTM1 levels was diminished by NeuroHeal, indicating blocked autophagy flux (Marmolejo-Martinez-Artesero et al., 2020).
Resveratrol, a natural phytochemical, is widely found in plants, fruits, and red wine (Zhu et al., 2017). Studies reveal that treatment with 0.5% of the food intake of resveratrol can alleviate muscle atrophy caused by innervation in mice. The reduction of the MAFbx-dependent system and the improvement of autophagy defects are responsible for this attenuation (Asami et al., 2018). Geranylgeraniol (GGOH), as a C20-type isoprene found in fruits, vegetables, and grains, serves as an intermediate product of the Mevalonate pathway and a precursor of Geranylgeranyl pyrophosphate (Muraguchi et al., 2011). Miyawaki et al. (2020) reported that GGOH administration increased muscle fiber size in denervation-induced skeletal muscle atrophy in vivo and curtailed denervation-induced MAFbx expression (Miyawaki et al., 2020). The inhibitory impact of GGOH on skeletal muscle atrophy could potentially be attributed to its androgen-inhibiting effects, subsequently curbing MAFbx and MuRF1 (Pires-Oliveira et al., 2010). In a hind limb unloading model, administration of quercetin to the gastrocnemius muscle curtailed MAFbx and MuRF1 expression, thereby suppressing skeletal muscle mass loss (Mukai et al., 2010). Isoflavones, natural organic compounds, inhibit denervation-induced apoptosis and muscle atrophy. Studies suggest that isoflavones can suppress MuRF1 transcriptional activity and myotube atrophy (Tabata et al., 2019).
In addition to the aforementioned roles of vitamin E in regulating reactive oxygen species during skeletal muscle atrophy, different vitamins also play significant roles in various stages of denervation-induced skeletal muscle atrophy. Vitamin C deficiency was reported to reduce muscle weight, elevate FOXO-1, MAFbx, and MuRF1 expression. Re-administration of vitamin C could restore muscle weight and lower gene expression in skeletal muscle atrophy (Takisawa et al., 2019). Vitamin D, another member of the vitamin family, participates in calcium absorption, utilization, and bone calcification. However, recent findings suggest its involvement in skeletal muscle functions in different situations. In mice, vitamin D receptor deletion resulted in reduced muscle fiber size. In experiments using C2C12 cells, vitamin D suppressed the expression of cathepsin L and MAFbx (Endo et al., 2003; Hirose et al., 2018). We have summarized the protective effects of drugs in the process of catabolism during denervated muscle atrophy in Fig. 1.
Figure 1: Overview of the effects of drugs at different stages of denervated skeletal muscle atrophy.
The Effect of Drugs on the Protein Synthesis Pathway
Possibly through activation of the adenosine monophosphate-activated protein kinase pathway, Royal jelly, comprising water, protein, sugar, and lipids, can avert the reduction in skeletal muscle fiber diameter following denervation. Treatment of C2C12 myoblasts with Royal jelly promoted differentiation and proliferation (Shirakawa et al., 2020). In the process of denervated skeletal muscle atrophy, the Notch signaling pathway is activated early after denervation and subsequently declined. Meanwhile, the effect of nandrolone on the Notch signaling ensues following nerve transection, which occurs earlier than the protective effect that prevents continued muscle loss (Liu et al., 2011). In denervated mouse models, dietary intake of 8-prenylnaringenin triggers Akt phosphorylation, thereby potentially alleviating skeletal muscle atrophy (Mukai et al., 2012).
The Effect of Drugs on Other Pathways
Due to its binding affinity to estrogen receptors (ERs), several physiological functions of genistein are attributed to its estrogenic activity (Kuiper et al., 1998). ERs are present in the skeletal muscle, and ER subtypes ER-α and ER-β have a vital role in the differentiation and inflammation of myoblasts (Ogawa et al., 2011; Velders et al., 2012). The intake of genistein exhibits a protective effect on muscle loss caused by innervation. ER-α is activated during soleus muscle atrophy. Therefore, it may be presumed that genistein targets ER-α, thereby exerting a protective effect against muscle atrophy (Aoyama et al., 2016). Nandrolone enhances strength and functional recovery in re-innervated muscles post-establishment of denervation atrophy (Isaacs et al., 2013). Studies have confirmed the efficacy of growth hormone in treating denervated skeletal muscle atrophy. In cases of peripheral nerve injury, Tuffaha et al. (2016) unveiled that growth hormone therapy could expedite axon regeneration in male rats, foster axon myelination, diminish muscle atrophy, and amplify muscle innervation. Nonetheless, further research is crucial to unveil the precise molecular mechanism (Tuffaha et al., 2016). Bortezomib retards the atrophy of rat thyroid muscle and posterior circular ganglion muscles caused by innervation, though the specific mechanism has not been clarified (Sei et al., 2015). Finally, we summarized the pharmacological protective effects mentioned in the review on denervated skeletal muscle atrophy and present them in Table 1.
Comprehending the intricate pathophysiological course of denervated skeletal muscle atrophy is significant in uncovering more drugs or methodologies to combat this condition. In this review, we introduced the proposition from the previous phase: denervated skeletal muscle atrophy can be categorized into four stages as a foundation and summarized various drugs reported as effective for addressing denervated skeletal muscle atrophy across different stages. Our prior investigations also highlighted the pivotal roles of salidroside, isoquercitrin, and PQQ in alleviating denervated skeletal muscle atrophy by modulating distinct signaling pathways or molecules. However, comprehensive research is imperative to ascertain the ongoing efficacy of these drugs.
Acknowledgement: The authors thank Professor Jian Xu and Rongrong Chen from Affiliated Maternity and Child Health Care Hospital of Nantong University for their valuable suggestions for this review.
Funding Statement: The present study was supported by the National Natural Science Foundation of China (Grant No. 32200940), Science and Technology Bureau of Nantong (Grant Nos. JC2020101, JC2021085), and Municipal Health Commission of Nantong (Grant No. MA2020019).
Author Contributions: The authors confirm contribution to the paper as follows: study conception and design: JIAYING QIU, YAN CHANG, HAIBO ZHANG, ZHENYU ZHANG; data collection: HUI XU, WANQING XU, QINGWEN ZHU; analysis and interpretation of results: JIAYING QIU, WENPENG LIANG, MENGSI LIN; draft manuscript preparation: JIAYING QIU, ZHENYU ZHANG; All authors reviewed the results and approved the final version of the manuscript.
Availability of Data and Materials: The research results highlighted in this review are from publicly available papers.
Ethics Approval: Not applicable.
Conflicts of Interest: The authors declare that they have no conflicts of interest to report regarding the present review.
References
Adhihetty PJ, O’Leary MF, Chabi B, Wicks KL, Hood DA (2007). Effect of denervation on mitochondrially mediated apoptosis in skeletal muscle. Journal of Applied Physiology 102: 1143–1151. [Google Scholar] [PubMed]
Aoyama S, Jia H, Nakazawa K, Yamamura J, Saito K, Kato H (2016). Dietary genistein prevents denervation-induced muscle atrophy in male rodents via effects on estrogen receptor-α. The Journal of Nutrition 146: 1147–1154. [Google Scholar] [PubMed]
Asami Y, Aizawa M, Kinoshita M, Ishikawa J, Sakuma K (2018). Resveratrol attenuates denervation-induced muscle atrophy due to the blockade of atrogin-1 and p62 accumulation. International Journal of Medical Sciences 15: 628–637. [Google Scholar] [PubMed]
Bodine SC, Latres E, Baumhueter S, Lai VK, Nunez L et al. (2001). Identification of ubiquitin ligases required for skeletal muscle atrophy. Science 294: 1704–1708. [Google Scholar] [PubMed]
Bohorov O, Buttery PJ, Correia JH, Soar JB (1987). The effect of the beta-2-adrenergic agonist clenbuterol or implantation with oestradiol plus trenbolone acetate on protein metabolism in wether lambs. The British Journal of Nutrition 57: 99–107. [Google Scholar] [PubMed]
Castets P, Rion N, Theodore M, Falcetta D, Lin S et al. (2019). mTORC1 and PKB/Akt control the muscle response to denervation by regulating autophagy and HDAC4. Nature Communications 10: 3187. [Google Scholar] [PubMed]
Castillero E, Martin AI, Lopez-Menduina M, Villanua MA, Lopez-Calderon A (2009). Eicosapentaenoic acid attenuates arthritis-induced muscle wasting acting on atrogin-1 and on myogenic regulatory factors. American Journal of Physiology Regulatory, Integrative and Comparative Physiology 297: R1322–1331. [Google Scholar] [PubMed]
Choi WH, Jang YJ, Son HJ, Ahn J, Jung CH, Ha TY (2018). Apigenin inhibits sciatic nerve denervation-induced muscle atrophy. Muscle & Nerve 58: 314–318. [Google Scholar]
Cui W, Liu CX, Zhang YC, Shen Q, Feng ZH, Wang J, Lu SF, Wu J, Li JX (2019). A novel oleanolic acid derivative HA-19 ameliorates muscle atrophy via promoting protein synthesis and preventing protein degradation. Toxicology and Applied Pharmacology 378: 114625. [Google Scholar] [PubMed]
Dai J, Xiang Y, Fu D, Xu L, Jiang J, Xu J (2020). Ficus carica L. attenuates denervated skeletal muscle atrophy via PPARalpha/NF-kappaB pathway. Frontiers in Physiology 11: 580223. [Google Scholar] [PubMed]
Demiryurek S, Babul A (2004). Effects of vitamin E and electrical stimulation on the denervated rat gastrocnemius muscle malondialdehyde and glutathione levels. The International Journal of Neuroscience 114: 45–54. [Google Scholar] [PubMed]
Dow DE, Dennis RG, Faulkner JA (2005). Electrical stimulation attenuates denervation and age-related atrophy in extensor digitorum longus muscles of old rats. The Journals of Gerontology: Series A 60: 416–424. [Google Scholar]
Dumitru A, Radu BM, Radu M, Cretoiu SM (2018). Muscle changes during atrophy. Advances in Experimental Medicine and Biology 1088: 73–92. [Google Scholar] [PubMed]
Endo I, Inoue D, Mitsui T, Umaki Y, Akaike M, Yoshizawa T, Kato S, Matsumoto T (2003). Deletion of vitamin D receptor gene in mice results in abnormal skeletal muscle development with deregulated expression of myoregulatory transcription factors. Endocrinology 144: 5138–5144. [Google Scholar] [PubMed]
Fujiwara Y, Tsukahara C, Ikeda N, Sone Y, Ishikawa T, Ichi I, Koike T, Aoki Y (2017). Oleuropein improves insulin resistance in skeletal muscle by promoting the translocation of GLUT4. Journal of Clinical Biochemistry and Nutrition 61: 196–202. [Google Scholar] [PubMed]
Gifondorwa DJ, Jimenz-Moreno R, Hayes CD, Rouhani H, Robinson MB, Strupe JL, Caress J, Milligan C (2012). Administration of recombinant heat shock protein 70 delays peripheral muscle denervation in the SOD1(G93A) mouse model of amyotrophic lateral sclerosis. Neurology Research International 2012: 170426. [Google Scholar] [PubMed]
Goncalves DA, Silveira WA, Lira EC, Graca FA, Paula-Gomes S, Zanon NM, Kettelhut IC, Navegantes LC (2012). Clenbuterol suppresses proteasomal and lysosomal proteolysis and atrophy-related genes in denervated rat soleus muscles independently of Akt. American Journal of Physiology Endocrinology and Metabolism 302: E123–133. [Google Scholar] [PubMed]
Gu X, Ding F, Yang Y, Liu J (2011). Construction of tissue engineered nerve grafts and their application in peripheral nerve regeneration. Progress in Neurobiology 93: 204–230. [Google Scholar] [PubMed]
He Q, Qiu J, Dai M, Fang Q, Sun X, Gong Y, Ding F, Sun H (2016). MicroRNA-351 inhibits denervation-induced muscle atrophy by targeting TRAF6. Experimental and Therapeutic Medicine 12: 4029–4034. [Google Scholar] [PubMed]
Hiramoto S, Yahata N, Saitoh K, Yoshimura T, Wang Y, Taniyama S, Nikawa T, Tachibana K, Hirasaka K (2018). Dietary supplementation with alkylresorcinols prevents muscle atrophy through a shift of energy supply. The Journal of Nutritional Biochemistry 61: 147–154. [Google Scholar] [PubMed]
Hirose Y, Onishi T, Miura S, Hatazawa Y, Kamei Y (2018). Vitamin D attenuates FOXO1-target atrophy gene expression in C2C12 muscle cells. Journal of Nutritional Science and Vitaminology 64: 229–232. [Google Scholar] [PubMed]
Hitachi K, Nakatani M, Tsuchida K (2019). Long non-coding RNA myoparr regulates GDF5 expression in denervated mouse skeletal muscle. Non-coding RNA 5: 33. [Google Scholar]
Huang Z, Fang Q, Ma W, Zhang Q, Qiu J, Gu X, Yang H, Sun H (2019). Skeletal muscle atrophy was alleviated by salidroside through suppressing oxidative stress and inflammation during denervation. Frontiers in Pharmacology 10: 997. [Google Scholar] [PubMed]
Isaacs J, Feher J, Shall M, Vota S, Fox MA, Mallu S, Razavi A, Friebe I, Shah S, Spita N (2013). Effects of nandrolone on recovery after neurotization of chronically denervated muscle in a rat model. Journal of Neurosurgery 119: 914–923. [Google Scholar] [PubMed]
Jackman RW, Kandarian SC (2004). The molecular basis of skeletal muscle atrophy. American Journal of Physiology Cell Physiology 287: C834–843. [Google Scholar] [PubMed]
Janice Sanchez B, Tremblay AK, Leduc-Gaudet JP, Hall DT, Kovacs E et al. (2019). Depletion of HuR in murine skeletal muscle enhances exercise endurance and prevents cancer-induced muscle atrophy. Nature Communications 10: 4171. [Google Scholar] [PubMed]
Khal J, Tisdale MJ (2008). Downregulation of muscle protein degradation in sepsis by eicosapentaenoic acid (EPA). Biochemical and Biophysical Research Communications 375: 238–240. [Google Scholar] [PubMed]
Kim Y, Kim CS, Joe Y, Chung HT, Ha TY, Yu R (2018). Quercetin reduces tumor necrosis factor alpha-induced muscle atrophy by upregulation of heme oxygenase-1. Journal of Medicinal Food 21: 551–559. [Google Scholar] [PubMed]
Komiya Y, Kobayashi C, Uchida N, Otsu S, Tanio T, Yokoyama I, Nagasao J, Arihara K (2019). Effect of dietary fish oil intake on ubiquitin ligase expression during muscle atrophy induced by sciatic nerve denervation in mice. Animal Science Journal 90: 1018–1025. [Google Scholar] [PubMed]
Kris-Etherton PM, Hecker KD, Bonanome A, Coval SM, Binkoski AE, Hilpert KF, Griel AE, Etherton TD (2002). Bioactive compounds in foods: Their role in the prevention of cardiovascular disease and cancer. The American Journal of Medicine 113: 71S–88S. [Google Scholar] [PubMed]
Kuiper GG, Lemmen JG, Carlsson B, Corton JC, Safe SH, van der Saag PT, van der Burg B, Gustafsson JA (1998). Interaction of estrogenic chemicals and phytoestrogens with estrogen receptor β. Endocrinology 139: 4252–4263. [Google Scholar] [PubMed]
Kuo YT, Shih PH, Kao SH, Yeh GC, Lee HM (2015). Pyrroloquinoline quinone resists denervation-induced skeletal muscle atrophy by activating PGC-1alpha and integrating mitochondrial electron transport chain complexes. PLoS One 10: e0143600. [Google Scholar] [PubMed]
Lala-Tabbert N, Lejmi-Mrad R, Timusk K, Fukano M, Holbrook J, St-Jean M, LaCasse EC, Korneluk RG (2019). Targeted ablation of the cellular inhibitor of apoptosis 1 (cIAP1) attenuates denervation-induced skeletal muscle atrophy. Skeletal Muscle 9: 13. [Google Scholar] [PubMed]
Lang F, Aravamudhan S, Nolte H, Turk C, Holper S, Muller S, Gunther S, Blaauw B, Braun T, Kruger M (2017). Dynamic changes in the mouse skeletal muscle proteome during denervation-induced atrophy. Disease Models & Mechanisms 10: 881–896. [Google Scholar]
Lee JH, Zhou HY, Cho SY, Kim YS, Lee YS, Jeong CS (2007). Anti-inflammatory mechanisms of apigenin: Inhibition of cyclooxygenase-2 expression, adhesion of monocytes to human umbilical vein endothelial cells, and expression of cellular adhesion molecules. Archives of Pharmacal Research 30: 1318–1327. [Google Scholar] [PubMed]
Li J, Chan MC, Yu Y, Bei Y, Chen P et al. (2017). miR-29b contributes to multiple types of muscle atrophy. Nature Communications 8: 15201. [Google Scholar] [PubMed]
Li YP, Chen Y, John J, Moylan J, Jin B, Mann DL, Reid MB (2005). TNF-alpha acts via p38 MAPK to stimulate expression of the ubiquitin ligase atrogin1/MAFbx in skeletal muscle. FASEB Journal 19: 362–370. [Google Scholar] [PubMed]
Liu XH, Yao S, Qiao RF, Levine AC, Kirschenbaum A, Pan J, Wu Y, Qin W, Bauman WA, Cardozo CP (2011). Nandrolone reduces activation of Notch signaling in denervated muscle associated with increased Numb expression. Biochemical and Biophysical Research Communications 414: 165–169. [Google Scholar] [PubMed]
Ma W, Zhang R, Huang Z, Zhang Q, Xie X et al. (2019). PQQ ameliorates skeletal muscle atrophy, mitophagy and fiber type transition induced by denervation via inhibition of the inflammatory signaling pathways. Annals of Translational Medicine 7: 440. [Google Scholar] [PubMed]
Marmolejo-Martinez-Artesero S, Romeo-Guitart D, Manas-Garcia L, Barreiro E, Casas C (2020). NeuroHeal reduces muscle atrophy and modulates associated autophagy. Cells 9: 1575. [Google Scholar] [PubMed]
Miyawaki A, Rojasawasthien T, Hitomi S, Aoki Y, Urata M et al. (2020). Oral administration of geranylgeraniol rescues denervation-induced muscle atrophy via suppression of atrogin-1. In Vivo 34: 2345–2351. [Google Scholar] [PubMed]
Mukai R, Horikawa H, Fujikura Y, Kawamura T, Nemoto H, Nikawa T, Terao J (2012). Prevention of disuse muscle atrophy by dietary ingestion of 8-prenylnaringenin in denervated mice. PLoS One 7: e45048. [Google Scholar] [PubMed]
Mukai R, Nakao R, Yamamoto H, Nikawa T, Takeda E, Terao J (2010). Quercetin prevents unloading-derived disused muscle atrophy by attenuating the induction of ubiquitin ligases in tail-suspension mice. Journal of Natural Products 73: 1708–1710. [Google Scholar] [PubMed]
Muraguchi T, Okamoto K, Mitake M, Ogawa H, Shidoji Y (2011). Polished rice as natural sources of cancer-preventing geranylgeranoic acid. Journal of Clinical Biochemistry and Nutrition 49: 8–15. [Google Scholar] [PubMed]
Ogawa M, Yamaji R, Higashimura Y, Harada N, Ashida H, Nakano Y, Inui H (2011). 17β-estradiol represses myogenic differentiation by increasing ubiquitin-specific peptidase 19 through estrogen receptor α. The Journal of Biological Chemistry 286: 41455–41465. [Google Scholar] [PubMed]
O’Leary MF, Hood DA (2009). Denervation-induced oxidative stress and autophagy signaling in muscle. Autophagy 5: 230–231. [Google Scholar] [PubMed]
Pairet M, Engelmann P, von Nicolai H, Champeroux P, Richard S, Rauber G, Engelhardt G (1997). Ambroxol improves the broncho-spasmolytic activity of clenbuterol in the guinea-pig. The Journal of Pharmacy and Pharmacology 49: 184–186. [Google Scholar] [PubMed]
Pierce A, deWaal E, Van Remmen H, Richardson A, Chaudhuri A (2006). A novel approach for screening the proteome for changes in protein conformation. Biochemistry 45: 3077–3085. [Google Scholar] [PubMed]
Pires-Oliveira M, Maragno AL, Parreiras-e-Silva LT, Chiavegatti T, Gomes MD, Godinho RO (2010). Testosterone represses ubiquitin ligases atrogin-1 and Murf-1 expression in an androgen-sensitive rat skeletal muscle in vivo. Journal of Applied Physiology 108: 266–273. [Google Scholar] [PubMed]
Powers SK, Kavazis AN, DeRuisseau KC (2005). Mechanisms of disuse muscle atrophy: Role of oxidative stress. American Journal of Physiology Regulatory, Integrative and Comparative Physiology 288: R337–344. [Google Scholar] [PubMed]
Qiu J, Fang Q, Xu T, Wu C, Xu L et al. (2018). Mechanistic role of reactive oxygen species and therapeutic potential of antioxidants in denervation-or fasting-induced skeletal muscle atrophy. Frontiers in Physiology 9: 215. [Google Scholar] [PubMed]
Qiu J, Wu L, Chang Y, Sun H, Sun J (2021). Alternative splicing transitions associate with emerging atrophy phenotype during denervation-induced skeletal muscle atrophy. Journal of Cellular Physiology 236: 4496–4514. [Google Scholar] [PubMed]
Reza MM, Subramaniyam N, Sim CM, Ge X, Sathiakumar D, McFarlane C, Sharma M, Kambadur R (2017). Irisin is a pro-myogenic factor that induces skeletal muscle hypertrophy and rescues denervation-induced atrophy. Nature Communications 8: 1104. [Google Scholar] [PubMed]
Schumacher MA, Chinnam N, Ohashi T, Shah RS, Erickson HP (2013). The structure of irisin reveals a novel intersubunit beta-sheet fibronectin type III (FNIII) dimer: Implications for receptor activation. The Journal of Biological Chemistry 288: 33738–33744. [Google Scholar] [PubMed]
Sei H, Taguchi A, Nishida N, Hato N, Gyo K (2015). Preventive effects of bortezomib on denervation-induced atrophy of the intrinsic laryngeal muscles: An experimental study in the rat. Acta Oto-Laryngologica 135: 713–717. [Google Scholar] [PubMed]
Shen Y, Zhang R, Xu L, Wan Q, Zhu J et al. (2019). Microarray analysis of gene expression provides new insights into denervation-induced skeletal muscle atrophy. Frontiers in Physiology 10: 1298. [Google Scholar] [PubMed]
Shiota C, Abe T, Kawai N, Ohno A, Teshima-Kondo S, Mori H, Terao J, Tanaka E, Nikawa T (2015). Flavones inhibit LPS-induced atrogin-1/MAFbx expression in mouse C2C12 skeletal myotubes. Journal of Nutritional Science and Vitaminology 61: 188–194. [Google Scholar] [PubMed]
Shirakawa T, Miyawaki A, Matsubara T, Okumura N, Okamoto H et al. (2020). Daily oral administration of protease-treated royal jelly protects against denervation-induced skeletal muscle atrophy. Nutrients 12: 3089. [Google Scholar] [PubMed]
Straughn AR, Kakar SS (2019). Withaferin A ameliorates ovarian cancer-Induced cachexia and proinflammatory signaling. Journal of Ovarian Research 12: 115. [Google Scholar] [PubMed]
Szychlinska MA, Castrogiovanni P, Trovato FM, Nsir H, Zarrouk M, Lo Furno D, di Rosa M, Imbesi R, Musumeci G (2019). Physical activity and Mediterranean diet based on olive tree phenolic compounds from two different geographical areas have protective effects on early osteoarthritis, muscle atrophy and hepatic steatosis. European Journal of Nutrition 58: 565–581. [Google Scholar] [PubMed]
Tabata S, Aizawa M, Kinoshita M, Ito Y, Kawamura Y, Takebe M, Pan W, Sakuma K (2019). The influence of isoflavone for denervation-induced muscle atrophy. European Journal of Nutrition 58: 291–300. [Google Scholar] [PubMed]
Takisawa S, Funakoshi T, Yatsu T, Nagata K, Aigaki T, Machida S, Ishigami A (2019). Vitamin C deficiency causes muscle atrophy and a deterioration in physical performance. Scientific Reports 9: 4702. [Google Scholar] [PubMed]
Tamaki H, Yotani K, Ogita F, Hayao K, Nakagawa K, Sugawara K, Kirimoto H, Onishi H, Kasuga N, Yamamoto N (2017). Electrical stimulation of denervated rat skeletal muscle ameliorates bone fragility and muscle loss in early-stage disuse musculoskeletal atrophy. Calcified Tissue International 100: 420–430. [Google Scholar] [PubMed]
Tuffaha SH, Budihardjo JD, Sarhane KA, Khusheim M, Song D et al. (2016). Growth hormone therapy accelerates axonal regeneration, promotes motor reinnervation, and reduces muscle atrophy following peripheral nerve injury. Plastic and Reconstructive Surgery 137: 1771–1780. [Google Scholar] [PubMed]
Velders M, Schleipen B, Fritzemeier KH, Zierau O, Diel P (2012). Selective estrogen receptor-β activation stimulates skeletal muscle growth and regeneration. FASEB Journal 26: 1909–1920. [Google Scholar] [PubMed]
Wang J, Gao X, Ren D, Zhang M, Zhang P et al. (2020). Triptolide induces atrophy of myotubes by triggering IRS-1 degradation and activating the FOXO3 pathway. Toxicology in Vitro 65: 104793. [Google Scholar] [PubMed]
Whitehouse AS, Smith HJ, Drake JL, Tisdale MJ (2001). Mechanism of attenuation of skeletal muscle protein catabolism in cancer cachexia by eicosapentaenoic acid. Cancer Research 61: 3604–3609. [Google Scholar] [PubMed]
Whitehouse AS, Tisdale MJ (2001). Downregulation of ubiquitin-dependent proteolysis by eicosapentaenoic acid in acute starvation. Biochemical and Biophysical Research Communications 285: 598–602. [Google Scholar] [PubMed]
Wu C, Tang L, Ni X, Xu T, Fang Q, Xu L, Ma W, Yang X, Sun H (2019). Salidroside attenuates denervation-induced skeletal muscle atrophy through negative regulation of pro-inflammatory cytokine. Frontiers in Physiology 10: 665. [Google Scholar] [PubMed]
Yadav A, Yadav SS, Singh S, Dabur R (2022). Natural products: Potential therapeutic agents to prevent skeletal muscle atrophy. European Journal of Pharmacology 925: 174995. [Google Scholar] [PubMed]
You JS, Park MN, Song W, Lee YS (2010). Dietary fish oil alleviates soleus atrophy during immobilization in association with Akt signaling to p70s6k and E3 ubiquitin ligases in rats. Applied physiology, Nutrition, and Metabolism 35: 310–318. [Google Scholar] [PubMed]
Zhao J, Zhang Y, Zhao W, Wu Y, Pan J, Bauman WA, Cardozo C (2008). Effects of nandrolone on denervation atrophy depend upon time after nerve transection. Muscle & Nerve 37: 42–49. [Google Scholar]
Zhou L, Huang YF, Xie H, Mei XY, Cao J (2020). Herbal complex ‘Buyang Huanwu Tang’ improves motor endplate function of denervated-dependent skeletal muscle atrophy in rat. Journal of Integrative Neuroscience 19: 89–99. [Google Scholar] [PubMed]
Zhu X, Wu C, Qiu S, Yuan X, Li L (2017). Effects of resveratrol on glucose control and insulin sensitivity in subjects with type 2 diabetes: Systematic review and meta-analysis. Nutrition & Metabolism 14: 60. [Google Scholar]
Cite This Article
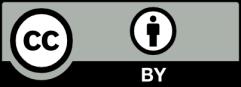
This work is licensed under a Creative Commons Attribution 4.0 International License , which permits unrestricted use, distribution, and reproduction in any medium, provided the original work is properly cited.