Open Access
ARTICLE
Structure, function, and mechanism of the TNFAIP8 (TIPE) family of proteins in cancer and inflammation
1 School of Pharmacy & Clinical Pharmacy, School of Integrative Pharmacy, Guangdong Pharmaceutical University, Guangzhou, 510006, China
2 Department of Biomedical Sciences Laboratory, Affiliated Dongyang Hospital of Wenzhou Medical University, Dongyang, 322100, China
3 The Second People’s Hospital of China Three Gorges University, Yichang, 443000, China
4 Guangdong Province Key Laboratory for Biotechnology Drug Candidates, Guangdong Pharmaceutical University, Guangzhou, 510006, China
5 Key Specialty of Clinical Pharmacy, The First Affiliated Hospital of Guangdong Pharmaceutical University, Guangzhou, 510080, China
* Corresponding Authors: Song Liu, ; Huiqun Tian,
# These authors contributed equally to this article
(This article belongs to the Special Issue: Frontiers in cancer: tumor microenvironment)
BIOCELL 2023, 47(10), 2217-2232. https://doi.org/10.32604/biocell.2023.030233
Received 28 March 2023; Accepted 28 June 2023; Issue published 08 November 2023
Abstract
The multiple roles of the tumor necrosis factor (TNF)-α-inducible protein 8 (TNFAIP8), also named TIPE family of proteins have been shown in tumor and inflammation progression and regulation of cellular autophagy and apoptosis. In this review, we found that the TIPE family showed highly homologous sequences and conserved functional domains, such as the death effector domain (DED)-like domain but displayed different roles and mechanisms in different biological activities. For example, while TIPE is primarily associated with tumor progression and antitumor drug resistance, TIPE1 suppresses tumor progression in most instances. TIPE2 has multiple roles in tumor progression regulation, and antitumor drug resistance. Moreover, TIPE2 was also involved in inflammatory response regulation, tumor typing, and staging. A few studies reported that TIPE3 was engaged in tumor development by activating the phosphatidylinositol-3-kinase (PI3K)/protein kinase B (AKT) signaling pathway. The structure, function, and mechanism of the TIPE family in cancer and inflammation have been summarized in this review. This might serve as a reference for further research on the TIPE family and shed new light on the crosstalk among antitumor responses, inflammation, and immunology.Graphic Abstract

Keywords
Cancer usually progresses in multi-steps and is related to various etiologic factors (Jemal et al., 2010). Most cancers are associated with somatic mutations and environmental factors, like cellular chronic inflammation (Coussens et al., 2013). Chronic inflammation has been linked to cancer development at different levels, including cellular transformation, promotion, survival, proliferation, invasion, angiogenesis, and metastasis (Wu et al., 2014). For example, mutagen-induced chronic inflammation can lead to sustained tissue damage and cell proliferation. Take the example of peroxynitrite, a DNA mutagenic agent which reacts with cellular DNA and causes its mutations, leading to the synthesis of tumor necrosis factor-alpha (TNF-α) and macrophage migration inhibitory factor (Pollard, 2004). The migration inhibitory factor contributes to tumorigenesis by impairing p53-dependent protective responses and interfering with the retinoblastoma (Rb)-adenoviral early region 2 binding factor (E2F) pathway (Singh et al., 2019).
Moreover, abnormal activation of inflammatory cells such as tumor-associated macrophages and dendritic cells, in the tumor microenvironment contributes to tumor cell proliferation and ineffective responses to tumor antigens (Salmaninejad et al., 2019). Further, pro-inflammatory cytokines and chemokines produced by tumor cells or tumor-associated immune cells, including TNF, interleukin-1 (IL-1), IL-6, and vascular endothelial growth factor (VEGF) have been reported to facilitate the antitumor immune response suppression and promote tumor development (Atretkhany et al., 2016). In addition, the balance between apoptosis and proliferation of tumor cells is disturbed throughout the process of tumorigenesis. Multiple signal pathways have been implicated to be involved, such as the Wingless-Type MMTV Integration Site Family (Wnt) signal pathway, the janus kinase (JNK) signal pathway, the phosphatidylinositol-3-kinase (PI3K) signal pathway, and the Hippo signaling pathway (Vendramini-Costa and Carvalho, 2012). For example, the Wnt signaling pathway is often dysregulated in human cancers. It has three branches, the Wnt/β-catenin signaling pathway, the planar cell polarity (Wnt-PCP) pathway, and the Wnt-Ca2+ signaling pathway. An aberrant Wnt signaling pathway promotes cancer stem cell renewal, cell proliferation, and differentiation, thus playing a key role in tumorigenesis, and treatment response (Zhao et al., 2022). The JNK signaling pathway mediates a wide range of cellular processes, including cell proliferation, survival, migration, cell apoptosis, senescence, and stress responses. As members of the mitogen-activated protein kinase (MAPK) family, c-Jun N-terminal Kinases (JNKs) have been identified as key disease drivers in some pathophysiological settings and central oncogenic signaling nodes in various cancers, for positively regulating cancer stem cell populations, promoting invasion and facilitating metastatic outgrowth (Latham et al., 2022). The phosphatidylinositol-3-kinase (PI3K)/Protein kinase B (AKT) pathway controls hallmarks of cancer, including cell survival, metastasis, and metabolism. It plays essential roles in the tumor environment, functioning in angiogenesis and inflammatory factor recruitment. An aberrantly activated PI3K/AKT signaling pathway can recruit oncogenic signaling proteins, including the serine and threonine kinase Protein kinase B. Once activated, Akt phosphorylates several substrates, such as mammalian target of rapamycin (mTOR), one of the most common downstream effectors of Akt, which integrates many proteins to promote cancer progression (He et al., 2021). The Hippo signaling pathway is associated with cellular mechanical strain, cell polarity/adhesion molecules, other signaling pathways (e.g., G protein-coupled receptor (GPCR), Epidermal growth factor receptor (EGFR), Wingless-Type MMTV Integration Site Family (WNT), Notch, and Transforming Growth Factor-β (TGFβ)/Bone Morphogenetic Protein (BMP)), and cellular metabolic status. As core components of the Hippo signaling pathway, the activation of the Yes-associated protein 1 (YAP) influences cell proliferation and resistance to apoptosis, which eventually leads to cancer development. The transcriptional coactivator with PDZ-binding motif (TAZ) stimulates the epithelial-to-mesenchymal transition (EMT) through transcriptional activation of zinc finger E-box binding homeobox 1/2 (ZEB1/2). Dysregulation of Hippo signaling generally results in aberrant YAP and TAZ transcriptional activity that enhances their oncogenic properties (Bae et al., 2017). Besides, autophagy in tumor cells was upregulated in many cancers, promoting tumor cell survival, proliferation, and metastasis. This was through inhibition of p53 activation, sustaining redox homeostasis, maintenance of essential amino acids levels, and inhibition of antitumor immune responses (Usman et al., 2021). Thus, cancers are linked to many factors including cell bio-activity, chronic inflammation, cell apoptosis, cell proliferation, and cell autophagy. This biological progress was not independent or individual but linked by different molecular hubs, signaling pathways, and crosstalk.
The tumor necrosis factor (TNF)-α-inducible protein 8-like is a recently identified protein family with four members, including the tumor necrosis factor (TNF)-α-inducible protein 8 (TNFAIP8, also called TIPE), tumor necrosis factor-α-inducible protein 8-like 1 (TNFAIP8L1 or TIPE1), tumor necrosis factor-α-inducible protein 8-like 2 (TNFAIP8L2, or TIPE2), and tumor necrosis factor-α-inducible protein 8-like 3 (TNFAIP8L3 or TIPE3). It has been reported that they were involved in cancer progression, inflammatory responses, and other biological processes (Hua et al., 2021). They were regarded as important molecules linked to cancer and inflammation. The TIPE family members are similar in structure but vary significantly in terms of biological functions. For example, TIPE regulates cell proliferation and apoptosis (Niture et al., 2018a). While TIPE1 was involved in cell autophagy (Liu et al., 2020a), TIPE2 was reported to support cellular immunity (Sun et al., 2008). The TIPE3 acts as a transporter of second messenger phosphatidylinositol (Fayngerts et al., 2014). In this work, we reviewed recent progress on the structures, functions, and mechanisms of the TIPE family in cancer and inflammation. Understanding their role and molecular mechanism may shed new insights into initiating, progressing, and hence management of their related cancers and immunological diseases.
Although the TIPE family proteins have different important roles in tumor homeostasis maintenance and autoimmunity, they have shown high amino acid sequence similarity and homologous structures (Figs. 1a and 1b). All TIPEs have a conserved C-terminus and a variable—terminus (Niture et al., 2019). The C-terminus of TIPEs contained a TIPE homology (TH) domain characterized by six/seven α-helix structures (Zhang et al., 2009). The TH homology domain contained a DED-like structure. It was reported that the DED-like domain of TIPE2 showed a mirror image of the classical DED structure and negatively regulated cellular apoptosis (Kumar et al., 2000; Niture et al., 2018a). Besides, TIPE3 has an N-terminal α0 helix connected with α1 via a flexible short hinge motif (Kim et al., 2017). TIPE1 was homologous to other TIPEs with two transcript variants encoding one protein (Zhong et al., 2021). Further, the α1-α6 helices of TIPE3 could be aligned with the helices α1-α6 of TIPE2 (Kim et al., 2017). Unlike other TIPE family members, TIPE3 has a unique 19 amino acids N-terminal (NT) region (Fayngerts et al., 2014). The crystal structures of TIPEs suggest that each TIPE family member contains a large hydrophobic central cavity, to which the phospholipid molecule binds (Fig. 1c) (Kim et al., 2017). The TIPEs have highly similar DED-like and TH domains, but different structures in other regions, which may determine the great differences in their expression and functions. The D-Box motif of TIPE is a significant motif of the TIPE and is involved in cell cycle-related protein degradation (Niture et al., 2018a). According to the NetOGlyc 4.0 Server and Prosite database predictions, T31, T36, T138, and S153 were predicted to be crucial amino acid residues of TIPE (Niture et al., 2018a). According to molecular modeling, His86 in mouse TIPE and Tyr76 in human TIPE could form hydrogen bonds with the phosphate group of phosphatidylethanolamine in the active cavity of TIPE. Therefore, these residues may be crucial for TIPE function (Niture et al., 2018a). Further, it was reported that the Arg75 and Arg91 residues of TIPE2 interacted with the free hydroxyl groups in the TH domain cavity of the TIPE2 protein to bind the phosphoinositides (Antony et al., 2016). Similarly, the Arg181 and Arg197 residues were also predicted to be crucial amino acid residues of TIPE3 that could bind with phosphoinositides (Antony et al., 2016). Additionally, some highly conserved hydrophobic residues in the central cavity of TIPE family proteins may also be crucial, such as Gly97 of human TIPE2 and Thr203 and Phe145 of human TIPE3 (Kim et al., 2017). However, only a few structures of TIPE1 have been reported until now.
Figure 1: The structures of the TIPE family. (a) Schematic diagram of the TIPE family. (b) Cartoon presentation of TIPE homology (TH) domain of TIPEs. A Phosphatidylethanolamine (PE) has been shown in a black stick in the cavity of TIPE. (c) Cartoon and surface diagrams of the TH domain of TIPEs. Hydrophobic sections were represented by red, and hydrophilic ones were represented by blue. Yellow arrows indicate hydrophobic cavities. The PDB Numbers of TIPE, TIPE2, and TIPE3 are as follows: 5jxd, 3f4m, and 4q9v, respectively.
TIPE plays many roles in tumor progression, antitumor drug resistance, antibacterial functions, and anti-inflammation
Like most TIPE family members, TIPE plays different roles in tumor progression and antitumor drug resistance (Fig. 2) (Zhang et al., 2009). It is widely expressed in the gastrointestinal tract, lymphatic system, bone marrow, reproductive system, and mammary gland (Zhang et al., 2009). Normally, the TIPE protein is a regulator of T lymphocytes in the lung mucosa, which is important in preventing bacterial infection (Li et al., 2021a). It has also been reported that TIPE specifically regulates the directionality of lymphocyte migration with little effect on the velocity (Sun et al., 2020), and it could inhibit caspase-induced apoptosis (Sun et al., 2015). Furthermore, TIPE is associated with glucocorticoid-mediated apoptosis in mouse thymocytes and acts as a promoter (Woodward et al., 2010). Much evidence has demonstrated that TIPE can promote tumor cell survival, growth, proliferation, and invasion in cancers (Zhang et al., 2009). For example, TIPE inhibits p53 acetylation and promotes the downregulation of the murine double minute 2 (MDM2)/p53 pathway in non-small cell lung cancer (NSCLC), leading to tumor cell proliferation induction and TIPE overexpression (Xing et al., 2018). As a major effector downstream of the Hippo pathway, YAP can be directly phosphorylated by large tumor suppressor 1 and 2 (LATS1/2). Phosphorylated YAP is always ubiquitinated and degraded in the cytoplasm, thereby inhibiting the tumor cells growth-promoting and anti-apoptotic functions of YAP. The Hippo pathway negatively regulates YAP activity through this phosphorylation cascade. In turn, when the Hippo pathway is inhibited, YAP is transported to the nucleus and binds to transcription factors that promote the expression of target genes (Zhang et al., 2006; Han et al., 2018). TIPE has been shown closely related to the Hippo pathway to promote connective tissue growth factor (CTGF) protein expression in lung cancer cells, leading to the suppression of the phosphorylation of LATS1 and YAP (Dong et al., 2017). Similarly, in hepatocellular carcinoma, TNFAIP8 acts in the same way as in non-small cell lung cancer (NSCLC), primarily by promoting tumor progression through inhibition of the Hippo signal pathway. While TIPE knockdown increased the G1 phase percentage and decreased the S phase percentage, its overexpression increased the S phase percentage and decreased G1 phase cells, accompanied by increased levels of cyclin D1 and cyclin E, with the downregulation of the p27 protein in TIPE-transfected SK-Hep-1 cells. In contrast, TIPE knockdown downregulated cyclin D1 and cyclin E, while the expression of p27 was upregulated. These results demonstrate that the TIPE promotes cell cycle progression at the G1/S transition (Dong et al., 2017). Besides, TIPE was reported to promote xenograft tumor growth by lowering cell apoptosis (Zhang et al., 2009).
Figure 2: TIPE regulates tumor progression through different signaling pathways. TIPE impacted the murine double minute 2 (MDM2)/p53 and Hippo signal pathways to promote tumor cell proliferation, migration, and invasion. Further, TIPE promoted xenograft tumor growth by suppressing tumor cell apoptosis. TIPE promoted tumor cell carcinogenicity, migration, and invasion by regulating the expression of anti-cancer genes and oncogenes, such as matrix metalloproteinase (MMP), vascular endothelial growth factor receptor (VEGFR), connective tissue growth factor (CTGF), and related genes.
Many researchers have suggested that the TIPE promoted tumor cell migration and progress by increasing the expression of metastasis-related molecules or collagen (Zhang et al., 2006). For instance, in lung cancer cell metastasis, inhibition of TIPE expression reduced the expression of matrix metalloproteinase 1 (MMP1) and MMP9 (Zhang et al., 2006). Similarly, TIPE promoted the expression of type I collagen and increased the proliferative capacity and oncogenicity of MDA-MB-435 cells (Zhang et al., 2006). Furthermore, TIPE promoted tumor cell migration by upregulating the expression of vascular endothelial growth factor receptor 2 (VEGFR-2), MMP-1, and MMP-9 (Zhang et al., 2006). Interestingly, TIPE has a pair of homologous autophagy regulatory proteins namely Tnfaip8/Oxi-a and Tnfaip8l1/Oxi-b, which are homologous to dopamine neurons and have opposite effects on mammalian target of rapamycin (mTOR), an autophagy marker. Tnfaip8/Oxi-a activates mTOR and induces autophagy, while Tnfaip8l1/Oxi-b is a novel mTOR inhibitor that inhibits neuronal autophagy (Ha et al., 2014). Additionally, TIPE can upregulate autophagy by increasing the expression of autophagy effectors, including microtubule-associated protein1 light chain 3 (LC3)βI/II, Beclin1, eukaryotic translation initiation factor 4E-binding protein 1 (4EBP1), p62, and sirtuin 1 (SIRT1) (Niture et al., 2018b). In addition, TIPE was reported to promote the migration and proliferation of thyroid cancer cells, but the relevant mechanism has not been clarified and needs further exploration (Yang et al., 2021). To summarize, TIPE may be an important therapeutic target for inhibiting cancer metastasis and progression (Kumar et al., 2004).
In addition to directly regulating tumor metastasis and progression, TIPE can regulate tumor progression by impacting the expression of anti-tumor genes and oncogenes (Day et al., 2017; Li et al., 2020b; Zhou et al., 2021). For example, TIPE knockdown enhanced the expression of anti-cancer genes, including interleukin-24 (IL-24), Latrophilin 2 (LPHN2), FAT atypical cadherin 3(FAT3), erythropoietin-producing hepatocellular carcinoma cell receptor A3 (EPHA3), and fatty acid oxidation-related genes, but decreased the expression of oncogenes, such as nuclear factor of activated T cells 5 (NFAT5), metastasis associated lung adenocarcinoma transcript 1 (MALAT1), and mesenchymal to epithelial transition factor (MET) (Day et al., 2017). Further, knockdown of TIPE by microRNA-138 promoted osteosarcoma cells to die (Zhou et al., 2021). However, lncRNA H19 upregulated TIPE and inhibited p53 expression, and promoted the invasion and migration of triple-negative breast cancer cells (Li et al., 2020b).
Apart from the regulation of tumor progression, much evidence has suggested that TIPE was involved in antitumor drug resistance. For example, TIPE promoted resistance to cisplatin treatment by inhibiting the expression of TNF-Iα in esophageal cancer (Zhang and Yang, 2020). When caspase-8/3 and p38 were knocked down, TIPE promoted cisplatin-induced apoptosis in tumor cells (Shi et al., 2013). Interestingly, TIPE could enhance glycemic metabolism and glycolytic metabolic rearrangement and improve metabolism-related product generation in prostate cancer cells, leading to a range of cellular metabolic changes and ATP generation (Niture et al., 2021). Thus, TIPE could induce antitumor drug resistance by mediating metabolic reactions and playing a carcinogenic role (Zhang et al., 2015c). Other reports have shown that TIPE participated in antitumor drug resistance by inducing autophagy or suppressing apoptosis in tumor cells. For example, TIPE activated the extracellular signal-regulated kinase (ERK) signal pathway by interacting with Ras-related C3 botulinum toxin sub-strate 1 (Rac1) to check tumor cell apoptosis and promote chemoresistance in acute myeloid leukemia (Pang et al., 2020). Furthermore, TIPE was reported to induce antitumor drug resistance and promote tumor cell survival by acting on the autophagy-related gene 3 (ATG3) protein (Niture et al., 2018b).
Moreover, TIPE has dual roles in antibacterial infection or anti-inflammation in addition to tumor progression and regulation of antitumor drug resistance. On one hand, TIPE mediates the lethal effect of bacteria and on the other hand, it exerts an indirect antibacterial effect. For example, in lung mucosa, TIPE selectively affected T lymphocyte subsets that highly expressed Ccr9, Tcf7, and Rag1/2 genes to target bacteria (Li et al., 2021a). Besides, another study has shown that TIPE promoted the expression of chemokines in T cells and determined their migration (Sun et al., 2020). However, TIPE-deficient mice could resist lethal listeria infections, presumably because TIPE enhanced the antibacterial ability of the liver and spleen in these mice (Porturas et al., 2015). In addition, TIPE was reported to act as an important regulator in colitis-associated inflammation (Lou et al., 2022). TIPE activated nuclear factor kappa B (NF-κB) and signal transducer and activator of transcription 3 (STAT3) signal pathways in the intestine, leading to the growth retardation of intestinal epithelial cells, thereby aggravating the chemotherapy-induced colitis (Sun et al., 2015).
TIPE1 normally acts as a suppressor in tumor progression and regulation
TIPE1 (TNFAIP8L1), a recently discovered TIPE family member, is known as a positive regulator in cell apoptosis in many cancers and is different from TIPE (Fig. 3) (Zhang et al., 2015c). Normally, TIPE1, as a regulator of necroptosis, can regulate programmed necroptosis and apoptosis in L929 cells or NIH3T3 cells (Hitomi et al., 2008). TIPE1 acted on multiple signaling pathways, such as the C-Jun N terminal kinase (C-JNK), Bcl2, and mTOR signaling pathways to induce cell apoptosis and inhibited tumor cell proliferation (Ha et al., 2014; Zhang et al., 2015c; Wang et al., 2016b). For example, TIPE1 inhibited JNK and p65 activities by interacting with Rac1, and it promoted apoptosis in primary hepatocellular carcinoma cells (Zhang et al., 2015c). Further, in hematological malignancy, over-expression of TIPE1 increased the expression of pro-apoptotic related Bcl-2 family of proteins, such as Bcl-2, Bax, p53, and Bik, and promoted the tumor cells apoptosis (Wang et al., 2016b). Besides, tuberous sclerosis complex 2 (TSC2) is a negative regulatory factor of mTOR. TIPE1 could downregulate the mTOR expression by upregulating TSC2 expression, ultimately leading to neuronal apoptosis (Ha et al., 2014). Overall, TIPE1 promoted tumor cell apoptosis by acting on different signaling pathways.
Figure 3: TIPE1 usually acts as a suppressor of tumor progression. TIPE1 inhibits tumor progression by mediating tumor cell apoptosis and suppressing tumor cell growth, proliferation, migration, and metastasis. TIPE1 initiates tumor cell apoptosis by acting on multiple signaling pathways, such as C-JNK, Bcl2 signaling pathway, and the mammalian target of rapamycin (mTOR) signaling pathway. TIPE1 mediates tumor initiation and progression suppression by inhibiting monocyte chemotaxis protein-1, matrix metalloproteinase 2 (MMP-2), and MMP-9 expression or decreasing protein kinase phosphorylation levels.
In addition to mediating apoptosis in tumor cells, TIPE1 suppressed tumor progress through different mechanisms. For example, TIPE1 was reported to reduce macrophage infiltration and inhibit osteosarcoma cell growth by downregulating monocyte chemotaxis protein-1 expression (Chen et al., 2019). TIPE1 regulated the ERK signaling pathway and inhibited tumor cell proliferation and migration in breast cancer by decreasing the protein kinase phosphorylation level (Hu et al., 2019). Further, TIPE1 is reportedly involved in cancer metastasis regulation. A report showed that the higher the TIPE1 expression, the lower is the lymphatic metastasis in primary hepatocellular carcinoma cells (Zhang et al., 2015c). Moreover, TIPE1 suppressed gastric cancer cell metastasis by inhibiting MMP-2 and MMP-9 expression (Ha et al., 2014; Wang et al., 2016b; Liu et al., 2018). Additionally, evidence has shown that TIPE1 could promote tumor cell proliferation and enhance their activity. For example, TIPE1 promoted tumor cell proliferation in cervical cancer by inhibiting p53 acetylation (Zhao et al., 2019).
TIPE2 is involved in tumor progression, regulation, antitumor drug resistance, tumor subtyping, and tumor staging
TIPE2 (TNFAIP8L2), like TIPE1 also generally acts as a tumor suppressor by promoting the apoptosis of tumor cells (Gu et al., 2020). Many reports have shown that TIPE2 was involved in regulating tumor progression by activating or suppressing multiple signaling pathways (Fig. 4). Under normal conditions, TIPE2 regulates two signaling pathways, the Ral guanine nucleotide dissociation stimulators (Ras-RalGDS)/Ral guanine nucleotide exchange factors (Ral-GEFs) pathway and the Ral signaling pathway, thereby inhibiting the action of Ras and acting as a tumor suppressor (Wang et al., 2018b). Additionally, TIPE2 acts as an inhibitor of Rac, a GTPase that promotes trailing edge polarization during cell migration (Fayngerts et al., 2017). For example, TIPE2 can suppress tumor cell growth, migration, and invasion by affecting downstream molecules of the Ras-related C3 botulinum toxin sub-strate 1 (Rac1) signal pathway, inhibiting the progress of non-small cell lung cancer (Li et al., 2016). Moreover, TIPE2 inhibited the expression of the downstream genes of the Rac1 signal pathway, such as the MMP-9 and urokinase-type plasminogen activator fibrinogen activator (uPA). This resulted in the suppression cell migration and invasion in hepatocellular carcinoma (Gus-Brautbar et al., 2012). On similar lines, TIPE2 mediated the inhibition of tumor cell invasion in papillary thyroid carcinoma (Zhao et al., 2018).
Figure 4: TIPE2 regulates tumor progression through multiple signaling pathways. TIPE2 suppressed the growth, proliferation, migration, and invasion of different kinds of tumor cells by suppressing the Ras-related C3 botulinum toxin sub-strate 1 (Rac1) signaling pathway, Wnt/β-catenin signaling pathway, transforming growth factor-beta (TGF-β)/Sma and Mad protein 2/3 (Smad2/3) signaling pathway, Protein kinase B (AKT) and extra-cellular signal-regulated protein kinase (ERK)1/2 signaling pathways, or interferon regulatory factor 4 (IRF4) signaling pathway. Other mechanisms include downregulation of F-actin polymerization, and decreasing the expression of vascular endothelial growth factor.
In addition to the Rac1 signal pathway, TIPE2 suppressed tumor progression by regulating the Wnt/β-catenin signaling pathway and its relevant signal pathways. For example, evidence from TIPE2-expressing EC9706 cells and xenograft tumor models have suggested that overexpression of TIPE2 suppressed the expression of β-catenin, c-myc, cyclinD1 in esophageal squamous cell carcinoma, thereby suppressing cancer development by inhibiting the Wnt/β-catenin signaling pathway (Zhu et al., 2018). Interestingly, when TIPE2 was overexpressed in glioma cells, it suppressed the hypoxia-induced activation of the Wnt/β-catenin pathway and was also associated with cyclin D1, c-myc, and epithelial-mesenchymal transition (Li et al., 2016). Moreover, in addition to suppressing the expression of Wnt3a, phospho(p)-β-catenin, and p-glycogen synthase kinase-3β, overexpression of TIPE2 also reduced the expression levels of phospho-Sma and Mad protein 2 (p-Smad2), phospho-Sma and Mad protein 3 (p-Smad3), and transforming growth factor-beta (TGF-β) in rectal adenocarcinoma cells. To summarize, TIPE2 suppressed cellular autophagy and the growth, proliferation, migration, and invasion of rectal adenocarcinoma cells by blocking both Wnt/β-catenin and TGF-β/Smad2/3 signaling pathways (Wu et al., 2019).
TIPE2 also modulates tumor progression through the PI3K/Akt and other related signal pathways. For example, TIPE2 suppressed the expression of β-catenin, cyclin D1 and c-myc in breast cancer cells by reducing the expression of p38 and phosphorylated Akt to significantly inhibit cancer cell invasion and migration (Lu et al., 2016; Zhang et al., 2016). Furthermore, TIPE2 inhibited tumor cell proliferation and growth by binding to β-catenin and reducing its nuclear translocation in endometrial cancer (Liu et al., 2020b). Similarly, overexpression of TIPE2 blocked the PI3K/Akt signaling pathway and suppressed the growth, proliferation, migration, invasion, and epithelial-mesenchymal transition (EMT) of prostate cancer cells (Lu et al., 2016). Moreover, TIPE2 was reported to be involved in cell apoptosis by blocking the Akt and extra-cellular signal-regulated protein kinase (ERK)1/2 signaling pathways in gastric cancer (Zhu et al., 2016). Additionally, there is evidence that TIPE2 suppressed the proliferation and growth of gastric cancer cells by modulating the activity of N-Ras and p27 in the interferon regulatory factor 4 (IRF4) signaling pathway (Zhao, 2020). Further, TIPE2 inhibited tumor cell aggregation and angiogenesis by downregulating F-actin polymerization and vascular endothelial growth factor expression (Li et al., 2016).
In addition to regulating tumor progression, TIPE2 has been reportedly involved in antitumor drug resistance. For example, TIPE2 blocked multi-drug resistance1 gene (MDR1) transcription and enhanced patient sensitivity to the cisplatin-mediated treatment in osteosarcoma by inhibiting the transforming growth factor-β-activated kinase 1 (Tak1/NF-κB) and activating protein-1 (AP-1) signaling pathways (Zhao et al., 2018). Besides, TIPE2 was reported to induce cellular autophagy and reduce cisplatin-mediated drug resistance in lung cancer cells by regulating the mTOR signaling pathway (Guo et al., 2020).
Much evidence has suggested that TIPE2 is correlated with tumor staging and subtyping. For example, it has been documented that TIPE2 was barely expressed in hepatocellular carcinoma (Gus-Brautbar et al., 2012), non-small cell lung cancer (Liu et al., 2016), esophageal squamous cell carcinoma (Zhu et al., 2018), oral tongue squamous cell carcinoma (Zhao, 2020), and gastric cancer (Zhao et al., 2015). However, TIPE2 was highly expressed in colon cancer tissues and non-Hodgkin’s lymphoma (NHL) (Zhang et al., 2013). Many reports have shown that the expression of TIPE2 was positively correlated with the Tumor Node Metastasis (TNM) staging in renal cell carcinoma and lymph node metastasis of colon cancer (Zhang et al., 2013). Further, a high expression of TIPE2 was negatively correlated with tumor size, envelope infiltration, peripheral infiltration, and tumor T-stage in thyroid adenocarcinoma (Jia et al., 2018). TIPE2 was more expressed in early-stage tumor tissues than in advanced-stage tumor tissues in pancreatic ductal adenocarcinoma (PDAC) (Sun et al., 2021). The expression was negatively correlated with the TNM stage and lymph node metastasis of PDAC (Sun et al., 2021). TIPE2 was upregulated in the normal columnar epithelium and squamous epithelium of early-stage tumor tissues but downregulated in advanced NSCLC tumor tissues with lymph node metastasis (Li et al., 2016). Moreover, as a biomarker correlating with tumor staging and subtyping, TIPE2 showed different carcinogenic effects on tumor staging and subtyping (Li et al., 2016). For example, in diffuse large B-cell lymphoma (DLBCL) and peripheral T-cell lymphoma, TIPE2 was more expressed in germinal-center B-cell (GCB) phenotypes than non-GCB phenotypes, suggesting that TIPE2 may act as an oncogenic gene in the progression of these tumors (Hao et al., 2016). Besides, TIPE2 expression patterns were different in different lung cancer tissue subtypes. For example, TIPE2 showed low expression in squamous and small-cell lung cancer but was strongly expressed in lung adenocarcinoma (Zhao, 2020). In addition, recent research on oral tongue squamous cell carcinoma (OTSCC) patients showed that high expression of TIPE2 inhibited the progression of OTSCC and was correlated with patient prognosis in terms of the TNM stage and degree of tumor differentiation (Zhao, 2020). To summarize, TIPE2 is important in cancer progression, staging, and classification.
TIPE2 acts as a negative regulator in inflammation and immune-related diseases
TIPE2 has been reported to be expressed in different cells, such as neurons, hepatocytes, colon cells, stomach gland cells, ureter cells, and bladder transitional epithelium (Hao et al., 2016). It normally acts as a negative regulator in innate and adaptive immunity and is highly expressed in our immune systems, such as lymphoid tissues and bone marrow (Zhang et al., 2011). Much evidence has demonstrated that the absence of TIPE2 could induce immune dysregulation and inflammation to cause damage, such as multi-organ inflammation, splenomegaly, and premature death (Sun et al., 2008). For example, the expression of TIPE2 was much higher in acute-on-chronic hepatitis B liver failure (ACHBLF) patients than that in chronic hepatitis B and normal subjects. This suggested that TIPE2 may serve as a predictor for disease onset and progression in ACHBLF patients (Wang et al., 2014). TIPE2 was also reported to reduce inflammation by inhibiting the nucleotide-binding oligomerization domain protein 2 (NOD2)-induced activation of mitogen-activated protein kinase (MAPK) and NF-κB signaling pathways. This is given that deletion of the NOD2 led to lowered myocardial ischemia-induced injury (Wang et al., 2014). Hence, TIPE2 can be a negative regulator linked to NOD2 and inflammatory responses (Zhang et al., 2015a). Moreover, TIPE2 was reported to be involved in the pathogenesis of patients with different types of asthma like eosinophilic asthma (EA), neutrophilic asthma (NA), mixed granulocytic asthma (MA), paucigranulocytic asthma (PA) by regulating the activities and functions of multiple inflammatory cells (Shi et al., 2020).
In addition to being involved in inflammation, TIPE2 is also a negative regulator of immune disorders. For example, TIPE2 has been shown to inhibit the development of Toll-like receptor 4 (TLR4)-mediated autoimmune inflammation in patients with myasthenia gravis (Li et al., 2018). Further, TIPE2 regulated TLR4 receptor-mediated inflammatory response pathways in murine colitis-associated colon cancer by inhibiting caspase-8 activity (Li et al., 2014). Besides, TIPE2 improved the activity of caspases to induce adjuvant arthritic fibroblast-like synoviocyte apoptosis by promoting the expression of death receptor 5 (DR5). Additionally, TIPE2 suppressed the activation of the NF-κB signal pathway in the adjuvant arthritis model (Shi et al., 2016). In systemic lupus erythematosus (SLE), the expression of TIPE2 was suppressed, which increased the expression of interleukins (IL): IL-6, IL-17, IL-21, and aggravated the inflammation (Li et al., 2018). Moreover, recent research has shown that TIPE2 influenced the in vitro biological activity of OTSCCs by negatively regulating the activities of Foxp3+ Treg cells (Zhao, 2020). In addition, overexpression of TIPE2 in the tumor micro-environment enhanced the activity of immune cells, such as the CD8+ cells and natural killer (NK) cells (Zhang et al., 2017). In a recent report, knockout of TIPE2 improved the progression of mature NK cells and elevated the expression of tumor infiltration effectors in NK cells (Bi et al., 2021). Moreover, TIPE2 deletion enhanced the antineoplastic activity and the antitumor function of NK cells (Bi et al., 2023). To summarize, TIPE2 plays important roles in inflammation, immune diseases, and tumor immunology and may be a crosstalk massager linked to inflammation, immune disorders, and tumor progression.
TIPE3 is linked to the PI3K/Akt and related signaling pathways for regulating tumor progression
TIPE3 (TNFAIP8L3) is a novel TIPE family member that was identified recently (Fayngerts et al., 2014). Much evidence has suggested that TIPE3 is highly expressed in human cervical cancer, colon cancer, NSCLC, breast cancer, esophageal cancer, and glioblastoma (Fayngerts et al., 2014; Cui et al., 2015). It was reported that TIPE3 could activate the PI3K/Akt signaling pathway, converting phosphatidylinositol diphosphate (PIP2) to phosphatidylinositol triphosphate (PIP3) for further signaling transduction, and is considered as a second messenger phosphatidyl inositol transporter (Zhang et al., 2017). TIPE3 activated the phosphatidylinositol-3-kinase (PI3K)-mediated signaling pathway and promoted tumor cell growth, proliferation, migration, and invasion by regulating phosphatidylinositol diphosphate (PIP2) and phosphatidylinositol triphosphate (PIP3) (Wang et al., 2018a). However, TIPE3 activated PI3K/Akt signaling and promoted tumor cell migration and invasion in gastric cancer, which is different from the roles of TIPE2 (Gao et al., 2020). Further, TIPE3 has reported to be involved in MMP and urokinase-type plasminogen activator fibrinogen activator (uPA) expression in breast cancer (Lian et al., 2017). TIPE3 promoted breast cancer cell migration by activating the Akt and NF-κB signaling pathways (Moniz and Vanhaesebroeck, 2014). In addition, TIPE3 promoted malignant glioma development by inhibition of p38 phosphorylation (Yuan et al., 2019). Overall, similar to the role of other TIPEs, TIPE3 may also be an oncogenic molecule and associated with PI3K/Akt and related signal pathways in tumor progression.
The TIPE family of proteins is a recently discovered group with numerous roles in regulating immune, inflammation, and tumor development (Table 1). Many studies have focused on TIPE and TIPE2, while few have studied TIPE1 and TIPE3. Although there are some similarities in the structure and expression of the TIPE proteins, they have different biological activities in different cells and tissues (Gu et al., 2020). According to recent studies, there is increasing evidence that TIPE and TIPE3 promote tumor initiation and progression, while TIPE1 and TIPE2 inhibit tumor progression in most cases (Gus-Brautbar et al., 2012; Fayngerts et al., 2014; Zhang et al., 2015c; Day et al., 2017). The differences in the structures of the TIPE proteins may be related to their different roles in tumor progression, which may require further research in the future. Inhibiting or blocking TIPE or TIPE3 has been predicted to inhibit tumor progression and reduce antitumor drug resistance in cancer therapy (Fayngerts et al., 2014; Day et al., 2017). Discovering and designing inhibitors targeting TIPE or TIPE3 in the development of antitumor drugs may be a feasible strategy for antitumor therapy. TIPE1 and TIPE2 have been regarded as tumor suppressors in most studies. Various factors and signaling pathways were involved in the expression regulation of TIPE1 and TIPE2 in different cancers (Gu et al., 2020; Zhang et al., 2021). Further elucidation of the regulatory aspects of TIPE1 and TIPE2 in different cancers may support positive approaches for tumor prevention and treatment.
Interestingly, although TIPE1 and TIPE2 act as tumor suppressors in most cases, there is also some evidence that TIPE1 can act as an oncogenic factor to promote tumor cell growth and proliferation by inhibiting the acetylation of p53 in cervical cancer (Zhao et al., 2019). Moreover, TIPE2 shows low expression in bladder cancer, cervical cancer, and ovarian cancer, and the roles and related mechanisms in these cancers remain unclear (Zhang et al., 2011; Jiang et al., 2022; Zhang et al., 2022). Currently, the functions and mechanisms of TIPE3 have been reported to a lower extent. The roles and mechanisms of the TIPE family in cancer can be deduced as intricate.
In addition to its diverse regulatory roles in cancer, TIPE2 is highly expressed in the immune system, such as lymph and bone marrow, where it is involved in inflammation and immune regulation (Sun et al., 2008). Studying the role and mechanism of TIPE2 in immune and inflammatory diseases may be a future direction for treating immune disorders, such as eczema, psoriasis, rheumatoid arthritis, and chronic hepatitis. At the same time, given the established connection between inflammation and tumors, studies on TIPE2-related signaling pathways may help us further understand the interaction between inflammation and tumor progression. As mentioned earlier, TIPE2 inhibits natural killer cell maturation and further reduces antitumor immunity, which may be a good basis to understand these aspects. Furthermore, developing TIPE2 agonists or inhibitors may be helpful for antitumor and immunological disease therapies.
In summary, the roles and mechanisms of the TIPE family in cancer and inflammatory responses are not yet fully understood. Deeper studies on the structure, function, and mechanism of the TIPE family may help us better understand the relationship among inflammation, immune responses, and tumor progression. This will provide us with more clues and ideas for the treatment and prognosis of tumors or related immune diseases. In addition, as a family with high amino acid sequence similarity (Niture et al., 2018a), whether there exists an interaction between different TIPE members and crosstalk mechanisms among the TIPE family may also be another noteworthy avenue of research.
Acknowledgement: None.
Funding Statement: This research was supported by the Medical Scientific Research Foundation of Guangdong Province of China (No. A2021236), 2022 Guangdong Provincial Education Science Planning Project (Higher Education Special Project, No. 2022GXJK221), the 2021 Open Project Fund of Guangdong Provincial Key Laboratory of Medicinal Functional Gene Research, the National Key Clinical Specialty Construction Project (Clinical Pharmacy) and High-Level Clinical Key Specialty (Clinical Pharmacy) in Guangdong Province, Science and Technology Program of Guangzhou, China (No. 202201010154), the Special Fund for the Cultivation of Scientific and Technological Innovation of College Students in Guangdong Province of China (No. pdjh2022b0270), the College Students’ Innovation and Entrepreneurship Training Project of Guangdong Province (Nos. 202210573054, 202210573041), Special Fund for the Cultivation of National Natural Science Foundation of China in School of Clinical Pharmacy, Guangdong Pharmaceutical University (No. SCP2022-03), Jinghua (Zhejiang Province) Science and Technology Research Program Project (No. 2021-4-135).
Author Contributions: The authors confirm their contribution to the paper as follows: conceptualization, SL, ZL, HT, and LK; writing, original draft preparation: ZL, CT, GL, SL, YW; writing, review and editing, SL, HT, LK; supervision, SL, HT, LK. All authors reviewed the results and approved the final version of the manuscript.
Availability of Data and Materials: Not applicable.
Ethics Approval: Not applicable.
Conflicts of Interest: The authors declare that they have no conflicts of interest to report regarding the present study.
References
Antony P, Baby B, Vijayan R (2016). Molecular insights into the binding of phosphoinositide to the TH domain region of TIPE proteins. Journal of Molecular Modeling 22: 272. https://doi.org/10.1007/s00894-016-3141-7 [Google Scholar] [PubMed] [CrossRef]
Atretkhany KN, Drutskaya MS, Nedospasov SA, Grivennikov SI, Kuprash DV (2016). Chemokines, cytokines and exosomes help tumors to shape inflammatory microenvironment. Pharmacology & Therapeutics 168: 98–112. https://doi.org/10.1016/j.pharmthera.2016.09.011 [Google Scholar] [PubMed] [CrossRef]
Bae JS, Kim SM, Lee H (2017). The Hippo signaling pathway provides novel anti-cancer drug targets. Oncotarget 8: 16084–16098. https://doi.org/10.18632/oncotarget.14306 [Google Scholar] [PubMed] [CrossRef]
Bi J, Cheng C, Zheng C, Huang C, Zheng X, Wan X, Chen YH, Tian Z, Sun H (2021). TIPE2 is a checkpoint of natural killer cell maturation and antitumor immunity. Science Advances 7: eabi6515. https://doi.org/10.1126/sciadv.abi6515 [Google Scholar] [PubMed] [CrossRef]
Bi J, Huang C, Jin X, Zheng C, Huang Y, Zheng X, Tian Z, Sun H (2023). TIPE2 deletion improves the therapeutic potential of adoptively transferred NK cells. Journal for Immunotherapy of Cancer 11: e006002. https://doi.org/10.1136/jitc-2022-006002 [Google Scholar] [PubMed] [CrossRef]
Cao X, Zhang L, Shi Y, Sun Y, Dai S et al. (2013). Human tumor necrosis factor (TNF)-alpha-induced protein 8-like 2 suppresses hepatocellular carcinoma metastasis through inhibiting Rac1. Molecular Cancer 12: 149. https://doi.org/10.1186/1476-4598-12-149 [Google Scholar] [PubMed] [CrossRef]
Chen P, Zhou J, Li J, Zhang Q, Zuo Q (2019). TIPE1 suppresses osteosarcoma tumor growth by regulating macrophage infiltration. Clinical and Translational Oncology 21: 334–341. https://doi.org/10.1007/s12094-018-1927-z [Google Scholar] [PubMed] [CrossRef]
Coussens LM, Zitvogel L, Palucka AK (2013). Neutralizing tumor-promoting chronic inflammation: A magic bullet? Science 339: 286–291. https://doi.org/10.1126/science.1232227 [Google Scholar] [PubMed] [CrossRef]
Cui J, Hao C, Zhang W, Shao J, Zhang N, Zhang G, Liu S (2015). Identical expression profiling of human and murine TIPE3 protein reveals links to its functions. Journal of Histochemistry & Cytochemistry 63: 206–216. https://doi.org/10.1369/0022155414564871 [Google Scholar] [PubMed] [CrossRef]
Cui J, Zhang G, Hao C, Wang Y, Lou Y, Zhang W, Wang J, Liu S (2011). The expression of TIPE1 in murine tissues and human cell lines. Molecular Immunology 48: 1548–1555. https://doi.org/10.1016/j.molimm.2011.04.023 [Google Scholar] [PubMed] [CrossRef]
Day TF, Mewani RR, Starr J, Li X, Chakravarty D et al. (2017). Transcriptome and proteome analyses of TNFAIP8 knockdown cancer cells reveal new insights into molecular determinants of cell survival and tumor progression. Methods in Molecular Biology 1513: 83–100. [Google Scholar] [PubMed]
Dong Q, Fu L, Zhao Y, Xie C, Li Q, Wang E (2017). TNFAIP8 interacts with LATS1 and promotes aggressiveness through regulation of Hippo pathway in hepatocellular carcinoma. Oncotarget 8: 15689–15703. https://doi.org/10.18632/oncotarget.14938 [Google Scholar] [PubMed] [CrossRef]
Duan D, Zhu YQ, Guan LL, Wang J (2014). Upregulation of SCC-S2 in immune cells and tumor tissues of papillary thyroid carcinoma. Tumor Biology 35: 4331–4337. https://doi.org/10.1007/s13277-013-1568-3 [Google Scholar] [PubMed] [CrossRef]
Fayngerts SA, Wang Z, Zamani A, Sun H, Boggs AE et al. (2017). Direction of leukocyte polarization and migration by the phosphoinositide-transfer protein TIPE2. Nature Immunology 18: 1353–1360. https://doi.org/10.1038/ni.3866 [Google Scholar] [PubMed] [CrossRef]
Fayngerts SA, Wu J, Oxley CL, Liu X, Vourekas A et al. (2014). TIPE3 is the transfer protein of lipid second messengers that promote cancer. Cancer Cell 26: 465–478. https://doi.org/10.1016/j.ccr.2014.07.025 [Google Scholar] [PubMed] [CrossRef]
Gao HY, Huo FC, Wang HY, Pei DS (2017). MicroRNA-9 inhibits the gastric cancer cell proliferation by targeting TNFAIP8. Cell Proliferation 50: e12331. https://doi.org/10.1111/cpr.12331 [Google Scholar] [PubMed] [CrossRef]
Gao JF, Zhang H, Lv J, Fan YY, Feng D, Song L (2020). Effects of the long and short isoforms of TIPE3 on the growth and metastasis of gastric cancer. Biomedicine & Pharmacotherapy 124: 109853. https://doi.org/10.1016/j.biopha.2020.109853 [Google Scholar] [PubMed] [CrossRef]
Ge X, Niture S, Lin M, Cagle P, Li PA, Kumar D (2021). MicroRNA-205-5p inhibits skin cancer cell proliferation and increase drug sensitivity by targeting TNFAIP8. Scientific Reports 11: 5660. https://doi.org/10.1038/s41598-021-85097-6 [Google Scholar] [PubMed] [CrossRef]
Gu Z, Cui X, Sun P, Wang X (2020). Regulatory roles of tumor necrosis factor-α-induced protein 8 like-protein 2 in inflammation, immunity and cancers: A review. Cancer Management and Research 12: 12735–12746. [Google Scholar] [PubMed]
Guo H, Ren H, Li J, Hao M, Hao J, Ren H, Guo L, Liu R (2020). TIPE2 suppressed cisplatin resistance by inducing autophagy via mTOR signalling pathway. Experimental and Molecular Pathology 113: 104367. https://doi.org/10.1016/j.yexmp.2020.104367 [Google Scholar] [PubMed] [CrossRef]
Gus-Brautbar Y, Johnson D, Zhang L, Sun H, Wang P, Zhang S, Zhang L, Chen YH (2012). The anti-inflammatory TIPE2 is an inhibitor of the oncogenic Ras. Molecular Cell 45: 610–618. https://doi.org/10.1016/j.molcel.2012.01.006 [Google Scholar] [PubMed] [CrossRef]
Ha JY, Kim JS, Kang YH, Bok E, Kim YS, Son J H (2014). Tnfaip8 l1/Oxi-β binds to FBXW5, increasing autophagy through activation of TSC2 in a Parkinson’s disease model. Journal of Neurochemistry 129: 527–538. https://doi.org/10.1111/jnc.12643 [Google Scholar] [PubMed] [CrossRef]
Hadisaputri YE, Miyazaki T, Suzuki S, Yokobori T, Kobayashi T, Tanaka N, Inose T, Sohda M, Kuwano H (2012). TNFAIP8 overexpression: Clinical relevance to esophageal squamous cell carcinoma. Annals of Surgical Oncology 3: S589–S596. https://doi.org/10.1245/s10434-011-2097-1 [Google Scholar] [PubMed] [CrossRef]
Han Y, Tang Z, Zhao Y, Li Q, Wang E (2018). TNFAIP8 regulates Hippo pathway through interacting with LATS1 to promote cell proliferation and invasion in lung cancer. Molecular Carcinogenesis 57: 159–166. https://doi.org/10.1002/mc.22740 [Google Scholar] [PubMed] [CrossRef]
Hao C, Zhang N, Geng M, Ren Q, Li Y, Wang Y, Chen YH, Liu S (2016). Clinical significance of TIPE2 protein upregulation in non-hodgkin’s lymphoma. Journal of Histochemistry & Cytochemistry 64: 556–564. https://doi.org/10.1369/0022155416662262 [Google Scholar] [PubMed] [CrossRef]
He Y, Sun MM, Zhang GG, Yang J, Chen KS, Xu WW, Li B (2021). Targeting PI3K/Akt signal transduction for cancer therapy. Signal Transduction and Targeted Therapy 6: 425. https://doi.org/10.1038/s41392-021-00828-5 [Google Scholar] [PubMed] [CrossRef]
Hitomi J, Christofferson DE, Ng A, Yao J, Degterev A, Xavier RJ, Yuan J (2008). Identification of a molecular signaling network that regulates a cellular necrotic cell death pathway. Cell 135: 1311–1323. https://doi.org/10.1016/j.cell.2008.10.044 [Google Scholar] [PubMed] [CrossRef]
Sun H, Lou Y, Porturas T, Morrissey S, Luo G et al. (2015). Exacerbated experimental colitis in TNFAIP8-deficient mice. Journal of immunology 194: 5736–5742. https://doi.org/10.4049/jimmunol.1401986 [Google Scholar] [PubMed] [CrossRef]
Hu W, Feng CM, Liu LY, Li N, Tian F, Du JX, Zhao Y, Xiang XX, Liu K, Zhao PQ (2019). TIPE1 inhibits breast cancer proliferation by downregulating ERK phosphorylation and predicts a favorable prognosis. Frontiers in Oncology 9: 400. https://doi.org/10.3389/fonc.2019.00400 [Google Scholar] [PubMed] [CrossRef]
Hua J, Zhuang G, Qi Z (2021). Current research status of TNFAIP8 in tumours and other inflammatory conditions (Review). International Journal of Oncology 59: 46. https://doi.org/10.3892/ijo.2021.5226 [Google Scholar] [PubMed] [CrossRef]
Jemal A, Siegel R, Xu J, Ward E (2010). Cancer statistics, 2010. CA: A Cancer Journal for Clinicians 60: 277–300. https://doi.org/10.3322/caac.20073 [Google Scholar] [PubMed] [CrossRef]
Jia W, Li Z, Chen J, Sun L, Liu C, Wang S, Chi J, Niu J, Lai H (2018). TIPE2 acts as a biomarker for tumor aggressiveness and suppresses cell invasiveness in papillary thyroid cancer (PTC). Cell & Bioscience 8: 49. https://doi.org/10.1186/s13578-018-0247-x [Google Scholar] [PubMed] [CrossRef]
Jiang Y, Zhang Z, Wang X, Feng Z, Hong B, Yu D, Wang Y (2022). A novel prognostic factor TIPE2 in bladder cancer. Pathology and Oncology Research 28: 1610282. https://doi.org/10.3389/pore.2022.1610282 [Google Scholar] [PubMed] [CrossRef]
Kim JS, Park J, Kim MS, Ha JY, Jang YW, Shin DH, Son JH (2017). The Tnfaip8-PE complex is a novel upstream effector in the anti-autophagic action of insulin. Scientific Reports 7: 6248. https://doi.org/10.1038/s41598-017-06576-3 [Google Scholar] [PubMed] [CrossRef]
Kumar D, Gokhale P, Broustas C, Chakravarty D, Ahmad I, Kasid U (2004). Expression of SCC-S2, an antiapoptotic molecule, correlates with enhanced proliferation and tumorigenicity of MDA-MB 435 cells. Oncogene 23: 612–616. https://doi.org/10.1038/sj.onc.1207123 [Google Scholar] [PubMed] [CrossRef]
Kumar D, Whiteside TL, Kasid U (2000). Identification of a novel tumor necrosis factor-α-inducible gene, SCC-S2, containing the consensus sequence of a death effector domain of fas-associated death domain-like interleukin-1beta-converting enzyme-inhibitory protein. Journal of Biological Chemistry 275: 2973–2978. https://doi.org/10.1074/jbc.275.4.2973 [Google Scholar] [PubMed] [CrossRef]
Latham SL, O’Donnell YEI, Croucher DR (2022). Non-kinase targeting of oncogenic c-Jun N-terminal kinase (JNK) signaling: The future of clinically viable cancer treatments. Biochemical Society Transactions 50: 1823–1836. https://doi.org/10.1042/BST20220808 [Google Scholar] [PubMed] [CrossRef]
Li M, Bou-Dargham MJ, Yu J, Etwebi Z, Sun H, Chen YH (2021a). TIPE polarity proteins are required for mucosal deployment of T lymphocytes and mucosal defense against bacterial infection. Molecular Biomedicine 2: 41. https://doi.org/10.1186/s43556-021-00059-8 [Google Scholar] [PubMed] [CrossRef]
Li Z, Cao S, Sun Y, Niu Z, Liu X, Niu J, Zhou Y (2022). TIPE3 is a candidate prognostic biomarker promoting tumor progression via elevating RAC1 in pancreatic cancer. Molecular Cancer 21: 160. https://doi.org/10.1186/s12943-022-01626-5 [Google Scholar] [PubMed] [CrossRef]
Li Z, Guo C, Liu X, Zhou C, Zhu F et al. (2016). TIPE2 suppresses angiogenesis and non-small cell lung cancer (NSCLC) invasiveness via inhibiting Rac1 activation and VEGF expression. Oncotarget 7: 62224–62239. https://doi.org/10.18632/oncotarget.11406 [Google Scholar] [PubMed] [CrossRef]
Li T, Jia L, Deng Y, Wang B, Quan S (2020a). TIPE1 impairs ovarian tumor growth by promoting caspase-dependent apoptosis. Oncology Letters 20: 365. https://doi.org/10.3892/ol.2020.12227 [Google Scholar] [PubMed] [CrossRef]
Li Y, Ma HY, Hu XW, Qu YY, Wen X, Zhang Y, Xu QY (2020b). LncRNA H19 promotes triple-negative breast cancer cells invasion and metastasis through the p53/TNFAIP8 pathway. Cancer Cell International 20: 200. https://doi.org/10.1186/s12935-020-01261-4 [Google Scholar] [PubMed] [CrossRef]
Li XM, Su JR, Yan SP, Cheng ZL, Yang TT, Zhu Q (2014). A novel inflammatory regulator TIPE2 inhibits TLR4-mediated development of colon cancer via caspase-8. Cancer Biomarkers: Section A of Disease Markers 14: 233–240. https://doi.org/10.3233/CBM-140402 [Google Scholar] [PubMed] [CrossRef]
Li T, Wang W, Gong S, Sun H, Zhang H, Yang AG, Chen YH, Li X (2018). Genome-wide analysis reveals TNFAIP8L2 as an immune checkpoint regulator of inflammation and metabolism. Molecular Immunology 99: 154–162. https://doi.org/10.1016/j.molimm.2018.05.007 [Google Scholar] [PubMed] [CrossRef]
Li Q, Yu D, Yu Z, Gao Q, Chen R et al. (2021b). TIPE3 promotes non-small cell lung cancer progression via the protein kinase B/extracellular signal-regulated kinase 1/2-glycogen synthase kinase 3β-β-catenin/Snail axis. Translational Lung Cancer Research 10: 936–954. https://doi.org/10.21037/tlcr-21-147 [Google Scholar] [PubMed] [CrossRef]
Lian K, Ma C, Hao C, Li Y, Zhang N, Chen YH, Liu S (2017). TIPE3 protein promotes breast cancer metastasis through activating AKT and NF-κB signaling pathways. Oncotarget 8: 48889–48904. https://doi.org/10.18632/oncotarget.16522 [Google Scholar] [PubMed] [CrossRef]
Liu W, Chen Y, Xie H, Guo Y, Ren D et al. (2018). TIPE1 suppresses invasion and migration through down-regulating Wnt/β-catenin pathway in gastric cancer. Journal of Cellular and Molecular Medicine 22: 1103–1117. [Google Scholar] [PubMed]
Liu ZJ, Liu HL, Zhou HC, Wang GC (2016). TIPE2 inhibits hypoxia-induced Wnt/β-catenin pathway activation and EMT in glioma cells. Oncology Research 24: 255–261. https://doi.org/10.3727/096504016X14666990347356 [Google Scholar] [PubMed] [CrossRef]
Liu Y, Qi X, Zhao Z, Song D, Wang L, Zhai Q, Zhang X, Zhao P, Xiang X (2020a). TIPE1-mediated autophagy suppression promotes nasopharyngeal carcinoma cell proliferation via the AMPK/mTOR signalling pathway. Journal of Cellular and Molecular Medicine 24: 9135–9144. https://doi.org/10.1111/jcmm.15550 [Google Scholar] [PubMed] [CrossRef]
Liu Y, Wang X, Wan L, Liu X, Yu H et al. (2020b). TIPE2 inhibits the migration and invasion of endometrial cells by targeting β-catenin to reverse epithelial-mesenchymal transition. Human Reproduction 35: 1377–1390. https://doi.org/10.1093/humrep/deaa062 [Google Scholar] [PubMed] [CrossRef]
Lou Y, Tian X, Sun C, Song M, Han M et al. (2022). TNFAIP8 protein functions as a tumor suppressor in inflammation-associated colorectal tumorigenesis. Cell Death & Disease 13: 311. https://doi.org/10.1038/s41419-022-04769-x [Google Scholar] [PubMed] [CrossRef]
Lu Q, Liu Z, Li Z, Chen J, Liao Z, Wu WR, Li YW (2016). TIPE2 overexpression suppresses the proliferation, migration, and invasion in prostate cancer cells by inhibiting PI3K/Akt signaling pathway. Oncology Research 24: 305–313. https://doi.org/10.3727/096504016X14666990347437 [Google Scholar] [PubMed] [CrossRef]
Moniz LS, Vanhaesebroeck B (2014). A new TIPE of phosphoinositide regulator in cancer. Cancer Cell 26: 443–444. https://doi.org/10.1016/j.ccell.2014.09.017 [Google Scholar] [PubMed] [CrossRef]
Niture S, Dong X, Arthur E, Chimeh U, Niture S, Zheng W, Kumar D (2018a). Oncogenic role of tumor necrosis factor α-induced protein 8 (TNFAIP8). Cells 8: 9. https://doi.org/10.3390/cells8010009 [Google Scholar] [PubMed] [CrossRef]
Niture S, Lin M, Odera JO, Moore J, Zhe H, Chen X, Suy S, Collins SP, Kumar D (2021). TNFAIP8 drives metabolic reprogramming to promote prostate cancer cell proliferation. The International Journal of Biochemistry & Cell Biology 130: 105885. https://doi.org/10.1016/j.biocel.2020.105885 [Google Scholar] [PubMed] [CrossRef]
Niture S, Moore J, Kumar D (2019). TNFAIP8: Inflammation, immunity and human diseases. Journal of Cellular Immunology 1: 29–34. [Google Scholar] [PubMed]
Niture S, Ramalinga M, Kedir H, Patacsil D, Niture SS, Li J, Mani H, Suy S, Collins S, Kumar D (2018b). TNFAIP8 promotes prostate cancer cell survival by inducing autophagy. Oncotarget 9: 26884–26899. https://doi.org/10.18632/oncotarget.25529 [Google Scholar] [PubMed] [CrossRef]
Padmavathi G, Banik K, Monisha J, Bordoloi D, Shabnam B, Arfuso F, Sethi G, Fan L, Kunnumakkara AB (2018). Novel tumor necrosis factor-α induced protein eight (TNFAIP8/TIPE) family: Functions and downstream targets involved in cancer progression. Cancer Letters 432: 260–271. https://doi.org/10.1016/j.canlet.2018.06.017 [Google Scholar] [PubMed] [CrossRef]
Pang Y, Zhao Y, Wang Y, Wang X, Wang R et al. (2020). TNFAIP8 promotes AML chemoresistance by activating ERK signaling pathway through interaction with Rac1. Journal of Experimental & Clinical Cancer Research 39: 158. https://doi.org/10.1186/s13046-020-01658-z [Google Scholar] [PubMed] [CrossRef]
Pollard JW (2004). Tumour-educated macrophages promote tumour progression and metastasis. Nature Reviews Cancer 4: 71–78. https://doi.org/10.1038/nrc1256 [Google Scholar] [PubMed] [CrossRef]
Porturas TP, Sun H, Buchlis G, Lou Y, Liang X, Cathopoulis T, Fayngerts S, Johnson DS, Wang Z, Chen YH (2015). Crucial roles of TNFAIP8 protein in regulating apoptosis and Listeria infection. Journal of Immunology 194: 5743–5750. https://doi.org/10.4049/jimmunol.1401987 [Google Scholar] [PubMed] [CrossRef]
Salmaninejad A, Valilou SF, Soltani A, Ahmadi S, Abarghan YJ, Rosengren RJ, Sahebkar A (2019). Tumor-associated macrophages: Role in cancer development and therapeutic implications. Cellular Oncology 42: 591–608. https://doi.org/10.1007/s13402-019-00453-z [Google Scholar] [PubMed] [CrossRef]
Shi TY, Cheng X, Yu KD, Sun MH, Shao ZM et al. (2013). Functional variants in TNFAIP8 associated with cervical cancer susceptibility and clinical outcomes. Carcinogenesis 34: 770–778. https://doi.org/10.1093/carcin/bgt001 [Google Scholar] [PubMed] [CrossRef]
Shi B, Hao Y, Li W, Dong H, Xu M, Gao P (2020). The enigmatic role of TIPE2 in asthma. American Journal of Physiology Lung Cellular and Molecular Physiology 319: L163–L172. https://doi.org/10.1152/ajplung.00069.2020 [Google Scholar] [PubMed] [CrossRef]
Shi C, Zhang S, Hong S, Pang J, Yesibulati Y, Yin P, Zhuang G (2016). The pro-apoptotic effects of TIPE2 on AA rat fibroblast-like synoviocytes via regulation of the DR5-caspase-NF-κB pathway in vitro. OncoTargets and Therapy 9: 993–1000. https://doi.org/10.2147/OTT.S92907 [Google Scholar] [PubMed] [CrossRef]
Singh N, Baby D, Rajguru JP, Patil PB, Thakkannavar SS, Pujari VB (2019). Inflammation and cancer. Annals of African Medicine 18: 121–126. https://doi.org/10.4103/aam.aam_56_18 [Google Scholar] [PubMed] [CrossRef]
Sun Y, Cao S, Li Z, Liu X, Xu J, Tian Y, Shen S, Zhou Y (2021). A novel prognostic factor TIPE2 inhibits cell proliferation and promotes apoptosis in pancreatic ductal adenocarcinoma (PDAC). International Journal of Medical Sciences 18: 2051–2062. https://doi.org/10.7150/ijms.51497 [Google Scholar] [PubMed] [CrossRef]
Sun H, Gong S, Carmody RJ, Hilliard A, Li L et al. (2008). TIPE2, a negative regulator of innate and adaptive immunity that maintains immune homeostasis. Cell 133: 415–426. https://doi.org/10.1016/j.cell.2008.03.026 [Google Scholar] [PubMed] [CrossRef]
Sun H, Lin M, Zamani A, Goldsmith JR, Boggs AE et al. (2020). The TIPE molecular pilot that directs lymphocyte migration in health and inflammation. Scientific Reports 10: 6617. https://doi.org/10.1038/s41598-020-63629-w [Google Scholar] [PubMed] [CrossRef]
Sun Z, Liu X, Song JH, Cheng Y, Liu Y, Jia Y, Meltzer SJ, Wang Z (2016). TNFAIP8 overexpression: A potential predictor of lymphatic metastatic recurrence in pN0 esophageal squamous cell carcinoma after Ivor Lewis esophagectomy. Tumor Biology 37: 10923–10934. https://doi.org/10.1007/s13277-016-4978-1 [Google Scholar] [PubMed] [CrossRef]
Sun H, Lou Y, Porturas T, Morrissey S, Luo G, Qi J, Ruan Q, Shi S, Chen YH (2015). Exacerbated experimental colitis in TNFAIP8-deficient mice. Journal of Immunology 194: 5736–5742. [Google Scholar]
Uhlén M, Fagerberg L, Hallström BM, Lindskog C, Oksvold P et al. (2015). Tissue-based map of the human proteome. Science 347: 1260419. https://doi.org/10.1126/science.1260419 [Google Scholar] [PubMed] [CrossRef]
Usman RM, Razzaq F, Akbar A, Farooqui AA, Iftikhar A et al. (2021). Role and mechanism of autophagy-regulating factors in tumorigenesis and drug resistance. Asia-Pacific Journal of Clinical Oncology 17: 193–208. https://doi.org/10.1111/ajco.13449 [Google Scholar] [PubMed] [CrossRef]
Vendramini-Costa DB, Carvalho JE (2012). Molecular link mechanisms between inflammation and cancer. Current Pharmaceutical Design 18: 3831–3852. https://doi.org/10.2174/138161212802083707 [Google Scholar] [PubMed] [CrossRef]
Wang L, Chen C, Feng S, Tian J (2018b). TIPE‐2 suppresses growth and aggressiveness of hepatocellular carcinoma cells through downregulation of the phosphoinositide 3‐kinase/AKT signaling pathway. Molecular Medicine Reports 17: 7017–7026. https://doi.org/10.3892/mmr.2018.8789 [Google Scholar] [PubMed] [CrossRef]
Wang LY, Fan YC, Zhao J, Gao S, Sun FK, Han J, Yang Y, Wang K (2014). Elevated expression of tumour necrosis factor-α-induced protein 8 (TNFAIP8)-like 2 mRNA in peripheral blood mononuclear cells is associated with disease progression of acute-on-chronic hepatitis B liver failure. Journal of Viral Hepatitis 21: 64–73. https://doi.org/10.1111/jvh.12116 [Google Scholar] [PubMed] [CrossRef]
Wang G, Guo C, Zhao H, Pan Z, Zhu F, Zhang L, Wang Q (2018a). TIPE3 differentially modulates proliferation and migration of human non-small-cell lung cancer cells via distinct subcellular location. BMC Cancer 18: 260. https://doi.org/10.1186/s12885-018-4177-0 [Google Scholar] [PubMed] [CrossRef]
Wang Y, Jiang Y, Zhou J, Song W, Li J et al. (2016a). Hepatitis C virus promotes hepatocellular carcinogenesis by targeting TIPE2, a new regulator of DNA damage response. Tumor Biology 37: 15265–15274. https://doi.org/10.1007/s13277-016-5409-z [Google Scholar] [PubMed] [CrossRef]
Wang Y, Liu Y, Hu C, Ni X, Huang X (2016b). Tumor necrosis factor α-induced protein 8-like 1 promotes apoptosis by regulating B-cell leukemia/lymphoma-2 family proteins in RAW264.7 cells. Oncology Letters 12: 3506–3512. https://doi.org/10.3892/ol.2016.5090 [Google Scholar] [PubMed] [CrossRef]
Wang K, Ren Y, Liu Y, Zhang J, He JJ (2017). Tumor necrosis factor (TNF)-α-induced protein 8-like-2 (TIPE2) inhibits proliferation and tumorigenesis in breast cancer cells. Oncology Research 25: 55–63. https://doi.org/10.3727/096504016X14719078133320 [Google Scholar] [PubMed] [CrossRef]
Woodward MJ, de Boer J, Heidorn S, Hubank M, Kioussis D, Williams O, Brady HJM (2010). Tnfaip8 is an essential gene for the regulation of glucocorticoid-mediated apoptosis of thymocytes. Cell Death & Differentiation 17: 316–323. https://doi.org/10.1038/cdd.2009.125 [Google Scholar] [PubMed] [CrossRef]
Wu Y, Antony S, Meitzler JL, Doroshow JH (2014). Molecular mechanisms underlying chronic inflammation-associated cancers. Cancer Letters 345: 164–173. https://doi.org/10.1016/j.canlet.2013.08.014 [Google Scholar] [PubMed] [CrossRef]
Wu DD, Liu SY, Gao YR, Lu D, Hong Y et al. (2019). Tumour necrosis factor-α-induced protein 8-like 2 is a novel regulator of proliferation, migration, and invasion in human rectal adenocarcinoma cells. Journal of Cellular and Molecular Medicine 23: 1698–1713. https://doi.org/10.1111/jcmm.14065 [Google Scholar] [PubMed] [CrossRef]
Wu X, Ma Y, Cheng J, Li X, Zheng H, Jiang L, Zhou R (2017). TIPE1 function as a prognosis predictor and negative regulator of lung cancer. Oncotarget 8: 78496–78506. https://doi.org/10.18632/oncotarget.19655 [Google Scholar] [PubMed] [CrossRef]
Xing Y, Liu Y, Liu T, Meng Q, Lu H, et al. (2018). TNFAIP8 promotes the proliferation and cisplatin chemoresistance of non-small cell lung cancer through MDM2/p53 pathway. Cell Communication and Signaling 16: 43. https://doi.org/10.1186/s12964-018-0254-x [Google Scholar] [PubMed] [CrossRef]
Yang J, Huang Y, Dong B, Dai Y (2021). Long noncoding RNA DLEU2 drives the malignant behaviors of thyroid cancer through mediating the miR-205-5p/TNFAIP8 axis. Endocrine Connections 10: 471–483. https://doi.org/10.1530/EC-21-0046 [Google Scholar] [PubMed] [CrossRef]
Yeung TL, Leung CS, Yip KP, Au Yeung CL, Wong STC, Mok SC (2015). Cellular and molecular processes in ovarian cancer metastasis. A review in the theme: Cell and molecular processes in cancer metastasis. American Journal of Physiology Cell Physiology 309: C444–C456. https://doi.org/10.1152/ajpcell.00188.2015 [Google Scholar] [PubMed] [CrossRef]
Yin H, Huang X, Tao M, Hu Q, Qiu J, Chen W, Wu J, Xie Y (2017). Adenovirus-mediated TIPE2 overexpression inhibits gastric cancer metastasis via reversal of epithelial-mesenchymal transition. Cancer Gene Therapy 24: 180–188. https://doi.org/10.1038/cgt.2017.3 [Google Scholar] [PubMed] [CrossRef]
Yuan F, Liu B, Xu Y, Li Y, Sun Q et al. (2019). TIPE3 is a regulator of cell apoptosis in glioblastoma. Cancer Letters 446: 1–14. https://doi.org/10.1016/j.canlet.2018.12.019 [Google Scholar] [PubMed] [CrossRef]
Zhang C, Chakravarty D, Sakabe I, Mewani R R, Boudreau HE, Kumar D, Ahmad I, Kasid UN (2006). Role of SCC-S2 in experimental metastasis and modulation of VEGFR-2, MMP-1, and MMP-9 expression. Molecular Therapy 13: 947–955. https://doi.org/10.1016/j.ymthe.2005.11.020 [Google Scholar] [PubMed] [CrossRef]
Zhang Z, Chang M, Song X, Wang K, Sun W et al. (2021). TIPE1 suppresses growth and metastasis of ovarian cancer. Journal of Oncology 2021: 5538911. https://doi.org/10.1155/2021/5538911 [Google Scholar] [PubMed] [CrossRef]
Zhang H, Han WJ, Zhang ZL (2022). The importance of tumor necrosis factor-α-induced protein-8 like-2 in the pathogenesis of cervical cancer and preeclampsia via regulation of cell invasion. The Tohoku Journal of Experimental Medicine 257: 181–191. https://doi.org/10.1620/tjem.2022.J026 [Google Scholar] [PubMed] [CrossRef]
Zhang Z, Liang X, Gao L, Ma H, Liu X et al. (2015c). TIPE1 induces apoptosis by negatively regulating Rac1 activation in hepatocellular carcinoma cells. Oncogene 34: 2566–2574. https://doi.org/10.1038/onc.2014.208 [Google Scholar] [PubMed] [CrossRef]
Zhang Z, Liu L, Cao S, Zhu Y, Mei Q (2017). Gene delivery of TIPE2 inhibits breast cancer development and metastasis via CD8+ T and NK cell-mediated antitumor responses. Molecular Immunology 85: 230–237. https://doi.org/10.1016/j.molimm.2017.03.007 [Google Scholar] [PubMed] [CrossRef]
Zhang Z, Liu L, Liu C, Cao S, Zhu Y, Mei Q (2016). TIPE2 suppresses the tumorigenesis, growth and metastasis of breast cancer via inhibition of the AKT and p38 signaling pathways. Oncology Reports 36: 3311–3316. https://doi.org/10.3892/or.2016.5192 [Google Scholar] [PubMed] [CrossRef]
Zhang Z, Qi H, Hou S, Jin X (2013). TIPE2 mRNA overexpression correlates with TNM staging in renal cell carcinoma tissues. Oncology Letters 6: 571–575. https://doi.org/10.3892/ol.2013.1388 [Google Scholar] [PubMed] [CrossRef]
Zhang L, Shi Y, Wang Y, Zhu F, Wang Q, Ma C, Chen Y H, Zhang L (2011). The unique expression profile of human TIPE2 suggests new functions beyond its role in immune regulation. Molecular Immunology 48: 1209–1215. https://doi.org/10.1016/j.molimm.2011.03.001 [Google Scholar] [PubMed] [CrossRef]
Zhang X, Wang J, Fan C, Li H, Sun H, Gong S, Chen YH, Shi Y (2009). Crystal structure of TIPE2 provides insights into immune homeostasis. Nature Structural & Molecular Biology 16: 89–90. https://doi.org/10.1038/nsmb.1522 [Google Scholar] [PubMed] [CrossRef]
Zhang YH, Yan HQ, Wang F, Wang YY, Jiang YN, Wang YN (2015b). TIPE2 inhibits TNF-α-induced hepatocellular carcinoma cell metastasis via Erk1/2 downregulation and NF-κB activation. International Journal of Oncology 46: 254–264. https://doi.org/10.3892/ijo.2014.2725 [Google Scholar] [PubMed] [CrossRef]
Zhang DL, Yang N (2020). TNFAIP8 regulates cisplatin resistance through TAF-Iα and promotes malignant progression of esophageal cancer. European Review for Medical and Pharmacological Sciences 24: 4775–4784. [Google Scholar] [PubMed]
Zhang H, Zhu T, Liu W, Qu X, Chen Y, Ren P, Wang Z, Wei X, Zhang Y, Yi F (2015a). TIPE2 acts as a negative regulator linking NOD2 and inflammatory responses in myocardial ischemia/reperfusion injury. Journal of Molecular Medicine 93: 1033–1043. https://doi.org/10.1007/s00109-015-1288-9 [Google Scholar] [PubMed] [CrossRef]
Zhao LL (2020). TIPE2 suppresses progression and tumorigenesis of the oral tongue squamous cell carcinoma by regulating FoxP3+ regulatory T cells. Journal of Bioenergetics and Biomembranes 52: 279–289. https://doi.org/10.1007/s10863-020-09840-w [Google Scholar] [PubMed] [CrossRef]
Zhao H, Ming T, Tang S, Ren S, Yang H, Liu M, Tao Q, Xu H (2022). Wnt signaling in colorectal cancer: Pathogenic role and therapeutic target. Molecular Cancer 21: 144. https://doi.org/10.1186/s12943-022-01616-7 [Google Scholar] [PubMed] [CrossRef]
Zhao P, Pang X, Jiang J, Wang L, Zhu X, Yin Y, Zhai Q, Xiang X, Feng F, Xu W (2019). TIPE1 promotes cervical cancer progression by repression of p53 acetylation and is associated with poor cervical cancer outcome. Carcinogenesis 40: 592–599. https://doi.org/10.1093/carcin/bgy163 [Google Scholar] [PubMed] [CrossRef]
Zhao P, Wang S, Jiang J, Liu H, Zhu X et al. (2018). TIPE2 sensitizes osteosarcoma cells to cis-platin by down-regulating MDR1 via the TAK1- NF-κB and—AP-1 pathways. Molecular Immunology 101: 471–478. https://doi.org/10.1016/j.molimm.2018.08.010 [Google Scholar] [PubMed] [CrossRef]
Zhao Q, Zhao M, Dong T, Zhou C, Peng Y, Zhou X, Fan B, Ma W, Han M, Liu S (2015). Tumor necrosis factor-α-induced protein-8 like-2 (TIPE2) upregulates p27 to decrease gastic cancer cell proliferation. Journal of Cellular Biochemistry 116: 1121–1129. https://doi.org/10.1002/jcb.25068 [Google Scholar] [PubMed] [CrossRef]
Zhong M, Chen Z, Yan Y, Bahet A, Cai X et al. (2021). Expression of TIPE family members in human colorectal cancer. Oncology Letters 21: 118. https://doi.org/10.3892/ol.2020.12379 [Google Scholar] [PubMed] [CrossRef]
Zhou Z, Li Z, Shen Y, Chen T (2021). MicroRNA-138 directly targets TNFAIP8 and acts as a tumor suppressor in osteosarcoma. Experimental and Therapeutic Medicine 22: 1403. https://doi.org/10.3892/etm.2021.10839 [Google Scholar] [PubMed] [CrossRef]
Zhu Y, Tao M, Wu J, Meng Y, Xu C, Tian Y, Zhou X, Xiang J, Zhang H, Xie Y (2016). Adenovirus-directed expression of TIPE2 suppresses gastric cancer growth via induction of apoptosis and inhibition of AKT and ERK1/2 signaling. Cancer Gene Therapy 23: 98–106. https://doi.org/10.1038/cgt.2016.6 [Google Scholar] [PubMed] [CrossRef]
Zhu L, Zhang X, Fu X, Li Z, Sun Z et al. (2018). TIPE2 suppresses progression and tumorigenesis of esophageal carcinoma via inhibition of the Wnt/β-catenin pathway. Journal of Translational Medicine 16: 7. https://doi.org/10.1186/s12967-018-1383-0 [Google Scholar] [PubMed] [CrossRef]
Cite This Article
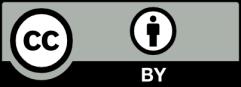
This work is licensed under a Creative Commons Attribution 4.0 International License , which permits unrestricted use, distribution, and reproduction in any medium, provided the original work is properly cited.