Open Access
REVIEW
Exploring exosomes to provide evidence for the treatment and prediction of Alzheimer’s disease
1 Edmond H. Fischer Signal Transduction Laboratory, School of Life Sciences, Jilin University, Changchun, 130012, China
2 Department of General Surgery, The Second Hospital of Jilin University, Changchun, 130041, China
* Corresponding Author: LINLIN ZENG. Email:
(This article belongs to the Special Issue: Novel Biomarkers in Diseases for Detection, Diagnosis, and Prognosis)
BIOCELL 2023, 47(10), 2163-2176. https://doi.org/10.32604/biocell.2023.031226
Received 23 May 2023; Accepted 22 June 2023; Issue published 08 November 2023
Abstract
Exosomes are extracellular vesicles with a 30–150 nm diameter originating from endosomes. In recent years, scientists have regarded exosomes as an ideal small molecule carrier for the targeted treatment of Alzheimer’s disease (AD) across the blood-brain barrier due to their nanoscale size and low immunogenicity. A large amount of evidence shows that exosomes are rich in biomarkers, and it has been found that the changes in biomarker content in blood, cerebrospinal fluid, and urine are often associated with the onset of AD patients. In this paper, some recent advances in the use of exosomes in the treatment of AD are reviewed, and various exosome markers and some latest detection methods are summarized to provide some evidence for the detection or treatment of AD by exosomes.Graphic Abstract
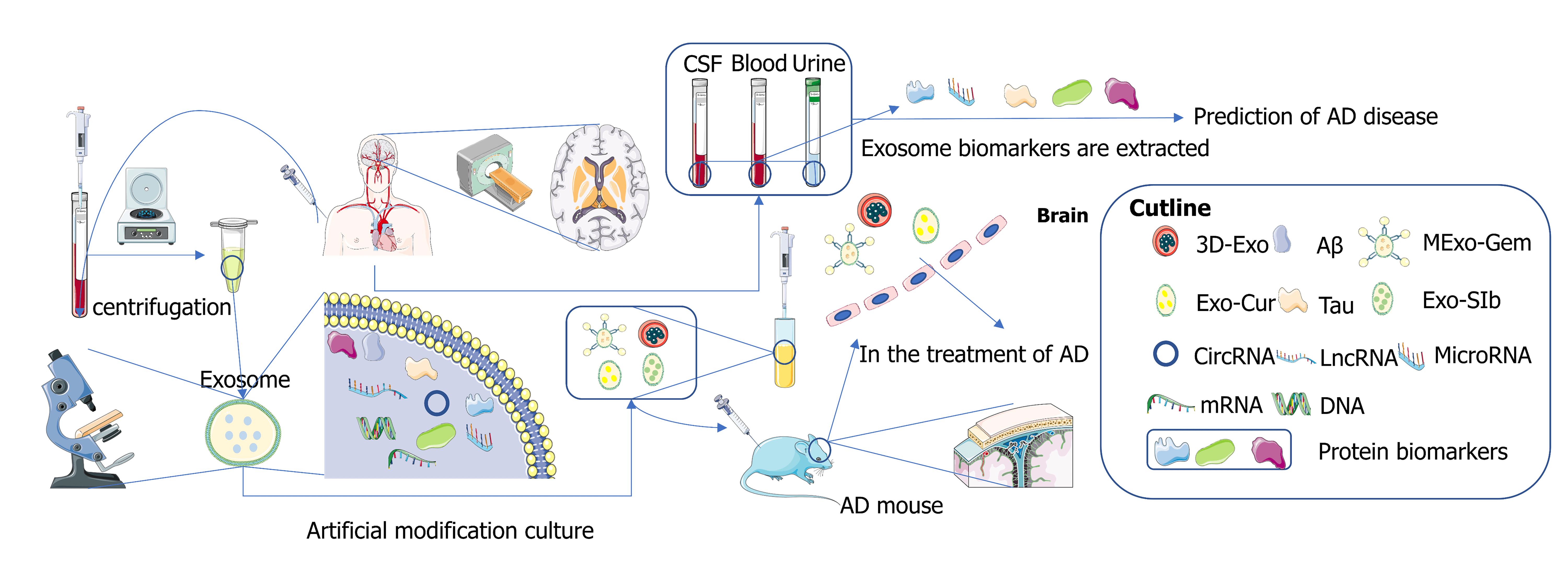
Keywords
Alzheimer’s disease (AD) is the most common dementia among older individuals, accounting for 60%–80% of the total population with dementia (Gopalakrishna et al., 2022). According to the data of Alzheimer’s Disease International, every 3 s, a patient develops AD in the world, and it is expected that by 2050, the number of AD worldwide will increase to more than 150 million (Joe and Ringman, 2019). Typical pathological features of AD mainly include neurofibrillary tangles (NFT) and senile plaques (Ising and Heneka, 2018), which often lead to damage or loss of synapses and neurons (Gkanatsiou et al., 2021). Accumulation of synaptic protein neurexin (axon protein) fragment in the brain also leads to specific memory loss (Fig. 1) (Sánchez-Hidalgo et al., 2022). Typical clinical symptoms of AD patients include decreased episodic memory and executive function (Tarawneh and Holtzman, 2012), while atypical clinical symptoms generally occur in the non-memory domain and are manifested as agnosia, aphasia, and executive dysfunction (Lam et al., 2013). Xia et al. (2022) found that human lifespan and memory storage are closely related to the C/EBPβ/AEP signaling pathway that drives AD, which closely links the pathogenesis of AD with the life cycle regulation. In this review, the association between exosomes and AD is explored and some evidence is provided for treating and predicting AD by exosomes. We hope to find a reasonable test and treatment to improve the quality of life of people affected with AD.
Figure 1: Pathogenesis of Alzheimer’s disease (AD). The figure illustrates several theories of the pathogenesis of AD, including neuron neurofibrillary tangles (NFT), senile plaques, and damage or loss of synapses.
Exosomes are small as vesicles. In recent years, exosomes have attracted the attention of scientists as a targeted vector molecule for treating AD. Exosomes can be used as drug carriers to cross the blood-brain barrier (BBB) and improve intracranial drug concentration to achieve therapeutic effects. Exosomes can also participate in the cleaning process of pathogenic amyloid beta (Aβ) protein and Tau protein, proving that exosomes can potentially treat AD. Mesenchymal stem cells (MSCs) are pluripotent stem cells capable of self-renewal and multidirectional differentiation (Samsonraj et al., 2017). MSC-exos is a subtype of extracellular microvesicles. As a type of exosome, its action process contributes to the improvement of immune regulation and neuroinflammation in pathological abnormal areas. It can significantly improve spatial learning ability and cognitive impairment of AD transgenic mice (Cui et al., 2019). Exosomes have nanoscale size and low immunogenicity, so they can be carriers of small molecules across the BBB to treat AD. In medical tests, exosome-derived proteins, lncRNAs, or miRNAs can be stably detected in blood or cerebrospinal fluid (CSF). These molecules are considered new biomarkers for diagnosing neurodegenerative diseases and have significant promise in diagnosing AD.
Exosome and Alzheimer’s Disease
Extracellular vesicles (EVs) comprise a group of heterogeneous membrane-derived vesicles of different origins, sizes, and characteristics and play a crucial role in cellular exchange (Gould and Raposo, 2013). Exosomes are heterogeneous subgroups of EVs, with size in the range of 30–150 nm, and originating from endosomes (Baietti et al., 2012; Bebelman et al., 2018). Exosomes comprise proteins, DNA, mRNA, microRNA, long non-coding RNA, and circular RNA involved in intercellular communication (Dai et al., 2020). Transmembrane ligands on the surface of the exosome can directly bind to surface receptors on recipient cells to generate downstream signaling cascades that activate target cells. Exosomes can also release molecules directly into the cytoplasm of target cells through fusion with the plasma membrane or internalization by the recipient cells for material exchange or information transfer (Gurung et al., 2021), which is closely related to the occurrence, development, and treatment of diseases (Jella et al., 2018).
Exosomes mediate the transcellular transduction of substances
Exosomes gradually lead to the pathogenesis of AD patients by delivering pathological forms of Aβ and Tau proteins (Song et al., 2020). Oligo-Aβ-containing exosomes isolated from the brain of AD patients have been found to be absorbed by SH-SY5Y cells (Fig. 2), resulting in cytotoxicity and transmission to other receptor cells (Sardar Sinha et al., 2018). Exosomes secreted by astrocytes can target Aβ to neuronal mitochondria, enhancing Aβ neurotoxicity by inducing apoptosis (Elsherbini et al., 2020). Exosomes can mediate the clearance of pathological proteins to protect neurons. For example, exosomes derived from bone marrow mesenchymal stem cells (BM-MSCs) reduce Aβ deposition and promote cognitive function recovery in AD mice by activating the sphingosine kinase (SphK)/S1P signaling pathway (Wang and Yang, 2021). These studies suggest that exosomes can mediate the clearance of pathological proteins to protect neurons. Exosomes can also serve as cell-to-cell communication devices, mediating the effect of trans-cell transduction. For example, sirtuin 2 is transmitted from oligodendrocytes to axons through exosomes, which deacetylates mitochondrial protein and enhances ATP production, providing a target for promoting axon bioenergy metabolism in diseases of the nervous system (Fig. 2). It can increase the energy capacity of mitochondria in axons, and the results prove that exosome-mediated transcellular signaling is an effective and robust mechanism (Schiapparelli et al., 2022).
Figure 2: Exosomes mediate the transport of protein. Exosomes can deliver p-Tau and amyloid beta (Aβ) proteins. Sirtuin 2 (SIRT2) is transmitted from oligodendrocytes to axons through exosomes, which deacetylates mitochondrial proteins and enhances ATP production (Schiapparelli et al., 2022). Oligo-Aβ-containing exosomes isolated from the brain of patients with AD are absorbed by SH-SY5Y cells, resulting in cytotoxicity and transmission to other receptor cells (Sardar Sinha et al., 2018). Mesenchymal stem cells (MSC)-exos can induce Th1 cells to convert to Th2 cells and reduce the potential of T cells to differentiate into effector T cells (Th17) that produce interleukin 17 (Xie et al., 2020).
Exosomes mediate some protein transport
Exosomes mediate the aggregation and clearance of Aβ
Aβ is produced by processing amyloid precursor protein (APP) as a physiological metabolite and its secretion into the extracellular environment. A balance between APP production and degradation/clearance controls the homeostasis level of extracellular Aβ. Studies have shown that in the case of endogenous injury, PC12-derived exosomes may promote the formation of Aβ protein fiber, which is closely related to the pathological dynamics of early AD (Yuyama et al., 2012). During the development of AD, protein deposits are formed at the later stage of Aβ aggregation. Before visible deposits are formed, a small amount of pathogenic Aβ accumulates, like a “seed” of aggregation, triggering more pathological proteins to accumulate and snowball (Uhlmann et al., 2020). When Aβ forms metastable oligomers heavier than 50 kDa, these are called Aβ oligomers (AβOs). AβOs can target to bind to dendritic spines, induce Tau mismatch, reduce neuronal activity (Schützmann et al., 2021) and induce the onset of AD. Exosomal membranes are particularly rich in GM1 ganglioside (He et al., 2022), which drives conformational changes of Aβ to form non-toxic amyloid fibrils and promote absorption of Aβ (Fernandez-Perez et al., 2017). Exosomes MExo-gem is a mannose-modified exosome containing the drug gemfibrozil. Macrophage-derived exosomes (Exos) drive conformational changes in Aβ to reduce amyloid fibrillary formation by binding Aβ and promoting microglial internalization of Aβ. Exosomes MExo-gem with Gem modification promotes microglia to clear Aβ by activating peroxisome proliferator-activated receptor-α, promoting nuclear translocation of transcription factor EB, and enhancing lysosomal activity (Hao et al., 2022) (Fig. 3). These pieces of evidence suggest that different exosomes from brain cells promote the formation of Aβ protein fiber. In addition, some exosomes can promote the absorption of Aβ protein by changing the conformation of Aβ protein.
Figure 3: Engineered exosome targeting therapy mechanisms. (a) Preparation of mannose-modified exosome containing gemfibrozil (MExo-gem) and exosomes that envelope curcumin (Exo-Cur). (b) Mechanism analysis of MExo-gem, Exo-Cur, and 3D-exo in the treatment of Alzheimer’s disease.
Exosomes mediate Tau transport
Abnormal intracellular aggregation of misfolded Tau proteins into insoluble aggregates is called neurofibrillary tangles (NFT), a typical pathological marker of AD (Sinsky et al., 2021). Tau spreads from the entorhinal cortex to the hippocampus early in the disease. The development of Tau pathology in AD is associated with progressive cognitive impairment and neuronal loss. Tau protein can be secreted from neurons through synaptic stimulation and transmitted along the nerve (Yamada et al., 2014). Tau fibrin can be transferred from cells to cells in vitro and in vivo (Sanders et al., 2014). Exosomes play an important role in cell communication and transport of pathogenic proteins associated with AD. Tau protein is recognized by specific cell vesicles called exosomes in the CSF of patients with AD (Saman et al., 2012). Tau first appears in the entorhinal cortex (EC) before any symptoms and then develops in a graded pattern, distributing to the hippocampus and neocortex. Increasing evidence indicates that pathological Tau proteins can diffuse between cells and recruit native Tau proteins, leading to the transformation into fibrous aggregates of pathological Tau proteins (Iba et al., 2013; Ahmed et al., 2014; Boluda et al., 2015). Intracellular accumulation of misfolded alpha-synuclein (αSyn) is a neuropathological marker of alpha-synuclein disease (Goedert et al., 2013). For AD patients, Tau and aSyn exist in the brain in the form of the copolymer, and the presence of mixed pathology of Tau and aSyn is associated with a higher risk of dementia (Sorrentino et al., 2017). Studies have shown that inoculation of pre-formed fibrous Tau protein into Tau transgenic mice can produce AD-like NFT pathology at a fairly rapid rate in connected brain regions (Iba et al., 2013; Ahmed et al., 2014; Boluda et al., 2015). Misfolded Tau proteins can diffuse through anatomically connected neurons, which indicates that Tau aggregates can spread beyond the trans-synaptic transmission, and pre-formed Tau aggregates can also diffuse beyond the synaptic connection (de Calignon et al., 2012; Harris et al., 2012; Liu et al., 2012), indicating the existence of non-synaptic transmission pathways (de Calignon et al., 2012). Microglia can effectively secrete exosomes. Studies have shown that microglia engulf Tau-containing cytopathic neurons or synapses and secrete Tau protein in exosomes, effectively delivering Tau protein to neurons (EL Andaloussi et al., 2013). Neutral Sphingomyelinase 2 (nSMase2) is a phosphoprotein phosphorylated only at serine residues (Back et al., 2018). Tau-containing exosomes secreted by microglia are sensitive to nSMase2 inhibition. This indicates that the synthesis of ceramides is crucial for exosome biogenesis (Asai et al., 2015). Higher levels of myelocyte-derived exosomes in CSF were closely associated with higher levels of Tau protein in CSF (Agosta et al., 2014). This evidence demonstrated that microglia depletion significantly inhibits Tau protein delivery to neuron exosomes and that microglia-derived exosomes deliver Tau protein to neurons in large quantities. In a recent study, the team used biotin molecular tags (Biotin) to label proteins, and electron microscopy showed that unbiased screening based on mass spectrometry identified about 200 transneuronal transported proteins (TNTP) isolated from the visual cortex. Most TNTP are present in exosomes, and viral TNTP, including Tau protein and β-synuclein, were detected in isolated exosomes and postsynaptic neurons. It indicates that the transport of TNTP by exosomes may be mediated by exosomes (Schiapparelli et al., 2022), providing new evidence for previous studies on the transport of Aβ and Tau proteins by exosomes.
Exploration of Exosome and Neuronal Regulation
Microglia mediate neuronal damage
In AD, astrocytes and microglia individually or jointly stimulate neuroinflammation through cell crosstalk, promoting pro-inflammatory cytokine release and neuronal loss (Kaur et al., 2019). Microglia can mediate synaptic elimination through phagocytosis (Lee and Chung, 2019), and activated microglia can release NO and pro-inflammatory cytokines, increase ROS levels, and induce oxidative stress damage of dopaminergic neurons (Jiang et al., 2019). Neuropathological analysis of AD showed that Aβ protein pathologic plaques promote the development of Tau protein pathology. The aggregation form of microtubule-associated protein Tau consists of paired helical filaments (PHF) (Li et al., 2016). The human natural PHF (AD-PHF) extracted from the brain of sporadic AD could induce Tau aggregation in the brain of wild-type (WT) mice. This PHF contains six human wild-types Tau isomers and all post-translational modification features of the PHF from the AD brain (Audouard et al., 2016). In AD-PHF injected 5*FAD mice, insoluble Tau protein levels were higher, Tau cortical diffusion was more important than WT mice, and the CDK5 kinase p25 activator was increased. Data showed that in vivo, Aβ enhanced the seeding of experimentally induced pathological fiber Tau protein (Vergara et al., 2019). Primary microglia can internalize Aβ fibrils and release vesicular bodies containing Aβ peptides (Gouwens et al., 2018). Microglia can directly absorb Aβ and degrade it in the lysosomal compartment (Govindpani et al., 2019).
Exosomes are involved in the bidirectional regulation of neurons
Neuron-derived exosomes can prevent the pro-inflammatory response of microglia cells by removing the Aβ protein (Yuyama et al., 2012). Moreover, adding exosomes inhibits the formation of toxic oligomers, thus effectively avoiding neuron injury. On the other hand, the transport of Tau protein by exosomes may cause neurotoxicity. The release and uptake of Tau protein between adjacent cells may play an important role in the pathological spread of Tau protein (Mohamed et al., 2013). Tau-containing exosomes from neurons can be specifically absorbed by microglia. When Tau protein cannot be degraded by microglia, it can be released through microglia exosomes and absorbed by neurons (Asai et al., 2015). Tau protein can be transported forward or backward through the endolysosomal pathway, ultimately enhancing the Tau protein-associated pathologic condition in recipient neurons (Wu et al., 2013).
There is increasing evidence that exosome-mediated communication plays an important physiological role in neuronal development, synaptic function, nerve regeneration, and neuron-glial interactions at the neural network level (Rajendran et al., 2014). According to the above existing studies, exosomes may have different regulatory effects on AD. Exosomes can inhibit the formation of toxic oligomers, remove Aβ protein, reduce the pro-inflammatory response of microglia cells, and effectively avoid neuronal damage in this way. On the other hand, microglia depletion significantly inhibited the transfer of Tau protein to neuronal exosomes, and many microglia-derived exosomes transferred Tau protein to neurons. Exosomes also promote the aggregation of glial Aβ protein. Abnormal aggregation of Tau and Aβ proteins will increase the probability of AD inflammatory induction.
The mechanism of mesenchymal stem cells—exos inhibiting neuroinflammation
Exosomes have great potential in medical detection and drug delivery due to their characteristics as transport vectors. Exosomes, as natural biological agents, can act as active molecules for cell-cell transport with good biocompatibility and instantaneously regulate the function of targeted cells (Iranifar et al., 2019). According to relevant studies, exosomes derived from mesenchymal stem cells (MSC-exos) can interact with target cells through different mechanisms. For example, MSC-exos can bind directly to membrane receptors, internalizing their contents into target cells. MSC-exos can also deliver bioactive substances to target cells by fusion with the plasma membrane. Composed of microvascular endothelial cells that line the brain’s capillaries, BBB acts as a highly selective membrane barrier that facilitates transport between systemic circulation and the central nervous system (Kadry et al., 2020). Exosomes can easily improve intracranial drug concentration through BBB (Qu et al., 2018). Compared with traditional administration methods, exosome administration avoids intracranial complications such as infection, non-specific absorption, and drug toxicity (Wang et al., 2019). The lipid bilayer structure of exosomes contributes to improving hydrophobic or hydrophilic drug transport efficiency (Kim et al., 2018). MSC-exos can regulate immunity, promote Aβ degradation and regulate Aβ degradation. MSC-exos regulates immune cells. MSC-exos can help inhibit the proliferation and differentiation of lymphocytes (Blazquez et al., 2014). In addition, MSC-exos is involved in inducing lymphocyte differentiation into anti-inflammatory type. This small molecule can induce Th1 cells to convert to Th2 cells and reduce the potential of T cells to differentiate into effector T cells (Th17) that produce interleukin 17 (Xie et al., 2020) (Fig. 2). In addition, some studies have suggested that inflammatory cytokines and proteins contained in MSC-exos have immunomodulatory effects. The excessive accumulation of Aβ in the brain triggers a neuroinflammatory process. MSC-exos contributes to improving immune regulation and neuroinflammation in pathologically abnormal areas. MSC-exos can reduce the expression of pro-inflammatory factors and up-regulate the expression of the anti-inflammatory factors in immune cells, exerting an immunosuppressive effect (Matthay and Abman, 2018).
Prospects for Exosomes in Therapy and Detection/Prediction of Alzheimer’s Disease
The prospect of exosomes for the treatment of Alzheimer’s disease
In some recent studies, the team used a 3-dimensional graphene scaffold and 2-dimensional graphene sheet as human umbilical cord mesenchymal stem cells (hUMSCs) culture substrate, extracted supernatant from hUMSCs and isolated exosomes, and found that 3D-Exo up-regulated A Disintegrin And Metalloproteinase 10 (ADAM10), down-regulated beta-secretase 1 (BACE1) expression, and decreased Aβ deposition in vitro and in vivo. It also reduces inflammation and oxidative stress in the brain by inhibiting microglia. 3D-exo significantly improved cognitive and memory abilities of AD model rats (Yang et al., 2020). This study provides a novel therapeutic intervention and the potential clinical application of exosomes extracted from hUMSCs grown on three-dimensional scaffolds for treating AD and other diseases. It demonstrates its efficacy and safety. Targeting amyloid dynamic balance is an important therapeutic strategy for AD. Through exosome modification, MExo-gem containing mannose-modified exosomes can bind to Aβ, and also possibly specifically target microglia by the interaction between mannose delivered by exosomes and mannose receptors expressed in microglia, thereby promoting Aβ entry into microglia. MExo-gem activates lysosome activity and accelerates Aβ clearance in microglia. MExo-gem improved AD model mice’s learning and memory ability (Hao et al., 2022). Exosomes are also used as drug carriers for AD therapy in nanomedicine delivery systems, where receptor-mediated endocytosis increases the solubility and permeability of curcumin through BBB. Engineered exosomes loaded with curcumin can prevent neuronal death by activating the AKT/GSK-3β pathway to inhibit Tau phosphorylation (Wang et al., 2019) (Fig. 3). Similarly, quercetin loaded in plasma exosomes can prevent Tau pathology better than its free form, thus achieving better AD treatment in quercetin exosystem agents (Qi et al., 2020). An exosecreting agent was created by combining genetic engineering with the co-transfection of parental cells. Rabies virus glycoprotein (RVG) is a cell-penetrating peptide with 29 amino acid residues. It can cross the BBB (Yang et al., 2023). The exosecreting agent exhibited RVG peptide on its surface, targeted at α7-nAChR, and enriched with neutral lysozyme variants with higher specificity and Aβ degradation. Exoinstitutional agents are preferentially internalized into cell lines in a level-dependent manner with α7-nAChR expression. When incubated with Aβ-producing N2a cells, it significantly reduced cellular endocrine levels of Aβ40. Exosystemic agents preferentially target the brain’s hippocampus, significantly reducing the expression of pro-inflammatory genes interleukin (IL)1α, tumor necrosis factor-α, and nuclear factor (NF)-κB, while increasing the expression of anti-inflammatory gene IL10 (Yu et al., 2021). Silibinin (SIB)-loaded macrophage-derived exosomes reverse Aβ-induced neuronal injury and reduce cognitive impairment in AD mice by regulating the NF-κB pathway (Huo et al., 2021). The above studies suggest that exosomes acting as bioengineered vectors or targeted drug delivery molecules can cross the BBB, improving transport rates and preventing complications of infection. Moreover, exosome-targeted vector therapy may improve learning and cognitive ability of mice with AD. These studies provide a basis for the clinical development of exosome drug therapy for AD.
Application of exosome-derived biomarkers in medical detection
Exosome derivatives predict the onset of Alzheimer’s disease
Exosomes can be used as markers for diagnosing and predicting diseases, including diabetes, obesity, cancer, and cardiovascular and neurodegenerative diseases (Lauritzen et al., 2020), and exosome biomarkers were found to be heterogeneous (Johnson et al., 2022). Reay et al. (2022) through linkage disequilibrium regression analysis technique and generalized statistical analysis of Genome-Wide Association Studies, found through gene association, that there is clear evidence of genetic overlap between blood biomarkers and psychiatric characteristics, including a series of biochemical indicators that tend to be associated with similar profiles of psychiatric disorders. Extensive changes in exo-miRNA expression levels were detected in AD and Parkinson’s disease (PD) patients by small RNA sequencing, and eight miRNAs were significantly elevated/decreased in AD and PD samples compared to controls (Nie et al., 2020) (Fig. 4), suggesting that the expression of exo-miRNA is somehow related to the pathogenesis of AD and PD.
Figure 4: Application of exosome in medical detection. (a) Alzheimer’s disease (AD) can be diagnosed by positron emission tomography (PET) or by measuring concentrations of Aβ and P-Tau in cerebrospinal fluid (Sonuç Karaboga and Sezgintürk, 2020). (b) Extensive changes in exo-miRNA expression levels were detected in patients with AD and PD patients by small RNA sequencing, and eight miRNAs were significantly elevated/decreased in AD and PD samples compared to those in controls (Nie et al., 2020).
Exosome derivative biomarkers in cerebrospinal fluid can accurately predict Alzheimer’s disease
CSF exosome derivative biomarkers can accurately predict AD. The concentration of p-Tau in CSF is closely correlated with AD (Hanes et al., 2020), and the phosphorylation spectrum of soluble Tau in the brain of AD patients is highly correlated with that of CSF in AD patients (Horie et al., 2020). Aβ protein is also one of the biomarkers of AD patients, and Aβ deposition is a relatively late result of Aβ aggregation in AD. Increased Aβ42/Aβ40 ratio can directly lead to accumulation and aggregation of Tau protein (Kwak et al., 2020), which can be detected by using positron emission tomography imaging or by measuring Aβ and p-Tau concentrations in CSF as biomarkers for diagnosis and tracking of AD in patients (Fig. 4) (Blennow, 2021). At present, plasma Aβ42/Aβ40 ratio measurement by immunoprecipitation mass spectrometry (IP-MS) can achieve more than 90% accuracy in identifying brain amyloid Aβ protein degeneration (Nakamura et al., 2018). As one of these biomarkers, Aβ has great potential in predicting AD. Therefore, the researchers established a method to detect the ratio of Aβ monomer (AD biomarker) in CSF using the competitive synergistic effect of Cu2+ between CDs and Aβ monomer using the adaptive characteristics of Eu/GMP network (Liu et al., 2020). This method provides an idea for early diagnosis of AD and a better understanding of the chemical nature of AD. The combination of exosome growth-associated protein 43 (GAP43), neuro granule protein, synaptosome-associated protein 25, and synaptotagmin 1 was found to detect preclinical AD 5 to 7 years before cognitive impairment by comparing AD patients with healthy individuals (Jia et al., 2021).
Prediction of blood exosome biomarkers and their derivatives on Alzheimer’s disease
Because of the trauma of the biopsy, a cheap, safe, and accurate prediction method is needed to detect AD. As an ideal method, the detection of exosome biomarkers has come into people’s field of vision. In one experiment, the concentrations of Aβ42, T-Tau, and p-Tau-T181 secreted in exosomes of AD were higher than those in control groups, confirming the consistency between CSF and exosome biomarkers. Exosome Aβ42, T-Tau, and p-Tau-T181 were confirmed to have the same ability to diagnose AD as CSF (Jia et al., 2019). The continuous increase in p-Tau protein levels in the blood and its high specificity with AD make blood-derived p-Tau a potential marker for the pathophysiological detection of AD (Benussi et al., 2020). Plasma p-Tau231—a p-Tau protein with high diagnostic accuracy in distinguishing between AD and non-AD dementia (AUC = 0.93), demonstrating the potential clinical utility of plasma p-Tau (Ashton et al., 2021). A disposable neurobiosensor probe for the determination of Tau-441 proteins has been developed using a nanocomposite composed of reduced graphene oxide (rGO) and gold nanoparticles (AuNP) using electrochemical impedance spectroscopy and cyclic voltammetry (CV). The nanocomposite surface (rGO-AuNP) modified with 11-mercaptoundecanoic acid (11-MUA) covalent anchor showed higher sensitivity. The neurobiosensor probe could capture the Tau-441 target protein in serum and CSF samples with recovery rates ranging from 96% to 108% (Sonuç Karaboga and Sezgintürk, 2020), suggesting that AD can be detected. The researchers used an interdigitated microelectrode (IME) as an impedance biosensor and detected blood-based Aβ using the gold nanoparticles (AuNPs) sandwich method. The IMEs sensor can detect Aβ with high sensitivity and selectivity according to its level. Mouse plasma samples were prepared from the blood of double-mutant APP/PS1 transgenic (TG) and wild-type (WT) mice, and the diagnostic ability of AD was tested by Aβ assay in the plasma samples. They found that the AuNPs sandwich method assisted Aβ detection and successfully distinguished TG and WT mice groups. Therefore, this sensing system can detect Aβ with high sensitivity and selectivity (Yoo et al., 2020). Rossi et al. (2020) efficiently and completely extracted the HAS-Aβ peptide complex from plasma by the Pierce albumin removal method, which could be identified using albumin biomarkers. Nano-probes can deliver clear signals to Aβ targets, which is a good choice for early diagnosis of AD (Hamd-Ghadareh et al., 2022). Due to the transient heterogeneity of Aβ aggregates, it is not easy to dynamically monitor Aβ and its aggregation intermediates by constructing a two-dimensional manganese dioxide nano-enzyme sensor array. The nano-enzyme biosensor system can accurately detect Aβ species and related aggregation processes in clinical blood samples (Hu et al., 2022).
According to the definition of AD by the National Institute of Aging (NIA) and the Alzheimer’s Association (AA), the clinical symptoms of AD can be divided into six stages. The existing research on the prediction of AD mainly focuses on the second stage: subjective cognitive decline (SCD), and the third stage: mild cognitive impairment (MCI). In one trial, two biomarkers, Aβ42 and a sniffer stick (SS-16), were used separately and in combination in patients with MCI and AD dementia. Lower SS-16 scores and higher Aβ42 levels in NDE were found in MCI and AD dementia. For the longitudinal group, 8 individuals with MCI developed AD dementia within 2 years, and 16 individuals with MCI developed AD dementia within 3 years. The combination of the SS-16 score and Aβ42 level in NDE showed better prediction of the transition from MCI to AD dementia at 2–3 years than did a single indicator (Zhao et al., 2020). Exosome-derived microRNAs are also good markers for predicting AD. ATP-binding cassette transporter A1 (ABCA1) is used as a marker to capture specific exosomes. Evaluation of the levels of ABCA1-labeled exosomes microRNA-135a (miR-135a) revealed that ABCA1 exosomes harvested from HT-22 cells and neuronal media were significantly higher than erythrocytes and leukocytes. The level of ABCA1-labeled exosome miR-135a in patients with MCI and AD (DAT) was higher than that in the control group and slightly increased in the serum of patients with SCD, suggesting that the level of exosome marker miR-135a can be used to diagnose AD (Liu et al., 2021). Another study reported that miR-30b-5p, miR-22-3p, and miR-378a-3p were significantly dysregulated in AD patients (Dong et al., 2021). A predictive model for AD was established by logistic regression analysis of serum exosome secretion markers. Combining these three miRs yielded better diagnostic ability (AUC = 0.88).
Urine exosome biomarker detection—a potential predictive method for Alzheimer’s disease
Exosomes in the blood can predict the progression of AD, and biomarkers can also be extracted to predict the progression of AD in urine. In one study, enzyme-linked immunoadsorption assay (ELISA) was used to detect levels of Aβ42 and p-Tau-S396 (standardized by CD63) in urinary exosomes from patients with AD and matched healthy subjects. The exosome concentration, particle size, and phenotype were measured and the nanoparticles were observed by transmission electron microscopy. The levels of Aβ42 and p-Tau-S396 in the urine exosomes of patients with AD were higher than those of matched healthy controls, and more exosomes were extracted from patients with AD. This experiment showed that early AD diagnosis can be achieved by detecting Aβ42, p-Tau-S396 levels, and urinary exosome content (Sun et al., 2019). These results suggest that marker detection is more accurate in CSF than in blood and urine marker. However, some studies have shown that combining some biomarkers in blood can achieve a similar detection effect as CSF biomarkers and confirmed that blood biomarkers such as Aβ42, T-Tau, and p-Tau-T181 have the same diagnostic ability as CSF markers. Urine exosome secretion markers also have certain predictive potential. Exosome-derived biomarkers in blood and urine are expected to provide a suitable method for accurately detecting AD in the future.
Multiple omics analysis can be used to predict multiple markers of Alzheimer’s disease
Johnson et al. (2022) analyzed the proteomics of more than 1000 AD brain tissues using tandem mass tag mass spectrometry (TMT-MS). RNA expression network modules constructed by transcription data and proteins obtained from TMT-MS overlap with the TMT AD protein network in 168 RNA networks. It was also found that protein network modules were more strongly correlated with cognitive function than RNA network modules. Using laser capture microscopy (LCM) and laber-free unlabeled quantitative method proteomic analysis, several TMT AD protein network modules were enriched in NFTs. Such as silk crack the originally activated protein kinase (MARK)/metabolic modules, and there is space between the iconic AD symptoms of consistency, the team by using the method of multiple omics analysis, they found a close relationship between the protein and the AD network module, and signaling by different protein markers and RNA network analysis can reveal the pathogenesis of AD. Exosome derivative markers and corresponding detection methods can also be used to predict AD development (Table 1) accurately. Combined analysis of blood or urine samples using a combination of exosome derivative markers can greatly improve the accuracy of prediction and replace the method of AD prediction by CSF, which is safer to implement. ELISA and similar methods have a price advantage over omics analysis. It has great potential value in medical detection applications. In the future, a clinical detection method is expected to be established based on the characteristics of biomarkers derived from different exosomes, that can use the combined analysis of multiple markers to accurately predict AD incidence.
The clinical situation of exosome application
Clinical progress of exosome biomarker detection methods
Through ClinicalTrials.Gov database (https://clinicaltrials.gov/) to collect AD, PD, and other neurological problems related to clinical data (Tables 2 and 3). Most of the clinical trials using biomarkers to detect AD in recent years stayed in the pre-phase III or phase NA. Very few trials enter the clinical phase IV. According to the current clinical results and clinical data, most exosome-derived biomarkers to predict related neurological diseases such as AD and PD are exosome-derived biomarkers. Biomarkers in patients with AD were investigated in two clinical trials using simvastatin administration (CTN: NCT01142336) and photo bioregulatory therapy (CTN: NCT03405662). These two clinical trials observed changes in the levels of typical AD-related biomarkers, such as Aβ42 and Tau, in the blood or CSF of these subjects. However, after analysis, the data were not statistically significant. The clinical data on these psychiatric disorders and the related reports mentioned above give us some experience in predicting AD. Using the correlation level changes of biomarkers, combined with gene association analysis, small RNA sequencing, SS-16, and other combined detection methods to predict whether patients are in the SCD and MCI stages, seems to prevent the conversion of SCD and MCI to AD to a certain extent. In a recent report, to further explore the potential of biomarkers to predict SCD, the Aß42/Aß40 ratio and the levels of T-tau and p-Tau in the CSF were used to explore differences between patients with SCD and controls (CO) of normal patients. The domain value of the Aß42/Aß40 ratio is calculated by means of data modeling for the definition of amyloid-positive protein. Comprehensive analysis of positive rates of different proteins defined in this clinical trial proved that the positive predictive value (PPV) of AD was 0.9 (Jessen et al., 2023), which provides a new scheme for the future clinical use of exosome-derived biomarkers to predict AD.
Advances in the clinical treatment of exosomes
The clinical evidence on the use of exosomes in the treatment of AD is still in a relatively nascent stage. Although researchers have carried out relevant clinical trials to explore the safety evaluation of exosomes in patients with AD (CTN: NCT04388982), clear clinical data is needed to prove the reliability and safety of exosomes in treating AD. However, new progress has been made in using exosomes to treat ischemic brain pawns. Neural stem cells (NSC) combined with NSC-derived exosomes have a significant therapeutic effect on ischemic stroke. Exosomes regulate downstream target genes through the miRNA they carry, thereby downregulating oxidative stress and inflammation in brain tissue, inhibiting cell apoptosis, and ultimately promoting the survival and differentiation of transplanted NSC (Zhang et al., 2023). This study showed that exosome drugs have great potential for treating neuropathic diseases. Currently, exosome-related drugs have been developed for the treatment of tumors. Exosome candidate therapy exoSTING can activate the local dose-dependent STING signaling pathway in tumors, activating the immune response, and promoting the uptake of tumor-resident antigen-presenting cells (Jang et al., 2021). This innovative therapy is the first clinical proof of concept. Although no exosome drugs have entered the clinical validation stage for AD treatment, the advantages of exosomes themselves is expected to be used to achieve targeted therapy for AD in the future.
Exosomes have the ability to transmit Aβ and Tau proteins; therefore, they are associated with the onset of AD. In addition, exosomes have the function of clearing Aβ protein to reduce neuroinflammation. Exosomes are considered potential drug vectors for the treatment of AD due to their BBB-crossing and low immunity properties. The synthetic exosomes MExo-gem promotes microglia to clear Aβ by activating PPARα. 3D-EXO up-regulates ADAM10, down-regulates BACE1 expression, and reduces Aβ deposition in vivo and in vitro. It reduces inflammation and oxidative stress in the brain by inhibiting microglia. SIB-Exo reverses Aβ-induced neuronal damage and reduces cognitive impairment in AD mice by regulating the NF-κB pathway. These experiments provide evidence for the future clinical treatment of AD with exosomes.
As research continues to evolve, partial biomarkers in the blood/urine/CSF show the potential to detect AD. According to our collection of clinical evidence and existing studies, some exosome-derived protein biomarkers have the ability to diagnose AD early, such as Aβ42, T-tau, and p-Tau. MicroRNAs derived from exosomes in blood/urine/CSF have also been found to have the ability to diagnose AD, such as miR-135a, miR-30b-5p, and miR-22-3p. Although to date, no exosome drug has been approved for clinical use in AD patients. It is expected that the detection of AD by exosome-derived biomarkers and the treatment of AD by exosome drugs can be realized in the future due to the potential excellent properties of exosomes.
Acknowledgement: Thanks to the editors and reviewers for their revisions.
Funding Statement: This research was funded by grants from the National Key Research and Development Program of China (Grant Number 2021YFA1500400). Science and Technology Department of Jilin Province (Grant Number 20200201386JC). Science and Technology Department of Jilin Province [Grant Number 20190701037GH]. Education Department of Jilin Province (Grant Number JJKH20200948KJ). The funding bodies played no role in the study design, in the collection, analysis, and interpretation of data, in the report’s writing, and in the decision to submit the article for publication.
Author Contributions: The authors confirm the following contributions to the paper. Study conception and design: Linlin Zeng and Xiangyu Quan; data collection: Xiangyu Quan and Jiangtao Li; draft manuscript preparation: Xiangyu Quan, Linlin Zeng, Xueting Ma, Guodong Li, and Xueqi Fu; preparation of figures: Xiangyu Quan, Jiangtao Li, and Xueting Ma; manuscript checking and approval: Linlin Zeng, Guodong Li, and Xueqi Fu. All authors reviewed the results and approved the final version of the manuscript.
Availability of Data and Materials: Data sharing not applicable to this article as no datasets were generated or analyzed during the current study.
Ethics Approval: Not applicable.
Conflicts of Interest: The authors declare that they have no conflicts of interest to report regarding the present study.
References
Agosta F, Dalla Libera D, Spinelli EG, Finardi A, Canu E et al. (2014). Myeloid microvesicles in cerebrospinal fluid are associated with myelin damage and neuronal loss in mild cognitive impairment and Alzheimer disease. Annals of Neurology 76: 813–825. https://doi.org/10.1002/ana.24235 [Google Scholar] [PubMed] [CrossRef]
Ahmed Z, Cooper J, Murray TK, Garn K, McNaughton E et al. (2014). A novel in vivo model of tau propagation with rapid and progressive neurofibrillary tangle pathology: The pattern of spread is determined by connectivity, not proximity. Acta Neuropathologica 127: 667–683. https://doi.org/10.1007/s00401-014-1254-6 [Google Scholar] [PubMed] [CrossRef]
Asai H, Ikezu S, Tsunoda S, Medalla M, Luebke J, Haydar T, Wolozin B, Butovsky O, Kügler S, Ikezu T (2015). Depletion of microglia and inhibition of exosome synthesis halt tau propagation. Nature Neuroscience 18: 1584–1593. https://doi.org/10.1038/nn.4132 [Google Scholar] [PubMed] [CrossRef]
Ashton NJ, Pascoal TA, Karikari TK, Benedet AL, Lantero-Rodriguez J et al. (2021). Plasma p-tau231: A new biomarker for incipient Alzheimer’s disease pathology. Acta Neuropathologica 141: 709–724. https://doi.org/10.1007/s00401-021-02275-6 [Google Scholar] [PubMed] [CrossRef]
Audouard E, Houben S, Masaracchia C, Yilmaz Z, Suain V et al. (2016). High-molecular-weight paired helical filaments from Alzheimer brain induces seeding of wild-type mouse tau into an Argyrophilic 4R tau pathology in vivo. American Journal of Pathology 186: 2709–2722. https://doi.org/10.1016/j.ajpath.2016.06.008 [Google Scholar] [PubMed] [CrossRef]
Back MJ, Ha HC, Fu Z, Choi JM, Piao Y, Won JH, Jang JM, Shin IC, Kim DK (2018). Activation of neutral sphingomyelinase 2 by starvation induces cell-protective autophagy via an increase in Golgi-localized ceramide. Cell Death & Disease 9: 670. https://doi.org/10.1038/s41419-018-0709-4 [Google Scholar] [PubMed] [CrossRef]
Baietti MF, Zhang Z, Mortier E, Melchior A, Degeest G et al. (2012). Syndecan-syntenin-ALIX regulates the biogenesis of exosomes. Nature Cell Biology 14: 677–685. https://doi.org/10.1038/ncb2502 [Google Scholar] [PubMed] [CrossRef]
Bebelman MP, Smit MJ, Pegtel DM, Baglio SR (2018). Biogenesis and function of extracellular vesicles in cancer. Pharmacology & Therapeutics 188: 1–11. https://doi.org/10.1016/j.pharmthera.2018.02.013 [Google Scholar] [PubMed] [CrossRef]
Benussi A, Karikari TK, Ashton N, Gazzina S, Premi E et al. (2020). Diagnostic and prognostic value of serum NfL and p-Tau181 in frontotemporal lobar degeneration. Journal of Neurology Neurosurgery and Psychiatry 91: 960–967. https://doi.org/10.1136/jnnp-2020-323487 [Google Scholar] [PubMed] [CrossRef]
Blazquez R, Sanchez-Margallo FM, de la Rosa O, Dalemans W, Alvarez V, Tarazona R, Casado JG (2014). Immunomodulatory potential of human adipose mesenchymal stem cells derived exosomes on in vitro stimulated T cells. Frontiers in Immunology 5: 556. https://doi.org/10.3389/fimmu.2014.00556 [Google Scholar] [PubMed] [CrossRef]
Blennow K (2021). Phenotyping Alzheimer’s disease with blood tests. Science 373: 626–628. https://doi.org/10.1126/science.abi5208 [Google Scholar] [PubMed] [CrossRef]
Boluda S, Iba M, Zhang B, Raible KM, Lee VMY, Trojanowski JQ (2015). Differential induction and spread of tau pathology in young PS19 tau transgenic mice following intracerebral injections of pathological tau from Alzheimer’s disease or corticobasal degeneration brains. Acta Neuropathologica 129: 221–237. https://doi.org/10.1007/s00401-014-1373-0 [Google Scholar] [PubMed] [CrossRef]
Cui GH, Guo HD, Li H, Zhai Y, Gong ZB, Wu J, Liu JS, Dong YR, Hou SX, Liu JR (2019). RVG-modified exosomes derived from mesenchymal stem cells rescue memory deficits by regulating inflammatory responses in a mouse model of Alzheimer’s disease. Immunity & Ageing 16: 10. https://doi.org/10.1186/s12979-019-0150-2 [Google Scholar] [PubMed] [CrossRef]
Dai J, Su Y, Zhong S, Cong L, Liu B, Yang J, Tao Y, He Z, Chen C, Jiang Y (2020). Exosomes: Key players in cancer and potential therapeutic strategy. Signal Transduction and Targeted Therapy 5: 145. https://doi.org/10.1038/s41392-020-00261-0 [Google Scholar] [PubMed] [CrossRef]
de Calignon A, Polydoro M, Suárez-Calvet M, William C, Adamowicz DH et al. (2012). Propagation of tau pathology in a model of early Alzheimer’s disease. Neuron 73: 685–697. https://doi.org/10.1016/j.neuron.2011.11.033 [Google Scholar] [PubMed] [CrossRef]
Dong Z, Gu H, Guo Q, Liang S, Xue J et al. (2021). Profiling of serum exosome MiRNA reveals the potential of a MiRNA panel as diagnostic biomarker for Alzheimer’s disease. Molecular Neurobiology 58: 3084–3094. https://doi.org/10.1007/s12035-021-02323-y [Google Scholar] [PubMed] [CrossRef]
El Andaloussi S, Maeger I, Breakefield XO, Wood MJA (2013). Extracellular vesicles: Biology and emerging therapeutic opportunities. Nature Reviews Drug Discovery 12: 348–358. [Google Scholar]
Elsherbini A, Kirov AS, Dinkins MB, Wang G, Qin H, Zhu Z, Tripathi P, Crivelli SM, Bieberich E (2020). Association of Aβ with ceramide-enriched astrosomes mediates Aβ neurotoxicity. Acta Neuropathologica Communications 8: 60. https://doi.org/10.1186/s40478-020-00931-8 [Google Scholar] [PubMed] [CrossRef]
Fernandez-Perez EJ, Sepulveda FJ, Peoples R, Aguayo LG (2017). Role of membrane GM1 on early neuronal membrane actions of Aβ during onset of Alzheimer’s disease. Biochimica Et Biophysica Acta-Molecular Basis of Disease 1863: 3105–3116. https://doi.org/10.1016/j.bbadis.2017.08.013 [Google Scholar] [PubMed] [CrossRef]
Gkanatsiou E, Nilsson J, Toomey CE, Vrillon A, Kvartsberg H et al. (2021). Amyloid pathology and synaptic loss in pathological aging. Journal of Neurochemistry 159: 258–272. https://doi.org/10.1111/jnc.15487 [Google Scholar] [PubMed] [CrossRef]
Goedert M, Spillantini MG, Del Tredici K, Braak H (2013). 100 years of Lewy pathology. Nature Reviews Neurology 9: 13–24. https://doi.org/10.1038/nrneurol.2012.242 [Google Scholar] [PubMed] [CrossRef]
Gopalakrishna G, Joshi P, Tsai PH, Patel N (2022). Advances in Alzheimer’s Dementia: An update for clinicians. American Journal of Geriatric Psychiatry 30: S11. https://doi.org/10.1016/j.jagp.2022.01.268 [Google Scholar] [CrossRef]
Gould SJ, Raposo G (2013). As we wait: Coping with an imperfect nomenclature for extracellular vesicles. Journal of Extracellular Vesicles 2: 10. https://doi.org/10.3402/jev.v2i0.20389 [Google Scholar] [PubMed] [CrossRef]
Gouwens LK, Ismail MS, Rogers VA, Zeller NT, Garrad EC, Amtashar FS, Makoni NJ, Osborn DC, Nichols MR (2018). Aβ42 protofibrils interact with and are trafficked through microglial-derived microvesicles. ACS Chemical Neuroscience 9: 1416–1425. https://doi.org/10.1021/acschemneuro.8b00029 [Google Scholar] [PubMed] [CrossRef]
Govindpani K, McNamara LG, Smith NR, Vinnakota C, Waldvogel HJ, Faull RL, Kwakowsky A (2019). Vascular dysfunction in Alzheimer’s disease: A prelude to the pathological process or a consequence of it? Journal of Clinical Medicine 8: 651. https://doi.org/10.3390/jcm8050651 [Google Scholar] [PubMed] [CrossRef]
Gurung S, Perocheau D, Touramanidou L, Baruteau J (2021). The exosome journey: From biogenesis to uptake and intracellular signalling. Cell Communication and Signaling 19: 47. https://doi.org/10.1186/s12964-021-00730-1 [Google Scholar] [PubMed] [CrossRef]
Hamd-Ghadareh S, Salimi A, Parsa S, Mowla SJ (2022). Development of three-dimensional semi-solid hydrogel matrices for ratiometric fluorescence sensing of Amyloid β peptide and imaging in SH-SY5 cells: Improvement of point of care diagnosis of Alzheimer’s disease biomarker. Biosensors & Bioelectronics 199: 113895. https://doi.org/10.1016/j.bios.2021.113895 [Google Scholar] [PubMed] [CrossRef]
Hanes J, Kovac A, Kvartsberg H, Kontsekova E, Fialova L et al. (2020). Evaluation of a novel immunoassay to detect p-tau Thr217 in the CSF to distinguish Alzheimer disease from other dementias. Neurology 95: e3026–e3035. https://doi.org/10.1212/WNL.0000000000010814 [Google Scholar] [PubMed] [CrossRef]
Hao Y, Su C, Liu X, Sui H, Shi Y, Zhao L (2022). Bioengineered microglia-targeted exosomes facilitate Aβ clearance via enhancing activity of microglial lysosome for promoting cognitive recovery in Alzheimer’s disease. Biomaterials Advances 136: 212770. https://doi.org/10.1016/j.bioadv.2022.212770 [Google Scholar] [PubMed] [CrossRef]
Harris JA, Koyama A, Maeda S, Ho K, Devidze N, Dubal DB, Yu GQ, Masliah E, Mucke L (2012). Human P301L-mutant tau expression in mouse entorhinal-hippocampal network causes tau aggregation and presynaptic pathology but no cognitive deficits. PLoS One 7: e45881. https://doi.org/10.1371/journal.pone.0045881 [Google Scholar] [PubMed] [CrossRef]
He X, Guan F, Lei L (2022). Structure and function of glycosphingolipids on small extracellular vesicles. Glycoconjugate Journal 39: 197–205. https://doi.org/10.1007/s10719-022-10052-0 [Google Scholar] [PubMed] [CrossRef]
Horie K, Barthélemy NR, Mallipeddi N, Li Y, Franklin EE, Perrin RJ, Bateman RJ, Sato C (2020). Regional correlation of biochemical measures of amyloid and tau phosphorylation in the brain. Acta Neuropathologica Communications 8: 149. https://doi.org/10.1186/s40478-020-01019-z [Google Scholar] [PubMed] [CrossRef]
Hu S, Yang C, Li Y, Luo Q, Luo H (2022). Nanozyme sensor array based on manganese dioxide for the distinction between multiple amyloid β peptides and their dynamic aggregation process. Biosensors & Bioelectronics 199: 113881. https://doi.org/10.1016/j.bios.2021.113881 [Google Scholar] [PubMed] [CrossRef]
Huo Q, Shi Y, Qi Y, Huang L, Sui H, Zhao L (2021). Biomimetic silibinin-loaded macrophage-derived exosomes induce dual inhibition of Aβ aggregation and astrocyte activation to alleviate cognitive impairment in a model of Alzheimer’s disease. Materials Science & Engineering C-Materials for Biological Applications 129: 112365. https://doi.org/10.1016/j.msec.2021.112365 [Google Scholar] [PubMed] [CrossRef]
Iba M, Guo JL, McBride JD, Zhang B, Trojanowski JQ, Lee VMY (2013). Synthetic tau fibrils mediate transmission of neurofibrillary tangles in a transgenic mouse model of Alzheimer’s-like Tauopathy. Journal of Neuroscience 33: 1024–1037. https://doi.org/10.1523/JNEUROSCI.2642-12.2013 [Google Scholar] [PubMed] [CrossRef]
Iranifar E, Seresht BM, Momeni F, Fadaei E, Mehr MH, Ebrahimi Z, Rahmati M, Kharazinejad E, Mirzaei H (2019). Exosomes and microRNAs: New potential therapeutic candidates in Alzheimer disease therapy. Journal of Cellular Physiology 234: 2296–2305. https://doi.org/10.1002/jcp.27214 [Google Scholar] [PubMed] [CrossRef]
Ising C, Heneka MT (2018). Functional and structural damage of neurons by innate immune mechanisms during neurodegeneration. Cell Death & Disease 9: 120. https://doi.org/10.1038/s41419-017-0153-x [Google Scholar] [PubMed] [CrossRef]
Jang SC, Economides KD, Moniz RJ, Sia CL, Lewis N et al. (2021). ExoSTING, an extracellular vesicle loaded with STING agonists, promotes tumor immune surveillance. Communications Biology 4: 497. https://doi.org/10.1038/s42003-021-02004-5 [Google Scholar] [PubMed] [CrossRef]
Jella KK, Nasti TH, Li ZT, Malla SR, Buchwald ZS, Khan MK (2018). Exosomes, their biogenesis and role in inter-cellular communication, tumor microenvironment and cancer immunotherapy. Vaccines 6: 69. https://doi.org/10.3390/vaccines6040069 [Google Scholar] [PubMed] [CrossRef]
Jessen F, Wolfsgruber S, Kleineindam L, Spottke A, Altenstein S et al. (2023). Subjective cognitive decline and stage 2 of Alzheimer disease in patients from memory centers. Alzheimers & Dementia 19: 487–497. https://doi.org/10.1002/alz.12674 [Google Scholar] [PubMed] [CrossRef]
Jia L, Qiu Q, Zhang H, Chu L, Du Y et al. (2019). Concordance between the assessment of Aβ42, T-tau, and P-T181-tau in peripheral blood neuronal-derived exosomes and cerebrospinal fluid. Alzheimers & Dementia 15: 1071–1080. https://doi.org/10.1016/j.jalz.2019.05.002 [Google Scholar] [PubMed] [CrossRef]
Jia L, Zhu M, Kong C, Pang Y, Zhang H et al. (2021). Blood neuro-exosomal synaptic proteins predict Alzheimer’s disease at the asymptomatic stage. Alzheimers & Dementia 17: 49–60. https://doi.org/10.1002/alz.12166 [Google Scholar] [PubMed] [CrossRef]
Jiang DQ, Ma YJ, Wang Y, Lu HX, Mao SH, Zhao SH (2019). Microglia activation induces oxidative injury and decreases SIRT3 expression in dopaminergic neuronal cells. Journal of Neural Transmission 126: 559–568. https://doi.org/10.1007/s00702-019-02005-z [Google Scholar] [PubMed] [CrossRef]
Joe E, Ringman JM (2019). Cognitive symptoms of Alzheimer’s disease: Clinical management and prevention. BMJ 367: 16217. https://doi.org/10.1136/bmj.l6217 [Google Scholar] [PubMed] [CrossRef]
Johnson ECB, Carter EK, Dammer EB, Duong DM, Gerasimov ES et al. (2022). Large-scale deep multi-layer analysis of Alzheimer’s disease brain reveals strong proteomic disease-related changes not observed at the RNA level. Nature Neuroscience 25: 213–225. https://doi.org/10.1038/s41593-021-00999-y [Google Scholar] [PubMed] [CrossRef]
Kadry H, Noorani B, Cucullo L (2020). A blood-brain barrier overview on structure, function, impairment, and biomarkers of integrity. Fluids and Barriers of the CNS 17: 69. https://doi.org/10.1186/s12987-020-00230-3 [Google Scholar] [PubMed] [CrossRef]
Kaur D, Sharma V, Deshmukh R (2019). Activation of microglia and astrocytes: A roadway to neuroinflammation and Alzheimer’s disease. Inflammopharmacology 27: 663–677. https://doi.org/10.1007/s10787-019-00580-x [Google Scholar] [PubMed] [CrossRef]
Kim MS, Haney MJ, Zhao Y, Yuan D, Deygen I, Klyachko NL, Kabanov AV, Batrakova EV (2018). Engineering macrophage-derived exosomes for targeted paclitaxel delivery to pulmonary metastases: In vitro and in vivo evaluations. Nanomedicine 14: 195–204. https://doi.org/10.1016/j.nano.2017.09.011 [Google Scholar] [PubMed] [CrossRef]
Kwak SS, Washicosky KJ, Brand E, von Maydell D, Aronson J et al. (2020). Amyloid-β42/40 ratio drives tau pathology in 3D human neural cell culture models of Alzheimer’s disease. Nature Communications 11: 1377. https://doi.org/10.1038/s41467-020-15120-3 [Google Scholar] [PubMed] [CrossRef]
Lam B, Masellis M, Freedman M, Stuss DT, Black SE (2013). Clinical, imaging, and pathological heterogeneity of the Alzheimer’s disease syndrome. Alzheimer’s Research & Therapy 5: 1. https://doi.org/10.1186/alzrt155 [Google Scholar] [PubMed] [CrossRef]
Lauritzen P, Ogitani Y, Sugiura K, Takahashi N, Alečković M et al. (2020). Extracellular vesicle and particle biomarkers define multiple human cancers. Cell 182: 1044–1061. https://doi.org/10.1016/j.cell.2020.07.009 [Google Scholar] [PubMed] [CrossRef]
Lee E, Chung WS (2019). Glial control of synapse number in healthy and diseased brain. Frontiers in Molecular Neuroscience 13: 42. https://doi.org/10.3389/fncel.2019.00042 [Google Scholar] [PubMed] [CrossRef]
Li T, Braunstein KE, Zhang J, Lau A, Sibener L, Deeble C, Wong PC (2016). The neuritic plaque facilitates pathological conversion of tau in an Alzheimer’s disease mouse model. Nature Communications 7: 12082. https://doi.org/10.1038/ncomms12082 [Google Scholar] [PubMed] [CrossRef]
Liu L, Drouet V, Wu JW, Witter MP, Small SA, Clelland C, Duff K (2012). Trans-synaptic spread of tau pathology in vivo. PLoS One 7: e31302. https://doi.org/10.1371/journal.pone.0031302 [Google Scholar] [PubMed] [CrossRef]
Liu C, Lu D, You X, Shi G, Deng J, Zhou T (2020). Carbon dots sensitized lanthanide infinite coordination polymer nanoparticles: Towards ratiometric fluorescent sensing of cerebrospinal Aβ monomer as a biomarker for Alzheimer’s disease. Analytica Chimica Acta 1105: 147–154. https://doi.org/10.1016/j.aca.2020.01.021 [Google Scholar] [PubMed] [CrossRef]
Liu CG, Meng S, Li Y, Lu Y, Zhao Y, Wang PC (2021). MicroRNA-135a in ABCA1-labeled exosome is a serum biomarker candidate for Alzheimer’s disease. Biomedical and Environmental Sciences 34: 19–28. [Google Scholar] [PubMed]
Matthay MA, Abman SH (2018). Exosome-based therapy for bronchopulmonary dysplasia. American Journal of Respiratory and Critical Care Medicine 197: 10–12. https://doi.org/10.1164/rccm.201709-1796ED [Google Scholar] [PubMed] [CrossRef]
Mohamed NV, Herrou T, Plouffe V, Piperno N, Leclerc N (2013). Spreading of tau pathology in Alzheimer’s disease by cell-to-cell transmission. European Journal of Neuroscience 37: 1939–1948. https://doi.org/10.1111/ejn.12229 [Google Scholar] [PubMed] [CrossRef]
Nakamura A, Kaneko N, Villemagne VL, Kato T, Doecke J et al. (2018). High performance plasma amyloid-β biomarkers for Alzheimer’s disease. Nature 554: 249–254. https://doi.org/10.1038/nature25456 [Google Scholar] [PubMed] [CrossRef]
Nie C, Sun Y, Zhen H, Guo M, Ye J, Liu Z, Yang Y, Zhang X (2020). Differential expression of plasma Exo-miRNA in neurodegenerative diseases by next-generation sequencing. Frontiers in Neuroscience 14: 438. https://doi.org/10.3389/fnins.2020.00438 [Google Scholar] [PubMed] [CrossRef]
Qi Y, Guo L, Jiang Y, Shi Y, Sui H, Zhao L (2020). Brain delivery of quercetin-loaded exosomes improved cognitive function in AD mice by inhibiting phosphorylated tau-mediated neurofibrillary tangles. Drug Delivery 27: 745–755. https://doi.org/10.1080/10717544.2020.1762262 [Google Scholar] [PubMed] [CrossRef]
Qu M, Lin Q, Huang L, Fu Y, Wang L et al. (2018). Dopamine-loaded blood exosomes targeted to brain for better treatment of Parkinson’s disease. Journal of Controlled Release 287: 156–166. https://doi.org/10.1016/j.jconrel.2018.08.035 [Google Scholar] [PubMed] [CrossRef]
Rajendran L, Bali J, Barr MM, Court FA, Krämer-Albers EM et al. (2014). Emerging roles of extracellular vesicles in the nervous system. Journal of Neuroscience 34: 15482–15489. https://doi.org/10.1523/JNEUROSCI.3258-14.2014 [Google Scholar] [PubMed] [CrossRef]
Reay WR, Kiltschewskij DJ, Geaghan MP, Atkins JR, Carr VJ, Green MJ, Cairns MJ (2022). Genetic estimates of correlation and causality between blood-based biomarkers and psychiatric disorders. Science Advances 8: eabj8969. https://doi.org/10.1126/sciadv.abj8969 [Google Scholar] [PubMed] [CrossRef]
Rossi E, Tran NT, Hirtz C, Lehmann S, Taverna M (2020). Efficient extraction of intact HSA-Aβ peptide complexes from sera: Toward albuminome biomarker identification. Talanta 216: 121002. https://doi.org/10.1016/j.talanta.2020.121002 [Google Scholar] [PubMed] [CrossRef]
Saman S, Kim W, Raya M, Visnick Y, Miro S et al. (2012). Exosome-associated tau is secreted in tauopathy models and is selectively phosphorylated in cerebrospinal fluid in early Alzheimer disease. Journal of Biological Chemistry 287: 3842–3849. https://doi.org/10.1074/jbc.M111.277061 [Google Scholar] [PubMed] [CrossRef]
Samsonraj RM, Raghunath M, Nurcombe V, Hui JH, van Wijnen AJ, Cool SM (2017). Concise review: Multifaceted characterization of human mesenchymal stem cells for use in regenerative medicine. Stem Cells Translational Medicine 6: 2173–2185. https://doi.org/10.1002/sctm.17-0129 [Google Scholar] [PubMed] [CrossRef]
Sanders DW, Kaufman SK, DeVos SL, Sharma AM, Mirbaha H et al. (2014). Distinct tau prion strains propagate in cells and mice and define different Tauopathies. Neuron 82: 1271–1288. https://doi.org/10.1016/j.neuron.2014.04.047 [Google Scholar] [PubMed] [CrossRef]
Sardar Sinha M, Ansell-Schultz A, Civitelli L, Hildesjö C, Larsson M, Lannfelt L, Ingelsson M, Hallbeck M (2018). Alzheimer’s disease pathology propagation by exosomes containing toxic amyloid-beta oligomers. Acta Neuropathologica 136: 41–56. https://doi.org/10.1007/s00401-018-1868-1 [Google Scholar] [PubMed] [CrossRef]
Schiapparelli LM, Sharma P, He HY, Li J, Shah SH et al. (2022). Proteomic screen reveals diverse protein transport between connected neurons in the visual system. Cell Reports 38: 110287. https://doi.org/10.1016/j.celrep.2021.110287 [Google Scholar] [PubMed] [CrossRef]
Schützmann MP, Hasecke F, Bachmann S, Zielinski M, Hänsch S, Schröder GF, Zempel H, Hoyer W (2021). Endo-lysosomal Aβ concentration and pH trigger formation of Aβ oligomers that potently induce Tau missorting. Nature Communications 12: 4634. https://doi.org/10.1038/s41467-021-24900-4 [Google Scholar] [PubMed] [CrossRef]
Sinsky J, Pichlerova K, Hanes J (2021). Tau protein interaction partners and their roles in Alzheimer’s disease and other Tauopathies. International Journal of Molecular Sciences 22: 9207. https://doi.org/10.3390/ijms22179207 [Google Scholar] [PubMed] [CrossRef]
Song Z, Xu Y, Deng W, Zhang L, Zhu H, Yu P, Qu Y, Zhao W, Han Y, Qin C (2020). Brain derived exosomes are a double-edged sword in Alzheimer’s disease. Frontiers in Molecular Neuroscience 13: 79. https://doi.org/10.3389/fnmol.2020.00079 [Google Scholar] [PubMed] [CrossRef]
Sonuç Karaboga MN, Sezgintürk MK (2020). Analysis of Tau-441 protein in clinical samples using rGO/AuNP nanocomposite-supported disposable impedimetric neuro-biosensing platform: Towards Alzheimer’s disease detection. Talanta 219: 121257. https://doi.org/10.1016/j.talanta.2020.121257 [Google Scholar] [PubMed] [CrossRef]
Sorrentino ZA, Brooks MMT, HudsonIII V, Rutherford NJ, Golde TE, Giasson BI, Chakrabarty P (2017). Intrastriatal injection of α-synuclein can lead to widespread synucleinopathy independent of neuroanatomic connectivity. Molecular Neurodegeneration 12: 40. https://doi.org/10.1186/s13024-017-0182-z [Google Scholar] [PubMed] [CrossRef]
Sun R, Wang H, Shi Y, Gao D, Sun Z, Chen Z, Jiang H, Zhang J (2019). A pilot study of urinary exosomes in Alzheimer’s disease. Neurodegenerative Diseases 19: 184–191. https://doi.org/10.1159/000505851 [Google Scholar] [PubMed] [CrossRef]
Sánchez-Hidalgo AC, Arias-Aragón F, Romero-Barragán MT, Martín-Cuevas C, Delgado-García JM, Martinez-Mir A, Scholl FG (2022). Selective expression of the neurexin substrate for presenilin in the adult forebrain causes deficits in associative memory and presynaptic plasticity. Experimental Neurology 347: 113896. https://doi.org/10.1016/j.expneurol.2021.113896 [Google Scholar] [PubMed] [CrossRef]
Tarawneh R, Holtzman DM (2012). The clinical problem of symptomatic Alzheimer disease and mild cognitive impairment. Cold Spring Harbor Perspectives in Medicine 2: a006148. https://doi.org/10.1101/cshperspect.a006148 [Google Scholar] [PubMed] [CrossRef]
Uhlmann RE, Rother C, Rasmussen J, Schelle J, Bergmann C et al. (2020). Acute targeting of pre-amyloid seeds in transgenic mice reduces Alzheimer-like pathology later in life. Nature Neuroscience 23: 1580–1588. https://doi.org/10.1038/s41593-020-00737-w [Google Scholar] [PubMed] [CrossRef]
Vergara C, Houben S, Suain V, Yilmaz Z, de Decker R et al. (2019). Amyloid-β pathology enhances pathological fibrillary tau seeding induced by Alzheimer PHF in vivo. Acta Neuropathologica 137: 397–412. https://doi.org/10.1007/s00401-018-1953-5 [Google Scholar] [PubMed] [CrossRef]
Wang H, Sui H, Zheng Y, Jiang Y, Shi Y, Liang J, Zhao L (2019). Curcumin-primed exosomes potently ameliorate cognitive function in AD mice by inhibiting hyperphosphorylation of the tau protein through the AKT/GSK-3β pathway. Nanoscale 11: 7481–7496. https://doi.org/10.1039/C9NR01255A [Google Scholar] [PubMed] [CrossRef]
Wang X, Yang G (2021). Bone marrow mesenchymal stem cells-derived exosomes reduce Abeta deposition and improve cognitive function recovery in mice with Alzheimer’s disease by activating sphingosine kinase/sphingosine-1-phosphate signaling pathway. Cell Biology International 45: 775–784. https://doi.org/10.1002/cbin.11522 [Google Scholar] [PubMed] [CrossRef]
Wu JW, Herman M, Liu L, Simoes S, Acker CM et al. (2013). Small misfolded tau species are internalized via bulk endocytosis and anterogradely and retrogradely transported in neurons. Journal of Biological Chemistry 288: 1856–1870. https://doi.org/10.1074/jbc.M112.394528 [Google Scholar] [PubMed] [CrossRef]
Xia Y, Qadota H, Wang ZH, Liu P, Liu X et al. (2022). Neuronal C/EBPβ/AEP pathway shortens life span via selective GABAnergic neuronal degeneration by FOXO repression. Science Advances 8: eabj8658. https://doi.org/10.1126/sciadv.abj8658 [Google Scholar] [PubMed] [CrossRef]
Xie M, Xiong W, She Z, Wen Z, Abdirahman AS, Wan W, Wen C (2020). Immunoregulatory effects of stem cell-derived extracellular vesicles on immune cells. Frontiers in Immunology 11: 13. https://doi.org/10.3389/fimmu.2020.00013 [Google Scholar] [PubMed] [CrossRef]
Yamada K, Holth JK, Liao F, Stewart FR, Mahan TE et al. (2014). Neuronal activity regulates extracellular tau in vivo. Journal of Experimental Medicine 211: 387–393. https://doi.org/10.1084/jem.20131685 [Google Scholar] [PubMed] [CrossRef]
Yang J, Wang P, Jiang X, Xu J, Zhang M et al. (2023). A nanotherapy of octanoic acid ameliorates cardiac arrest/cardiopulmonary resuscitation-induced brain injury via RVG29- and neutrophil membrane-mediated injury relay targeting. ACS Nano 17: 3528–3548. https://doi.org/10.1021/acsnano.2c09931 [Google Scholar] [PubMed] [CrossRef]
Yang LY, Zhai YX, Hao Y, Zhu ZC, Cheng GS (2020). The regulatory functionality of exosomes derived from hUMSCs in 3D culture for Alzheimer’s disease therapy. Small 16: e1906273. https://doi.org/10.1002/smll.201906273 [Google Scholar] [PubMed] [CrossRef]
Yoo YK, Kim G, Park D, Kim J, Kim Y, Kim HY, Yang SY, Lee JY, Hwang KS (2020). Gold nanoparticles assisted sensitivity improvement of interdigitated microelectrodes biosensor for amyloid-β detection in plasma sample. Sensors and Actuators B: Chemical 308: 127710. https://doi.org/10.1016/j.snb.2020.127710 [Google Scholar] [CrossRef]
Yu Y, Li W, Mao L, Peng W, Long D, Li D, Zhou R, Dang X (2021). Genetically engineered exosomes display RVG peptide and selectively enrich a neprilysin variant: A potential formulation for the treatment of Alzheimer’s disease. Journal of Drug Targeting 29: 1128–1138. https://doi.org/10.1080/1061186X.2021.1929257 [Google Scholar] [PubMed] [CrossRef]
Yuyama K, Sun H, Mitsutake S, Igarashi Y (2012). Sphingolipid-modulated exosome secretion promotes clearance of amyloid-β by microglia. The Journal of Biological Chemistry 287: 10977–10989. https://doi.org/10.1074/jbc.M111.324616 [Google Scholar] [PubMed] [CrossRef]
Zhang R, Mao W, Niu L, Bao W, Wang Y et al. (2023). NSC-derived exosomes enhance therapeutic effects of NSC transplantation on cerebral ischemia in mice. eLife 12: e84493. https://doi.org/10.7554/eLife.84493 [Google Scholar] [PubMed] [CrossRef]
Zhao A, Li Y, Yan Y, Qiu Y, Li B, Xu W, Wang Y, Liu J, Deng Y (2020). Increased prediction value of biomarker combinations for the conversion of mild cognitive impairment to Alzheimer’s dementia. Translational Neurodegeneration 9: 30. https://doi.org/10.1186/s40035-020-00210-5 [Google Scholar] [PubMed] [CrossRef]
Cite This Article
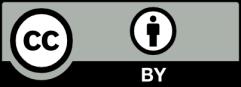
This work is licensed under a Creative Commons Attribution 4.0 International License , which permits unrestricted use, distribution, and reproduction in any medium, provided the original work is properly cited.