Open Access
REVIEW
CPT1A in cancer: Tumorigenic roles and therapeutic implications
Medical School, Kunming University of Science and Technology, Kunming, 650500, China
* Corresponding Author: ZHIZHOU SHI. Email:
(This article belongs to the Special Issue: Mitochondrial Signaling and Metabolism in Cancer)
BIOCELL 2023, 47(10), 2207-2215. https://doi.org/10.32604/biocell.2023.027677
Received 08 November 2022; Accepted 14 April 2023; Issue published 08 November 2023
Abstract
Metabolic reprogramming frequently occurs in the majority of cancers, wherein fatty acid oxidation (FAO) is usually induced and serves as a compensatory mechanism to improve energy consumption. Carnitine palmitoyltransferase 1A (CPT1A) is the rate-limiting enzyme for FAO and is widely involved in tumor growth, metastasis, and chemo-/radio-resistance. This review summarizes the most recent advances in understanding the oncogenic roles and mechanisms of CPT1A in tumorigenesis, including in proliferation and tumor growth, invasion and metastasis, and the tumor microenvironment. Importantly, CPT1A has been shown to be a biomarker for diagnosis and prognosis prediction and proved to be a candidate therapeutic target, especially for the treatment of drug- and radiation-resistant tumors. In summary, CPT1A plays remarkable roles in promoting cancer progression and is a potential anticancer therapeutic target.Graphic Abstract
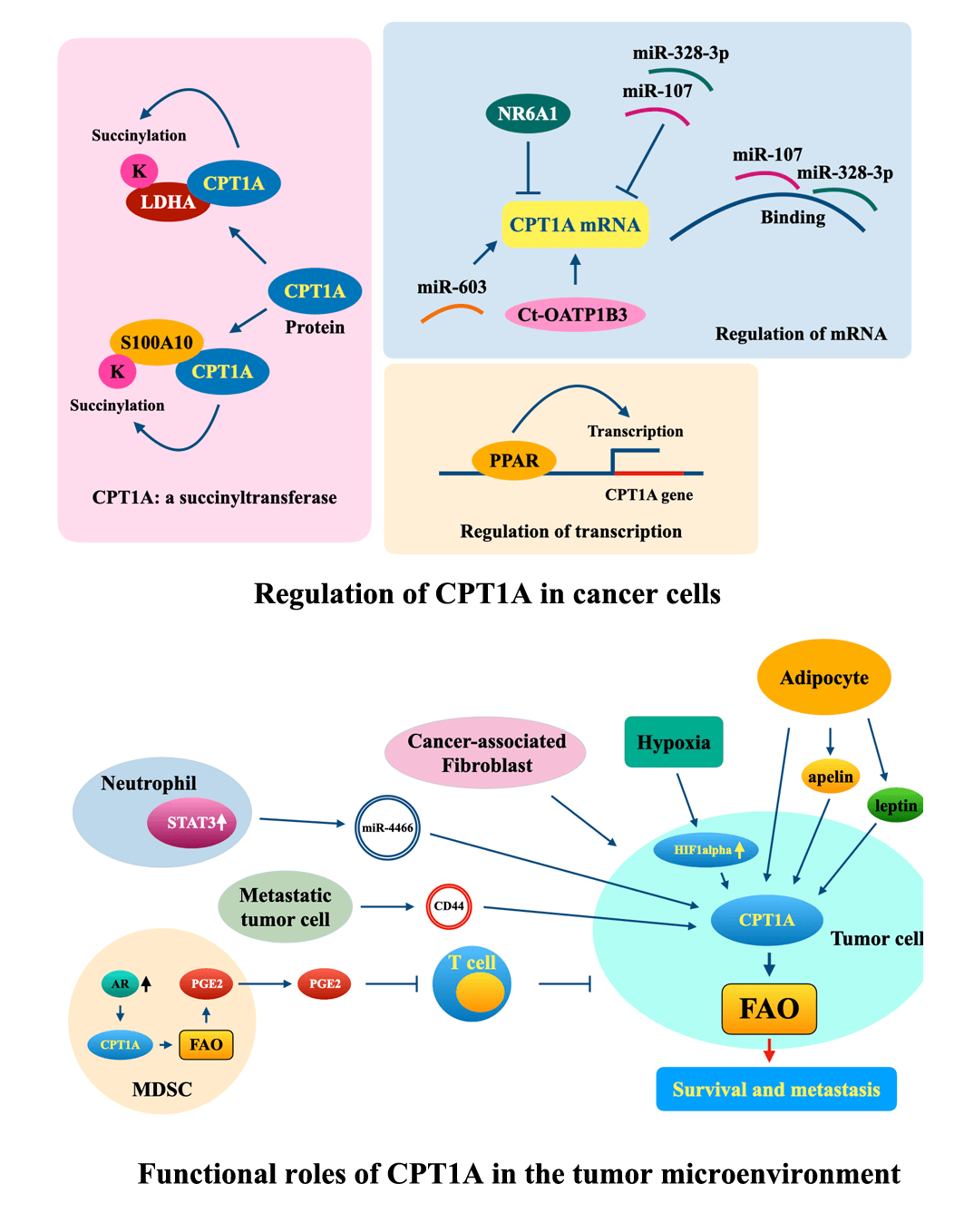
Keywords
Metabolic reprogramming is one of the critical features of cancer and maintains the rapid proliferation of cancer cells by affecting energy metabolism. In addition to the well-studied dysregulation of glucose metabolism (the Warburg effect), compelling studies have revealed the significant roles of changes in lipid metabolism during carcinogenesis (Santos and Schulze, 2012). Lipid metabolism consists of lipid synthesis and oxidation, and importantly, enhancement of fatty acid oxidation (FAO) promotes the metastasis, stemness, and treatment resistance of cancer cells (Wright et al., 2017; He et al., 2019; Jiang et al., 2022). CPT1, a key enzyme of FAO, has three isoforms: Carnitine palmitoyltransferase 1A (CPT1A; liver isoform), CPT1B (muscle isoform), and CPT1C (brain isoform) (Bonnefont et al., 2004). CPT1A controls the rate-limiting process in fatty acid β-oxidation, during which acyl-coenzyme A (acyl-CoA) esters are converted into acyl-carnitines. CPT1A is reported to be dysregulated in many types of cancers and regulates nearly all aspects of tumorigenesis (Lu et al., 2021a; Peng et al., 2021; Tang et al., 2022b; Wang et al., 2020b). Herein, we reviewed the most recent findings on the roles, mechanisms, diagnostic and prognostic relevance, and therapeutic applications of CPT1A in cancer.
The Biological Function of Carnitine Palmitoyltransferase 1A in Fatty Acid Oxidation
FAO is a vital metabolic pathway that produces nicotinamide adenine dinucleotide phosphate (NADPH) and adenosine triphosphate (ATP). Human cells have three types of FAO: α- (functions in peroxisomes), β- (functions in peroxisomes and mitochondria), and ω-oxidation (functions in the endoplasmic reticulum) (Talley and Mohiuddin, 2022). In mitochondria, diet-derived fatty acids are β-oxidized to produce energy, while β-oxidization in peroxisomes is usually carried out to generate H2O2 (Reddy and Hashimoto, 2001). Enhancement of mitochondrial β-oxidization confers stemness and chemoresistance to cancer cells; therefore, β-oxidization might be developed as a therapeutic target in the future (Li et al., 2022; Liu et al., 2023).
Cells take up fatty acids (FAs) by fatty acid transport molecules such as the CD36 molecule (CD36) (Glatz and Luiken, 2018) and FA-binding proteins (Lee et al., 2018). Then, fatty acyl-CoA synthetases convert FAs to fatty acyl-CoA, and then fatty acyl-CoA participates in oxidation. CPT1 catalyzes the transformation of fatty acyl-CoA to fatty acyl-carnitine and transfers it across the outer mitochondrial membrane. Then, carnitine/acylcarnitine translocase shuttles fatty acyl-carnitine into the mitochondrial matrix, and CPT2, which is located on the matrix surface of the inner membrane, reconverts fatty acyl-carnitine to fatty acyl-CoA (Adeva-Andany et al., 2017). Next, fatty acyl-CoA is sequentially catalyzed by acyl-CoA dehydrogenase, enoyl-CoA hydratase, hydroxyacyl-CoA dehydrogenase, and 3-ketoacyl-CoA thiolase. The final product, acetyl-CoA, is used to generate ATP via the tricarboxylic acid cycle (Fig. 1).
Figure 1: The process of fatty acid oxidation. FA, fatty acid; CPT1, carnitine palmitoyltransferase 1; TCA, tricarboxylic acid cycle.
The CPT1A gene is located on 11q13.3 and is widely expressed in the brain, intestine, kidney, lung, ovary, pancreas, and spleen tissues (Jariwala et al., 2021). Owing to its critical role in FAO, dysregulation of CPT1A is involved in the progression of many diseases, such as obesity, psoriasis, type 2 diabetes, and cancer (Rufer et al., 2009; Schlaepfer et al., 2014; Schlaepfer and Joshi, 2020). CPT1A was reported to be overexpressed in many types of cancers, such as acute myeloid leukemia (Mao et al., 2021), high-grade glioma (Petővári et al., 2020), breast cancer (Jariwala et al., 2021) and ovarian cancer (Ghoneum et al., 2020), and to play important oncogenic roles in tumorigenesis.
Roles and Mechanisms of CPT1A in Tumorigenesis
CPT1A participates in nearly all aspects of tumorigenesis. During the progression of nonatrophic gastritis to gastric cancer (GC), the expression level of CPT1A was consistently increased (Dhondrup et al., 2022). A high-fat diet promotes the regeneration and tumorigenesis of intestinal tissues by activating the PPAR-CPT1A-FAO signaling pathway (Mana et al., 2021). CPT1A supported the growth of castration-resistant prostate cancer by increasing histone acetylation in an androgen-dependent manner (Joshi et al., 2019). In particular, blocking CPT1A with biodegradable polyethyleneimine-functionalized polyhydroxybutyrate nanoparticle (PHB-PEI NP)-delivered miR-124, whose binding and negative regulation of CPT1A hindered the tumorigenicity of prostate cancer cells (Conte et al., 2020). Therefore, we reviewed the most recent findings on the roles and regulatory mechanisms of CPT1A in tumorigenesis.
Proliferation and tumor growth
CPT1A is overexpressed in many types of cancers, and its high expression promotes the proliferation and tumor growth of cancer cells. In luminal breast cancer (BC), CPT1A was amplified and overexpressed, and both in vitro and in vivo experiments revealed that CPT1A potentiated the proliferation, colony formation, and mammosphere formation of luminal breast cancer cells by augmenting FAO (Jariwala et al., 2021). In acute myeloid leukemia (AML), CPT1A was highly expressed, and knockdown of CPT1A suppressed cell proliferation; additionally, it exerted synergistic therapeutic effects with ABT199 (BCL2 inhibitor) and ST1326 (CPT1A-selective inhibitor) on AML cells (Mao et al., 2021). In nasopharyngeal cancer cells and biopsy tissues, CPT1A was highly expressed, and CPT1A mechanically promoted tumor formation in vivo and proliferation and colony formation in vitro by enhancing FAO (Tang et al., 2022a). In gastric cancer (GC) cells and tissues, CPT1A was significantly upregulated, and its high expression activated FAO by increasing the NADP+/NADPH ratio and improved cell proliferation in GC (Wang et al., 2020b). CPT1A binds to and succinylates lactate dehydrogenase A (LDHA) at K222, suppresses lysosomal degradation of LDHA by blocking the interaction between LDHA and p62 and finally promotes GC cell proliferation and invasion (Li et al., 2020). Silencing CPT1A induced anoikis and decreased the suspension-cultured viability of high-grade serous ovarian cancer (HGSOC) cells in vitro; CPT1A inhibitor etomoxir markedly suppressed tumor progression in a patient-derived xenograft model (Sawyer et al., 2020). CPT1A was preferentially expressed in non-stem tumor cells compared with glioma stem cells (GSCs), and overexpression of CPT1A in GSCs promoted mitochondrial fusion and differentiation by phosphorylating dynamin-related protein 1 at Ser637 (Luo et al., 2021). Knockdown of CPT1A in an animal model of glioblastoma suppressed tumor growth and increased survival (Sperry et al., 2020). Depletion of CPT1A increased the cellular lipid concentration and inhibited the colony formation of pancreatic cancer cells and breast cancer cells (Guan et al., 2019).
CPT1A is involved in the oncogene-induced proliferation and tumor growth of cancer cells (Fig. 2). In HepG2 liver cancer cells, nuclear receptor subfamily 6, group A, member 1 decreased CPT1A expression and attenuated cell proliferation (Wang et al., 2019b). In GSCs, silencing peroxisome proliferator-activated receptor-alpha (PPARα) downregulated CPT1A and suppressed the proliferation and colony formation abilities of GSCs in vitro and tumorigenicity in vivo (Haynes et al., 2019). In breast cancer, the long noncoding RNA nuclear paraspeckle assembly transcript 1 (NEAT1)/miR-107 axis directly targeted and regulated CPT1A and ultimately promoted the growth and metastasis of breast cancer (Xiong et al., 2019). In hepatocellular carcinoma (HCC), miR-603 targeted and downregulated fatty acid-binding protein 1 (FABP1), increased downstream CPT1A expression and promoted the proliferation, migration, and invasion of cancer cells (Lin et al., 2021b). In summary, these findings suggested that overexpression of CPT1A promotes tumor growth, probably in an FAO-dependent manner.
Figure 2: Regulation of CPT1A in cancer cells. K, lysine; CPT1A, carnitine palmitoyltransferase 1A; LDHA, lactate dehydrogenase A; S100A10, S100 calcium-binding protein A10; NR6A1, nuclear receptor subfamily 6, group A, member 1; Ct-OATP1B3, cancer-type organic anion transporting polypeptide 1B3; PPAR, peroxisome proliferator-activated receptor.
High expression of CPT1A is correlated with the metastasis status of several types of cancers and confers improved migration and invasion abilities to tumor cells. Overexpression of CPT1A has been observed in breast cancer cell lines, tissues, and serum, and its high expression is significantly associated with metastasis and poor prognosis of breast cancer patients (Han et al., 2019; Jariwala et al., 2021; Tan et al., 2021). CPT1A is overexpressed in papillary thyroid cancer (PTC), and its increased expression is correlated with lymph node metastasis, TNM stage, and unfavorable outcome in PTC patients. CPT1A overexpression functionally promoted the migration of PTC cells (Lu et al., 2021a). CPT1A was overexpressed in GC tissues, and its overexpression potentiated the invasion and epithelial-mesenchymal transition (EMT) of GC cells (Wang et al., 2020b). As a succinyltransferase, CPT1A succinylated S100A10 at Lys47 and functionally promoted the invasion and migration of GC cells (Wang et al., 2019a).
Many oncogenic factors promote tumor cell metastasis by regulating CPT1A (Fig. 2). Mitochondrial fission, which is often increased in many types of cancers, promotes cell proliferation and metastasis, partially by regulating the PPARγ coactivator-1α ((PGC-1α)/PPARα/CPT1A) axis (Wu et al., 2022). In HGSOC, overexpression of cancer-type organic anion transporting polypeptide 1B3 (Ct-OATP1B3) upregulated CPT1A via insulin-like growth factor 2 mRNA-binding protein 2-dependent mRNA stability and promoted FAO, ultimately enhancing cancer cell migration and invasion (Huang et al., 2022). One study reported that miR-33b expression decreased in HGSOC tissues from metastatic omental cancer, owing to promoter hypermethylation, while miR-33b overexpression inhibited malignant phenotypes of ovarian cancer cells by suppressing fatty acid synthase-mediated de novo lipogenesis and CPT1A-mediated FAO and ultimately inhibited tumor metastasis (Wang et al., 2021). CPT1A participates in the miR-603/FABP1-induced migration and invasion of liver cancer cells (Lin et al., 2021b). The expression level of miR-328-3p is low in breast cancer, especially in metastatic breast cancer. MiR-328-3p directly regulates CPT1A and promotes breast cancer metastasis (Zeng et al., 2021).
In summary, on the one hand, CPT1A can promote tumor cell proliferation by activating FAO or succinylating the target genes as a succinyltransferase; on the other hand, CPT1A itself is regulated via transcription and mRNA stability during tumorigenesis (Fig. 2).
In addition to tumor cells, reprogramming of FAO and dysregulation of CPT1A also frequently occur in cancer-associated fibroblasts, adipocytes, immune cells, and other cells, eventually leading to a cancer-promoting microenvironment and immune suppression (Fig. 3). Long-term nicotine exposure improves the metastatic potential of lung cancer cells, and mechanically promotes the secretion of exosomal miR-4466 by N2-neutrophils and then enhances the metastatic ability of primary cancer cells in a CPT1A-dependent manner (Tyagi et al., 2022). Cancer-associated fibroblasts promote the migration and invasion of colon cancer cells by increasing CPT1A expression and enhancing FAO (Peng et al., 2021). Exosomal CD44, secreted by lymphatic metastatic gastric tumor cells, promoted lymph node metastasis of primary cancer cells by augmenting CPT1A-mediated FAO (Wang et al., 2022). In the tumor microenvironment, myeloid-derived suppressor cells express β2 adrenergic receptor and sequentially increase FAO by upregulating CPT1A, then secrete the immunosuppressor PGE2 and attenuate antitumor immunity (Mohammadpour et al., 2021). Importantly, the blockade of lipid oxidation using ranolazine inhibited tumor growth and led to antitumor immune activation, including increased infiltration of dendritic cells and CD8+ T cells in a CPT1A-dependent manner in a prostate cancer animal model (Guth et al., 2020).
Figure 3: Functional roles of CPT1A in the tumor microenvironment. MDSC, myeloid-derived suppressor cell; FAO, fatty acid oxidation; AR, adrenergic receptor; CPT1A, carnitine palmitoyltransferase 1A; PGE2, prostaglandin E2; STAT3, signal transducer and activator of transcription 3; CD44, CD44 molecule.
As one of the predominant components in the tumor microenvironment, adipocytes accelerate tumor growth and metastasis. Adipocytes can promote the invasion of colon tumor cells into surrounding adipose tissues. In one study, fatty acid or adipocyte treatment enhanced the invasion of colon cancer cells by activating the CPT1A-FAO-β-catenin signaling pathway (Xiong et al., 2020). Adipocyte-secreted apelin potentiated the metastasis of apelin receptor-expressing ovarian cancer cells by enhancing lipid oxidation and energy production in a CPT1A-dependent manner (Dogra et al., 2021). The adipocyte-secreted hormone leptin enhanced FAO in colorectal and breast cancer cells by upregulating CPT1A (Liu et al., 2019). Coculture of prostate cancer (PCa) cells with periprostatic adipose tissue cells increased the expression of CPT1A in these cells (Altuna-Coy et al., 2022).
Hypoxia plays a critical role in the progression of cancers and is also a major characteristic of the tumor microenvironment; thus, targeting hypoxia-regulated signaling pathways probably offers novel therapeutic strategies. In gastric adenocarcinoma, hypoxia leads to the induction of hypoxia-inducible factor (HIF)-1α and reduces the expression of FAO-related genes, including CPT1A, and CPT1A expression was negatively correlated with the level of HIF-1α in gastric adenocarcinoma tissues (Ezzeddini et al., 2021). Rios-Colon et al. (2021) reported that in PCa cells, CPT1A promoted the cell proliferation of cancer cells under hypoxic conditions in vitro; CPT1A also accelerated tumor growth in vivo with more hypoxic regions. Moreover, CPT1A overexpression in PCa cells significantly promoted the pathways of lipid catabolism, ER stress, and serine synthesis and repressed the androgen response (Joshi et al., 2020). Considering the crucial roles of CPT1A in the proliferation and migration of tumor cells and in processes related to the cancer-promoting microenvironment and immune suppression, targeting CPT1A will benefit tumor therapy because of its antitumor effects on both tumor cells and microenvironment cells.
Diagnostic and Prognostic Relevance and Therapeutic Applications
CPT1A is often reported to be highly expressed in several types of cancers and is identified as a potential diagnostic and prognostic cancer biomarker (Table 1). High expression of CPT1A was associated with pathological stage and grade in patients with GC and was closely associated with poor survival in GC, AML, and HGSOC (Sawyer et al., 2020; Wang et al., 2020b; Mao et al., 2021). The expression level of CPT1A is higher and was negatively correlated with tumor differentiation grades in canine mammary tumor tissues (Cacciola et al., 2020). High expression levels of CPT1A were significantly associated with the TNM stage and histological grade. Of note, the level of CPT1A is higher in the serum of breast cancer patients than in healthy patients (Tan et al., 2021). According to immunohistochemical analysis, the transcript and protein levels of CPT1A and pyruvate kinase muscle isozyme 2 decreased in cervical squamous cell carcinoma and also in HPV16-positive cervical intraepithelial neoplasia stages II–III tissues compared with HPV16-negative tissues (Abudula et al., 2020).
In contrast to most cancers, CPT1A expression was decreased in renal carcinoma (RC), and high expression of CPT1A was associated with good overall survival in RC (Zhao et al., 2019; Xu et al., 2020b). Overexpression of CPT1A suppressed malignant phenotypes of RC cells (Xu et al., 2020b). Another study also confirmed a decrease in CPT1A expression in clear cell renal carcinoma (ccRCC) and further revealed that overexpression of CPT1A attenuated lipid accumulation and tumor growth by regulating the PPARα/CD36 signaling pathway (Yang et al., 2022). STF-62247 increased the expression level of CPT1A in ccRCC and showed potential antitumor activities (Johnson et al., 2022). HIF could also downregulate CPT1A expression and diminish FAO in ccRCC (Tan and Welford, 2020), and LRPPRC was found to be the downstream regulator of CPT1A in ccRCC cells (Lu et al., 2021b).
Many clinical drugs and candidate drugs exert antitumor effects by suppressing FAO and/or CPT1A in tumor cells or cells in the tumor microenvironment. In a mouse model of lung cancer, high-dose dexamethasone, often used in the treatment of immunotherapy-induced autoimmune diseases, inhibited tumor progression and reduced the expression levels of FAO-related genes, including CPT1A (Xu et al., 2020a). An in vivo study showed that bezafibrate, an agonist of the PGC-1α/PPAR complex, promoted the infiltration of CD8+ T cells into lung tumor tissues and upregulated FAO-related genes, including CPT1A, in tumor cells (Wan et al., 2020). Anticancer roles of betulinic acid were reported in previous studies, and nanoliposomes loaded with betulinic acid attenuated the proliferation and glycolysis of CRC cells and decreased the CPT1A levels (Wang et al., 2020a). Withania somnifera root extract could decrease total free FAs and the expression level of CPT1A in prostate cancer cells (Kim et al., 2020). In diethylnitrosamine-induced HCC, both sterol O-acyltransferase 1 (SOAT1) and CPT1A were upregulated, and blocking these two exerted synergistic antitumor effects on HCC (Ren et al., 2021). The combination of CPT1A knockdown and blocking of cyclin-dependent kinase 9 exerted synergistic anticancer effects in PCa cells (Itkonen et al., 2019).
Resistance to chemotherapy is often a critical determinant of patient survival in several types of cancers, and blocking FAO/CPT1A shows promising therapeutic effects in chemoresistant tumors. Both in vitro and in patient-derived xenograft models, knockout and pharmacologic suppression of CPT1A sensitized HGSOC cells to platinum (Huang et al., 2021). In epithelial ovarian cancer, NK2 homeobox2-8 epigenetically suppressed key FAO genes, including CPT1, and importantly, blocking FAO using perhexiline enhanced the therapeutic effects of platinum (Zhu et al., 2019). The expression of CPT1A is higher in oxaliplatin-resistant than in oxaliplatin-sensitive colon cancer cells, and oxaliplatin treatment induces CPT1A expression. Both in vitro and in vivo experiments suggested that silencing CPT1A by siRNAs or etomoxir could sensitize drug-resistant tumor cells to oxaliplatin (Lin et al., 2021a). MAPK inhibitor treatment enhanced FAO in melanoma cells by regulating CPT1A, and elevated FAO allowed BRAFV600E cells which frequently acquired resistance to MAPK-targeted therapy to survive after MAPK inhibitor treatment. Blocking FAO, MAPK, and glycolysis synergistically repressed cancer cell growth both in vitro and in vivo (Aloia et al., 2019). CB-839 is an inhibitor of glutaminase, and in triple-negative breast cancer cells resistant to CB-839, the expression of CPT2 and activity of CPT1 were elevated. Blocking CPT1 and glutaminase synergistically inhibited the migration and proliferation of CB-839-resistant triple-negative breast cancer cells (Reis et al., 2019).
Radioresistant cancer cells often enhance metabolism to provide more energy to allow tumor cells to survive under adverse conditions. High PGC-1α expression was associated with poor survival in patients with nasopharyngeal carcinoma who received radiation therapy. CPT1A, which was transcriptionally activated by the PGC1α/CCAAT enhancer binding protein beta complex and sequentially improves FAO and participates in the radiation resistance of nasopharyngeal tumor cells (Du et al., 2019). The expression of CPT1A/CPT2 was higher in patients with recurrent breast cancer and correlated with unfavorable outcomes. Knockout of CPT1A/CPT2 repressed radiation-induced ERK phosphorylation and radioresistance in radioresistant breast cancer cells and radiation-derived breast cancer stem cells (Han et al., 2019).
In summary, CPT1A is dysregulated in many types of cancers and has the potential as a diagnostic and prognostic biomarker. Importantly, genetic or pharmacologic inhibition of CPT1A sensitizes tumor cells of several types of cancers to chemotherapy and radiotherapy. These findings suggested that CPT1A might be a candidate target for overcoming chemoresistance and radioresistance in tumors.
CPT1A is upregulated and associated with poor prognosis in the majority of cancers, such as GC, AML, HGSOC, and BC; in contrast, CPT1A is decreased and correlates with good prognosis in renal carcinoma (Zhao et al., 2019; Sawyer et al., 2020; Wang et al., 2020b; Mao et al., 2021; Peng et al., 2021; Tan et al., 2021). The reasons why both activation and inactivation of CPT1A and FAO can promote tumorigenesis in different types of cancers are still fairly unclear.
Blocking CPT1A or FAO has recently shown potential therapeutic effects in many types of cancers; in particular, this approach has shown promise for the treatment of chemoresistant and radioresistant cancer cells. Nevertheless, the roles and mechanisms of CPT1A and FAO in resistance to chemo- or radiotherapy urgently need to be investigated exhaustively, and future studies should focus on the application of CPT1A- or FAO-based methods in cancer therapy. Owing to its important roles in the cancer-promoting microenvironment and immune suppression, CPT1A might be developed as a new target for tumor immunotherapy.
Acknowledgement: None.
Funding Statement: This work was funded by the National Natural Science Foundation of China (Nos. 82160585 and 81760526) and the Yunnan Provincial Research Foundation for Basic Research, China (No. 202001AT070028).
Author Contributions: All authors contributed to the review conception and design. Ideas, study outline and modifications were provided by Zhi-Zhou Shi. The collection of references and the first draft of the manuscript were provided by Sheng-Jie Song. All authors have read and approved the final manuscript.
Availability of Data and Materials: All data generated or analyzed during this study are included in this paper.
Ethics Approval: Not Applicable.
Conflicts of Interest: The authors declare that they have no conflicts of interest to report regarding the present study.
References
Abudula A, Rouzi N, Xu L, Yang Y, Hasimu A (2020). Tissue-based metabolomics reveals potential biomarkers for cervical carcinoma and HPV infection. Bosnian Journal of Basic Medical Sciences 20: 78–87. https://doi.org/10.17305/bjbms.2019.4359 [Google Scholar] [PubMed] [CrossRef]
Adeva-Andany MM, Calvo-Castro I, Fernández-Fernández C, Donapetry-García C, Pedre-Piñeiro AM (2017). Significance of l-carnitine for human health. IUBMB Life 69: 578–594. https://doi.org/10.1002/iub.1646 [Google Scholar] [PubMed] [CrossRef]
Aloia A, Müllhaupt D, Chabbert CD, Eberhart T, Flückiger-Mangual S et al. (2019). A fatty acid oxidation-dependent metabolic shift regulates the adaptation of BRAF-mutated melanoma to MAPK inhibitors. Clinical Cancer Research 25: 6852–6867. https://doi.org/10.1158/1078-0432.CCR-19-0253 [Google Scholar] [PubMed] [CrossRef]
Altuna-Coy A, Ruiz-Plazas X, Sánchez-Martin S, Ascaso-Til H, Prados-Saavedra M, Alves-Santiago M, Bernal-Escoté X, Segarra-Tomás J, Chacón MR (2022). The lipidomic profile of the tumoral periprostatic adipose tissue reveals alterations in tumor cell’s metabolic crosstalk. BMC Medicine 20: 255. https://doi.org/10.1186/s12916-022-02457-3 [Google Scholar] [PubMed] [CrossRef]
Bonnefont JP, Djouadi F, Prip-Buus C, Gobin S, Munnich A, Bastin J (2004). Carnitine palmitoyltransferases 1 and 2: Biochemical, molecular and medical aspects. Molecular Aspects of Medicine 25: 495–520. https://doi.org/10.1016/j.mam.2004.06.004 [Google Scholar] [PubMed] [CrossRef]
Cacciola NA, Sgadari M, Petillo O, Margarucci S, Martano M, Cocchia N, Maiolino P, Restucci B (2020). Carnitine palmitoyltransferase 1 A expression profile in canine mammary tumors. Veterinary Journal 257: 105453. https://doi.org/10.1016/j.tvjl.2020.105453 [Google Scholar] [PubMed] [CrossRef]
Conte R, Valentino A, di Cristo F, Peluso G, Cerruti P, di Salle A, Calarco A (2020). Cationic polymer nanoparticles-mediated delivery of miR-124 impairs tumorigenicity of prostate cancer cells. International Journal of Molecular Sciences 21: 869. https://doi.org/10.3390/ijms21030869 [Google Scholar] [PubMed] [CrossRef]
Dhondrup R, Zhang X, Feng X, Lobsang D, Hua Q et al. (2022). Proteomic analysis reveals molecular differences in the development of gastric cancer. Evidence-Based Complementary and Alternative Medicine 2022: 8266544. https://doi.org/10.1155/2022/8266544 [Google Scholar] [PubMed] [CrossRef]
Dogra S, Neelakantan D, Patel MM, Griesel B, Olson A, Woo S (2021). Adipokine apelin/APJ pathway promotes peritoneal dissemination of ovarian cancer cells by regulating lipid metabolism. Molecular Cancer Research 19: 1534–1545. https://doi.org/10.1158/1541-7786.MCR-20-0991 [Google Scholar] [PubMed] [CrossRef]
Du Q, Tan Z, Shi F, Tang M, Xie L et al. (2019). PGC1α/CEBPB/CPT1A axis promotes radiation resistance of nasopharyngeal carcinoma through activating fatty acid oxidation. Cancer Science 110: 2050–2062. https://doi.org/10.1111/cas.14011 [Google Scholar] [PubMed] [CrossRef]
Ezzeddini R, Taghikhani M, Salek Farrokhi A, Somi MH, Samadi N, Esfahani A, Rasaee MJ (2021). Downregulation of fatty acid oxidation by involvement of HIF-1α and PPARγ in human gastric adenocarcinoma and related clinical significance. Journal of Physiology Biochemistry 77: 249–260. https://doi.org/10.1007/s13105-021-00791-3 [Google Scholar] [PubMed] [CrossRef]
Ghoneum A, Gonzalez D, Abdulfattah AY, Said N (2020). Metabolic plasticity in ovarian cancer stem cells. Cancers 12: 1267. https://doi.org/10.3390/cancers12051267 [Google Scholar] [PubMed] [CrossRef]
Glatz JFC, Luiken J (2018). Dynamic role of the transmembrane glycoprotein CD36 (SR-B2) in cellular fatty acid uptake and utilization. Journal of Lipid Research 59: 1084–1093. https://doi.org/10.1194/jlr.R082933 [Google Scholar] [PubMed] [CrossRef]
Guan L, Chen Y, Wang Y, Zhang H, Fan S et al. (2019). Effects of carnitine palmitoyltransferases on cancer cellular senescence. Journal of Cellular Physiology 234: 1707–1719. https://doi.org/10.1002/jcp.27042 [Google Scholar] [PubMed] [CrossRef]
Guth A, Monk E, Agarwal R, Bergman BC, Zemski-Berry KA, Minic A, Jordan K, Schlaepfer IR (2020). Targeting fat oxidation in mouse prostate cancer decreases tumor growth and stimulates anti-cancer immunity. International Journal of Molecular Sciences 21: 9660. https://doi.org/10.3390/ijms21249660 [Google Scholar] [PubMed] [CrossRef]
Han S, Wei R, Zhang X, Jiang N, Fan M et al. (2019). CPT1A/2-Mediated FAO enhancement-A metabolic target in radioresistant breast cancer. Frontiers in Oncology 9: 1201. https://doi.org/10.3389/fonc.2019.01201 [Google Scholar] [PubMed] [CrossRef]
Haynes HR, Scott HL, Killick-Cole CL, Shaw G, Brend T et al. (2019). shRNA-mediated PPARα knockdown in human glioma stem cells reduces in vitro proliferation and inhibits orthotopic xenograft tumour growth. Journal of Pathology 247: 422–434. https://doi.org/10.1002/path.5201 [Google Scholar] [PubMed] [CrossRef]
He W, Liang B, Wang C, Li S, Zhao Y et al. (2019). MSC-regulated lncRNA MACC1-AS1 promotes stemness and chemoresistance through fatty acid oxidation in gastric cancer. Oncogene 38: 4637–4654. https://doi.org/10.1038/s41388-019-0747-0 [Google Scholar] [PubMed] [CrossRef]
Huang D, Chowdhury S, Wang H, Savage SR, Ivey RG et al. (2021). Multiomic analysis identifies CPT1A as a potential therapeutic target in platinum-refractory, high-grade serous ovarian cancer. Cell Reports Medicine 2: 100471. https://doi.org/10.1016/j.xcrm.2021.100471 [Google Scholar] [PubMed] [CrossRef]
Huang Y, Du Y, Zheng Y, Wen C, Zou H, Huang J, Zhou H, Zhao H, Wu L (2022). Ct-OATP1B3 promotes high-grade serous ovarian cancer metastasis by regulation of fatty acid beta-oxidation and oxidative phosphorylation. Cell Death & Disease 13: 556. https://doi.org/10.1038/s41419-022-05014-1 [Google Scholar] [PubMed] [CrossRef]
Itkonen HM, Poulose N, Walker S, Mills IG (2019). CDK9 inhibition induces a metabolic switch that renders prostate cancer cells dependent on fatty acid oxidation. Neoplasia 21: 713–720. https://doi.org/10.1016/j.neo.2019.05.001 [Google Scholar] [PubMed] [CrossRef]
Jariwala N, Mehta GA, Bhatt V, Hussein S, Parker KA, Yunus N, Parker JS, Guo JY, Gatza ML (2021). CPT1A and fatty acid β-oxidation are essential for tumor cell growth and survival in hormone receptor-positive breast cancer. NAR Cancer 3: zcab035. https://doi.org/10.1093/narcan/zcab035 [Google Scholar] [PubMed] [CrossRef]
Jiang N, Xie B, Xiao W, Fan M, Xu S et al. (2022). Fatty acid oxidation fuels glioblastoma radioresistance with CD47-mediated immune evasion. Nature Communications 13: 1511. https://doi.org/10.1038/s41467-022-29137-3 [Google Scholar] [PubMed] [CrossRef]
Johnson M, Nowlan S, Sahin G, Barnett DA, Joy AP, Touaibia M, Cuperlovic-Culf M, Zofija Avizonis D, Turcotte S (2022). Decrease of intracellular glutamine by STF-62247 results in the accumulation of lipid droplets in von hippel-lindau deficient cells. Frontiers in Oncology 12: 841054. https://doi.org/10.3389/fonc.2022.841054 [Google Scholar] [PubMed] [CrossRef]
Joshi M, Kim J, D’Alessandro A, Monk E, Bruce K, Elajaili H, Nozik-Grayck E, Goodspeed A, Costello JC, Schlaepfer IR (2020). CPT1A over-expression increases reactive oxygen species in the mitochondria and promotes antioxidant defenses in prostate cancer. Cancers 12: 3431. https://doi.org/10.3390/cancers12113431 [Google Scholar] [PubMed] [CrossRef]
Joshi M, Stoykova GE, Salzmann-Sullivan M, Dzieciatkowska M, Liebman LN, Deep G, Schlaepfer IR (2019). CPT1A supports castration-resistant prostate cancer in androgen-deprived conditions. Cells 8: 1115. https://doi.org/10.3390/cells8101115 [Google Scholar] [PubMed] [CrossRef]
Kim SH, Singh KB, Hahm ER, Lokeshwar BL, Singh SV (2020). Withania somnifera root extract inhibits fatty acid synthesis in prostate cancer cells. Journal of Traditional Complementary Medicine 10: 188–197. https://doi.org/10.1016/j.jtcme.2020.02.002 [Google Scholar] [PubMed] [CrossRef]
Lee GS, Pan Y, Scanlon MJ, Porter CJH, Nicolazzo JA (2018). Fatty acid-binding protein 5 mediates the uptake of fatty acids, but not drugs, into human brain endothelial cells. Journal of Pharmaceutical Sciences 107: 1185–1193. https://doi.org/10.1016/j.xphs.2017.11.024 [Google Scholar] [PubMed] [CrossRef]
Li J, Xia Q, Di C, Li C, Si H et al. (2022). Tumor cell-intrinsic CD96 mediates chemoresistance and cancer stemness by regulating mitochondrial fatty acid β-oxidation. Advanced Science 10: e2202956. https://doi.org/10.1002/advs.202202956 [Google Scholar] [PubMed] [CrossRef]
Li X, Zhang C, Zhao T, Su Z, Li M et al. (2020). Lysine-222 succinylation reduces lysosomal degradation of lactate dehydrogenase a and is increased in gastric cancer. Journal of Experimental & Clinical Cancer Research 39: 172. https://doi.org/10.1186/s13046-020-01681-0 [Google Scholar] [PubMed] [CrossRef]
Lin YX, Wu XB, Zheng CW, Zhang QL, Zhang GQ, Chen K, Zhan Q, An FM (2021b). Mechanistic investigation on the regulation of FABP1 by the IL-6/miR-603 signaling in the pathogenesis of hepatocellular carcinoma. Biomed Research International 2021: 8579658. https://doi.org/10.1155/2021/8579658 [Google Scholar] [PubMed] [CrossRef]
Lin D, Zhang H, Liu R, Deng T, Ning T et al. (2021a). iRGD-modified exosomes effectively deliver CPT1A siRNA to colon cancer cells, reversing oxaliplatin resistance by regulating fatty acid oxidation. Molecular Oncology 15: 3430–3446. https://doi.org/10.1002/1878-0261.13052 [Google Scholar] [PubMed] [CrossRef]
Liu S, Lai J, Feng Y, Zhuo Y, Zhang H et al. (2023). Acetyl-CoA carboxylase 1 depletion suppresses de novo fatty acid synthesis and mitochondrial β-oxidation in castration-resistant prostate cancer cells. Journal of Biological Chemistry 299: 102720. https://doi.org/10.1016/j.jbc.2022.102720 [Google Scholar] [PubMed] [CrossRef]
Liu Q, Sun Y, Fei Z, Yang Z, Duan K, Zi J, Cui Q, Yu M, Xiong W (2019). Leptin promotes fatty acid oxidation and OXPHOS via the c-Myc/PGC-1 pathway in cancer cells. Acta Biochimica et Biophysica Sinica 51: 707–714. https://doi.org/10.1093/abbs/gmz058 [Google Scholar] [PubMed] [CrossRef]
Lu W, Che X, Qu X, Zheng C, Yang X et al. (2021b). Succinylation regulators promote clear cell renal cell carcinoma by immune regulation and RNA N6-methyladenosine methylation. Frontiers in Cell and Developmental Biology 9: 622198. https://doi.org/10.3389/fcell.2021.622198 [Google Scholar] [PubMed] [CrossRef]
Lu J, Zhang Y, Sun M, Ding C, Zhang L, Kong Y, Cai M, Miccoli P, Ma C, Yue X (2021a). Multi-omics analysis of fatty acid metabolism in thyroid carcinoma. Frontiers in Oncology 11: 737127. https://doi.org/10.3389/fonc.2021.737127 [Google Scholar] [PubMed] [CrossRef]
Luo M, Liu YQ, Zhang H, Luo CH, Liu Q et al. (2021). Overexpression of carnitine palmitoyltransferase 1A promotes mitochondrial fusion and differentiation of glioblastoma stem cells. Laboratory Investigation 102: 722–730. https://doi.org/10.1038/s41374-021-00724-0 [Google Scholar] [PubMed] [CrossRef]
Mana MD, Hussey AM, Tzouanas CN, Imada S, Barrera Millan Y et al. (2021). High-fat diet-activated fatty acid oxidation mediates intestinal stemness and tumorigenicity. Cell Reports 35: 109212. https://doi.org/10.1016/j.celrep.2021.109212 [Google Scholar] [PubMed] [CrossRef]
Mao S, Ling Q, Pan J, Li F, Huang S et al. (2021). Inhibition of CPT1a as a prognostic marker can synergistically enhance the antileukemic activity of ABT199. Journal of Translational Medicine 19: 181. https://doi.org/10.1186/s12967-021-02848-9 [Google Scholar] [PubMed] [CrossRef]
Mohammadpour H, MacDonald CR, McCarthy PL, Abrams SI, Repasky EA (2021). β2-adrenergic receptor signaling regulates metabolic pathways critical to myeloid-derived suppressor cell function within the TME. Cell Reports 37: 109883. https://doi.org/10.1016/j.celrep.2021.109883 [Google Scholar] [PubMed] [CrossRef]
Peng S, Chen D, Cai J, Yuan Z, Huang B et al. (2021). Enhancing cancer-associated fibroblast fatty acid catabolism within a metabolically challenging tumor microenvironment drives colon cancer peritoneal metastasis. Molecular Oncology 15: 1391–1411. https://doi.org/10.1002/1878-0261.12917 [Google Scholar] [PubMed] [CrossRef]
Petővári G, Dankó T, Krencz I, Hujber Z, Rajnai H, Vetlényi E, Raffay R, Pápay J, Jeney A, Sebestyén A (2020). Inhibition of metabolic shift can decrease therapy resistance in human high-grade glioma cells. Pathology & Oncology Research 26: 23–33. https://doi.org/10.1007/s12253-019-00677-2 [Google Scholar] [PubMed] [CrossRef]
Reddy JK, Hashimoto T (2001). Peroxisomal β-oxidation and peroxisome proliferator-activated receptor α: An adaptive metabolic system. Annual Review of Nutrition 21: 193–230. https://doi.org/10.1146/annurev.nutr.21.1.193 [Google Scholar] [PubMed] [CrossRef]
Reis LMD, Adamoski D, Ornitz Oliveira Souza R, Rodrigues Ascenção CF, Sousa de Oliveira KR et al. (2019). Dual inhibition of glutaminase and carnitine palmitoyltransferase decreases growth and migration of glutaminase inhibition-resistant triple-negative breast cancer cells. Journal of Biological Chemistry 294: 9342–9357. https://doi.org/10.1074/jbc.RA119.008180 [Google Scholar] [PubMed] [CrossRef]
Ren M, Xu H, Xia H, Tang Q, Bi F (2021). Simultaneously targeting SOAT1 and CPT1A ameliorates hepatocellular carcinoma by disrupting lipid homeostasis. Cell Death Discovery 7: 125. https://doi.org/10.1038/s41420-021-00504-1 [Google Scholar] [PubMed] [CrossRef]
Rios-Colon L, Kumar P, Kim S, Sharma M, Su Y et al. (2021). Carnitine palmitoyltransferase 1 regulates prostate cancer growth under hypoxia. Cancers 13: 6302. https://doi.org/10.3390/cancers13246302 [Google Scholar] [PubMed] [CrossRef]
Rufer AC, Thoma R, Hennig M (2009). Structural insight into function and regulation of carnitine palmitoyltransferase. Cellular and Molecular Life Sciences 66: 2489–2501. https://doi.org/10.1007/s00018-009-0035-1 [Google Scholar] [PubMed] [CrossRef]
Santos CR, Schulze A (2012). Lipid metabolism in cancer. FEBS Journal 279: 2610–2623. https://doi.org/10.1111/j.1742-4658.2012.08644.x [Google Scholar] [PubMed] [CrossRef]
Sawyer BT, Qamar L, Yamamoto TM, McMellen A, Watson ZL, Richer JK, Behbakht K, Schlaepfer IR, Bitler BG (2020). Targeting fatty acid oxidation to promote anoikis and inhibit ovarian cancer progression. Molecular Cancer Research 18: 1088–1098. https://doi.org/10.1158/1541-7786.MCR-19-1057 [Google Scholar] [PubMed] [CrossRef]
Schlaepfer IR, Joshi M (2020). CPT1A-mediated fat oxidation, mechanisms, and therapeutic potential. Endocrinology 161: 267. https://doi.org/10.1210/endocr/bqz046 [Google Scholar] [PubMed] [CrossRef]
Schlaepfer IR, Rider L, Rodrigues LU, Gijón MA, Pac CT et al. (2014). Lipid catabolism via CPT1 as a therapeutic target for prostate cancer. Molecular Cancer Therapeutics 13: 2361–2371. https://doi.org/10.1158/1535-7163.MCT-14-0183 [Google Scholar] [PubMed] [CrossRef]
Sperry J, Condro MC, Guo L, Braas D, Vanderveer-Harris N et al. (2020). Glioblastoma utilizes fatty acids and ketone bodies for growth allowing progression during ketogenic diet therapy. iScience 23: 101453. https://doi.org/10.1016/j.isci.2020.101453 [Google Scholar] [PubMed] [CrossRef]
Talley JT, Mohiuddin SS (2022). Biochemistry, fatty acid oxidation. In: StatPearls. Treasure Island: StatPearls Publishing. [Google Scholar]
Tan SK, Welford SM (2020). Lipid in renal carcinoma: Queen bee to target? Trends in Cancer 6: 448–450. https://doi.org/10.1016/j.trecan.2020.02.017 [Google Scholar] [PubMed] [CrossRef]
Tan Z, Zou Y, Zhu M, Luo Z, Wu T et al. (2021). Carnitine palmitoyl transferase 1A is a novel diagnostic and predictive biomarker for breast cancer. BMC Cancer 21: 409. https://doi.org/10.1186/s12885-021-08134-7 [Google Scholar] [PubMed] [CrossRef]
Tang M, Dong X, Xiao L, Tan Z, Luo X et al. (2022a). CPT1A-mediated fatty acid oxidation promotes cell proliferation via nucleoside metabolism in nasopharyngeal carcinoma. Cell Death & Disease 13: 331. https://doi.org/10.1038/s41419-022-04730-y [Google Scholar] [PubMed] [CrossRef]
Tang M, Wang J, Fan L (2022b). Comprehensive analysis of copy number variation, nucleotide mutation, and transcription level of PPAR pathway-related genes in endometrial cancer. PPAR Research 2022: 5572258. https://doi.org/10.1155/2022/5572258 [Google Scholar] [PubMed] [CrossRef]
Tyagi A, Wu SY, Sharma S, Wu K, Zhao D et al. (2022). Exosomal miR-4466 from nicotine-activated neutrophils promotes tumor cell stemness and metabolism in lung cancer metastasis. Oncogene 41: 3079–3092. https://doi.org/10.1038/s41388-022-02322-w [Google Scholar] [PubMed] [CrossRef]
Wan H, Xu B, Zhu N, Ren B (2020). PGC-1α activator-induced fatty acid oxidation in tumor-infiltrating CTLs enhances effects of PD-1 blockade therapy in lung cancer. Tumori Journal 106: 55–63. https://doi.org/10.1177/0300891619868287 [Google Scholar] [PubMed] [CrossRef]
Wang L, Li C, Song Y, Yan Z (2020b). Inhibition of carnitine palmitoyl transferase 1A-induced fatty acid oxidation suppresses cell progression in gastric cancer. Archives of Biochemistry Biophysics 696: 108664. https://doi.org/10.1016/j.abb.2020.108664 [Google Scholar] [PubMed] [CrossRef]
Wang Y, Wan X, Hao Y, Zhao Y, Du L, Huang Y, Liu Z, Wang Y, Wang N, Zhang P (2019b). NR6A1 regulates lipid metabolism through mammalian target of rapamycin complex 1 in HepG2 cells. Cell Communication and Signaling 17: 77. https://doi.org/10.1186/s12964-019-0389-4 [Google Scholar] [PubMed] [CrossRef]
Wang M, Yu W, Cao X, Gu H, Huang J et al. (2022). Exosomal CD44 transmits lymph node metastatic capacity between gastric cancer cells via YAP-CPT1A-mediated FAO reprogramming. Frontiers in Oncology 12: 860175. https://doi.org/10.3389/fonc.2022.860175 [Google Scholar] [PubMed] [CrossRef]
Wang G, Yu Y, Wang YZ, Zhu ZM, Yin PH, Xu K (2020a). Effects and mechanisms of fatty acid metabolism‐mediated glycolysis regulated by betulinic acid‐loaded nanoliposomes in colorectal cancer. Oncology Reports 44: 2595–2609. https://doi.org/10.3892/or.2020.7787 [Google Scholar] [PubMed] [CrossRef]
Wang X, Yung MMH, Sharma R, Chen F, Poon YT, Lam WY, Li B, Ngan HYS, Chan KKL, Chan DW (2021). Epigenetic silencing of miR-33b promotes peritoneal metastases of ovarian cancer by modulating the TAK1/FASN/CPT1A/NF-κB axis. Cancers 13: 4795. https://doi.org/10.3390/cancers13194795 [Google Scholar] [PubMed] [CrossRef]
Wang C, Zhang C, Li X, Shen J, Xu Y et al. (2019a). CPT1A-mediated succinylation of S100A10 increases human gastric cancer invasion. Journal of Cellular and Molecular Medicine 23: 293–305. https://doi.org/10.1111/jcmm.13920 [Google Scholar] [PubMed] [CrossRef]
Wright HJ, Hou J, Xu B, Cortez M, Potma EO, Tromberg BJ, Razorenova OV (2017). CDCP1 drives triple-negative breast cancer metastasis through reduction of lipid-droplet abundance and stimulation of fatty acid oxidation. Proceedings of the National Academy of Sciences of the United States of America 114: E6556–E6565. https://doi.org/10.1073/pnas.1703791114 [Google Scholar] [PubMed] [CrossRef]
Wu D, Yang Y, Hou Y, Zhao Z, Liang N, Yuan P, Yang T, Xing J, Li J (2022). Increased mitochondrial fission drives the reprogramming of fatty acid metabolism in hepatocellular carcinoma cells through suppression of Sirtuin 1. Cancer Communications 42: 37–55. https://doi.org/10.1002/cac2.12247 [Google Scholar] [PubMed] [CrossRef]
Xiong Y, Liu Z, Li Z, Wang S, Shen N, Xin Y, Huang T (2019). Long non‐coding RNA nuclear paraspeckle assembly transcript 1 interacts with microRNA‐107 to modulate breast cancer growth and metastasis by targeting carnitine palmitoyltransferase‐1. International Journal of Oncology 55: 1125–1136. https://doi.org/10.3892/ijo.2019.4869 [Google Scholar] [PubMed] [CrossRef]
Xiong X, Wen YA, Fairchild R, Zaytseva YY, Weiss HL, Evers BM, Gao T (2020). Upregulation of CPT1A is essential for the tumor-promoting effect of adipocytes in colon cancer. Cell Death & Disease 11: 736. https://doi.org/10.1038/s41419-020-02936-6 [Google Scholar] [PubMed] [CrossRef]
Xu Y, Wu G, Ma X, Li J, Ruan N, Zhang Z, Cao Y, Chen Y, Zhang Q, Xia Q (2020b). Identification of CPT1A as a prognostic biomarker and potential therapeutic target for kidney renal clear cell carcinoma and establishment of a risk signature of CPT1A-related genes. International Journal of Genomics 2020: 9493256. https://doi.org/10.1155/2020/9493256 [Google Scholar] [PubMed] [CrossRef]
Xu L, Xia H, Ni D, Hu Y, Liu J, Qin Y, Zhou Q, Yi Q, Xie Y (2020a). High-dose dexamethasone manipulates the tumor microenvironment and internal metabolic pathways in anti-tumor progression. International Journal of Molecular Sciences 21: 1846. https://doi.org/10.3390/ijms21051846 [Google Scholar] [PubMed] [CrossRef]
Yang H, Zhao H, Ren Z, Yi X, Zhang Q, Yang Z, Kuang Y, Zhu Y (2022). Overexpression CPT1A reduces lipid accumulation via PPARα/CD36 axis to suppress the cell proliferation in ccRCC. Acta Biochimica Biophysica Sinica 54: 220–231. https://doi.org/10.3724/abbs.2021023 [Google Scholar] [PubMed] [CrossRef]
Zeng F, Yao M, Wang Y, Zheng W, Liu S et al. (2021). Fatty acid β-oxidation promotes breast cancer stemness and metastasis via the miRNA-328-3p-CPT1A pathway. Cancer Gene Therapy 29: 383–395. https://doi.org/10.1038/s41417-021-00348-y [Google Scholar] [PubMed] [CrossRef]
Zhao Z, Liu Y, Liu Q, Wu F, Liu X, Qu H, Yuan Y, Ge J, Xu Y, Wang H (2019). The mRNA expression signature and prognostic analysis of multiple fatty acid metabolic enzymes in clear cell renal cell carcinoma. Journal of Cancer 10: 6599–6607. https://doi.org/10.7150/jca.33024 [Google Scholar] [PubMed] [CrossRef]
Zhu J, Wu G, Song L, Cao L, Tan Z, Tang M, Li Z, Shi D, Zhang S, Li J (2019). NKX2-8 deletion-induced reprogramming of fatty acid metabolism confers chemoresistance in epithelial ovarian cancer. eBioMedicine 43: 238–252. https://doi.org/10.1016/j.ebiom.2019.04.041 [Google Scholar] [PubMed] [CrossRef]
Cite This Article
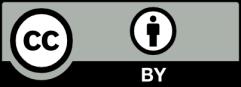
This work is licensed under a Creative Commons Attribution 4.0 International License , which permits unrestricted use, distribution, and reproduction in any medium, provided the original work is properly cited.