Open Access
REVIEW
Dental pulp stem cells and banking of teeth as a lifesaving therapeutic vista
1 Oral Health Institute, Department of Dentistry, Hamad Medical Corporation, Doha, Qatar
2 College of Dental Medicine, Qatar University, Doha, Qatar
3 Saveetha Dental College, Saveetha Institute of Medical and Technical Sciences (SIMATS), Chennai, India
4 Pushpagiri College of Dental Sciences, Medicity, Perumthuruthy, India
* Corresponding Author: SUKUMARAN ANIL. Email:
(This article belongs to the Special Issue: Cell-Based Regenerative Therapies)
BIOCELL 2023, 47(1), 71-80. https://doi.org/10.32604/biocell.2023.024334
Received 28 May 2022; Accepted 12 July 2022; Issue published 26 September 2022
Abstract
Exfoliated deciduous or an extracted healthy adult tooth can be used to harvest, process, and cryogenically preserve dental pulp stem cells. Future stem cell-based regenerative medicine methods could benefit significantly from these mesenchymal stem cells. Teeth serve as a substantial source of mesenchymal stem cells, otherwise disposed of as medical waste. Care should be taken to store this treasure trove of stem cells. Collective responsibility of patients, dentists, and physicians is necessary to ensure that this valuable resource is not wasted and that every possible dental pulp stem cell is available for use in the future. The dental pulp stem cells (DPSC) inside teeth represent a significant future source of stem cells for regenerative medicine procedures. This review describes the ontogeny, the laboratory processing and collection, and isolation methods of DPSC. This review also discusses currently available stem cell banking facilities and their potential use in regenerative medicine procedures in dental and general medical applications in the future.Keywords
Stem cell therapy and research have grown leaps and bounds in the last few decades. The exceptional scope of stem cells often necessitates and enhances the sources that generate them. Amidst diverse established stem cell sources, dental stem cells have been more compelling due to the ease of availability and manifest high plasticity and potentiality (Ferro et al., 2012; Potdar and Jethmalani, 2015). Because of their regenerative and multilineage differentiation potential, it is possible to isolate stem cells from many oral tissues, including the periodontal ligament, dental pulp, dental follicle, and oral mucosa. Dental stem cells (DSC) resemble mesenchymal stem cells (MSC). Most stem cells that are widely used in regenerative medicine can be effectively derived from DSC due to their heterogeneity and mesenchymal properties (Chalisserry et al., 2017).
Human dental pulp stem cells have been extensively explored for their multitudinous therapeutic possibilities (Ledesma-Martinez et al., 2016). Dental pulp stem cells originate from the neural crest cells, which makes them highly plastic than mesodermal derived bone marrow stem cells. In addition, neural crest cells have an inherent pluripotentialiality to differentiate into species-specific tissue developmental patterns (Zhai et al., 2019). DSCs thus are highly proliferative and can differentiate into various cell types, including odontoblasts, neural progenitors, osteoblasts, chondrocytes, and adipocytes (Galler et al., 2014).
Stem cell banking refers to the process of collecting, isolating, and preserving stem cells for use in regenerative medicine and therapy. Cryopreservation is a process of sustaining the viability of cells and tissues by freezing and storing them at sub-zero temperatures when biochemical reactions do not occur. This concise review highlights the main strategies related to the use of dental pulp stem cells, their characterization, storage, and stem cell and tooth banking.
Dental pulp stem cells (DPSCs)
DPSCs can be harvested from either extracted teeth or naturally exfoliated deciduous teeth. Exfoliated anterior deciduous teeth with root resorption of less than two-thirds are preferred for DPSC extraction. There are several sources of DSCs, namely the third molar, premolars, supernumerary, permanent, and deciduous teeth (Miura et al., 2003; Huang et al., 2008; Wang et al., 2012). DPSCs have the advantage of involving a minimally invasive process for retrieval and a capacity for long-term cryopreservation. DPSCs can be differentiated by modulating growth factors, transcriptional factors, extracellular matrix proteins, and receptor molecules into different cell types such as odontoblast, osteoblast, chondrocyte, cardiomyocytes, neuron cells, adipocyte, corneal epithelial cell, melanoma cell, and insulin-secreting Beta cells (Fig. 1) (Gronthos et al., 2000; Yu et al., 2010).
Figure 1: Multiple differentiation potential of dental pulp stem cells.
Therapeutic applications of dental pulp stem cells
DPSCs were found to be promising in many regenerative therapies such as: dental pulp regeneration (Iohara et al., 2013), bone tissue engineering (Akkouch et al., 2014), neurology (Ahmed Nel et al., 2016), angiogenesis/vasculogenesis (Gandia et al., 2008), endocrinology (Carnevale et al., 2013), and healing (Hirose et al., 2016) (Fig. 1). DPSCs are a unique type of mesenchymal stem cells of neural crest origin and express pluripotent stem cell markers such as Oct4, Nanog, Sox, and Klf4 with potent neurogenicity and more immunosuppressive activities (Kerkis et al., 2006; Mead et al., 2014). By modulating DPSCs with growth factors, transcriptional factors, extracellular matrix proteins, and receptor molecules, they can be differentiated into numerous cell types, such as odontoblast, osteoblast, chondrocyte, cardiomyocytes, neuron cells, adipocyte, corneal epithelial cell, melanoma cell, and insulin-secreting beta cells (Potdar and Jethmalani, 2015).
Dental pulp stem cells (DPSCs) secrete a dentin matrix and differentiate into odontoblasts by stimulating specific cytokines or growth factors (Couble et al., 2000). Stem cell-based dental pulp regeneration can maintain tooth vitality and regenerate a complete dental pulp containing blood vessels, nerves, and newly formed dentin in both ectopic and in situ regeneration (Xuan et al., 2018; Meng et al., 2020). Furthermore, the possibility of regenerating whole teeth with functional properties by using scaffold and DPSCs cells is being carried out successfully (Huang et al., 2021). Hence, stem cell-mediated root regeneration may be an alternative to replacing teeth (Meng et al., 2020).
Dental stem cells are a potential source for the engineering of bone tissue, and the osteogenic differentiation capacity of DPSCS has been demonstrated both in vitro and in vivo (Akkouch et al., 2014; Yasui et al., 2017). Dexamethasone, L-ascorbic acid, and β-glycerol phosphate supplementation have been shown to extensively induce osteogenic differentiation. Due to their osteogenic potential, DPSCs have been combined with 3D polymeric scaffolds to generate bone-like hard tissues (Hao et al., 2022; Xie et al., 2022).
DPSC can stimulate long-term regenerations of nerves in the damaged spinal cord and can be effectively used to manage neurodegenerative diseases, including Alzheimer’s (Ahmed Nel et al., 2016). Compared to MSCs, DPSCs exhibit a higher proliferation rate, higher expression of trophic factors, potent neuroprotective effects, and neuro-supportive properties in vitro and in vivo, demonstrating their potential for use in stroke treatment (Nito et al., 2022) and the treatment of pathologies caused by insufficient angiogenesis. The potential of DPSCs to treat myocardial infarction and ischemia has been confirmed (Loo et al., 2014; Augustine et al., 2021). DPSC is an excellent source of stem cells for repairing infarcted myocardium and regenerative therapy for ischemic heart disease (Gandia et al., 2008).
DPSCs have been differentiated to generate hepatocyte-like cells with acquired hepatocyte functions, such as glycogen storage and urea production (Ishkitiev et al., 2012). Chen et al. (2016) performed hepatic differentiation of DPSCs using cryopreserved dental pulp tissue. DPSCs have the potential to differentiate into pancreatic cell lineage resembling islet-like cell aggregates. Human DPSCs express genes related to pancreatic β-cell development and function under appropriate stimuli, including insulin, pancreatic, and duodenal homeobox-1 (Carnevale et al., 2013). Transplantation of islet-like cell clusters derived from human DPSC in diabetic mice showed reversal of hyperglycemia (Kanafi et al., 2013). The current research is promising, and DPSCs could be used as an autologous stem cell therapy for diabetes (Hata et al., 2020).
Vital deciduous and permanent teeth without any infections serve as a viable source of dental pulp stem cells (Perry et al., 2008; Temmerman et al., 2010). Chen et al. (2011) showed that the DPSCs could be retrieved from infected vital human teeth encompassing periodontitis, supernumerary teeth, inflamed malpositioned teeth, root resorption, and pericoronitis, as well as fractured teeth. Several other studies also confirmed that infected vital teeth could be a source of DPSCs (Pereira et al., 2012; Malekfar et al., 2016; Werle et al., 2016; Tsai et al., 2017). Greater root resorption might have a negative impact on the viability of the stem cells (Bernardi et al., 2011). Challenge lies in the preservation of the cells from degradation, which begins immediately after extraction or exfoliation. In addition, teeth with diseases such as periapical abscesses, tumors, or cysts must not be considered for stem cell recovery (Cordeiro et al., 2008). Most tooth banking services recommend and prefer professionally extracted deciduous teeth when they become mobile over naturally exfoliated ones. This is because deciduous teeth that become very mobile naturally through trauma, or disease, often have a compromised blood supply and may not be suitable for DPSC recovery. Harvested teeth should be washed with bactericidal solutions to minimize contamination. The tooth is stored at 4°C in phosphate buffer saline (PBS) during laboratory transfer to avoid dehydration and necrosis. The stem cell isolation should be performed within 120 h after extraction. Perry et al. (2008) demonstrated that DPSC cultures were established from 80% of extracted human third molars processed within 24 h of extraction. DPSC cultures can be initiated for at least 120 h post-extraction if teeth are stored at 48°C in a variety of collection or transport media. A longer time frame may lower post-thawing cell recovery efficiency. Exfoliated anterior deciduous teeth with less than two-thirds root resorption are preferred for DSC extraction. Increased root resorption reduces the stem cell viability from human exfoliated deciduous teeth (SHED). Dental pulp tissues from wisdom teeth and first premolars extracted for orthodontic purposes are the common sources of DPSC in adults. DPSC harvesting and isolation efficiency is better from an immature permanent tooth with incomplete root formation (Bernardi et al., 2011).
The exfoliated or extracted tooth shows no signs of post-removal bleeding, and the pulp appears greyish. It indicates a necrotic pulp tissue and hence is not ideal for DPSC retrieval. However, some services accept naturally exfoliated or home-extracted deciduous teeth. The requirement for acceptance is the presence of bleeding after extraction/exfoliation as evidence of a vital blood supply (Zeitlin, 2020). BioEden® (Austin, TX) provides a safe home collection kit to store and transport the tooth to the tooth banking facility.
Once the tooth is extracted or exfoliated, it must be preserved from the naturally occurring degradation process. Keeping the tooth hydrated and preventing necrosis of the pulp tissue are essential concerns. The tooth must be immediately placed in a vial with a transport medium. The choice of the transport medium is a matter of preference of the banking facility. However, immersing it in water is not recommended. An isotonic solution mimicking physiological conditions would be ideal (Moazami et al., 2012). PBS and Hanks buffered saline solution (HBSS) supplemented with nutrients are widely used solutions for transport. Save-a-Tooth™ kit contains HBSS as storage media (Zeitlin, 2020). BioEden® has pasteurized bovine milk as its transport medium in its home collection kit. It has been a transport medium for avulsed teeth over the years because of its biocompatibility and neutral pH (Andersson et al., 2012). The maximum permissible time from harvesting to processing at the storage facility should not exceed 40 h, beyond which the tooth may lose its viability of the pulp tissue.
Once the vital tooth is transported to the laboratory, it is cleaned and disinfected, and the crown of the tooth is separated to reach the pulp chamber and pulp tissue. The separation of the crown from the root(s) must be done carefully to avoid damage to the soft tissue within. Diamond burs with coolants or special forceps can be used in the cementoenamel junction at the cervical third of the tooth to open the pulp chamber. Alternately, the teeth could be mechanically fractured and the dental pulp gently isolated with forceps, without cutting or drilling. This could prevent any damaging effects on the viability of DPSCs (Hilkens et al., 2013). Next, the dental pulp tissue is separated from the root canal walls and the pulp chamber. It is easier to isolate the dental pulp tissue from the pulp chamber in teeth with a large opening at the apical foramen region, like resorbed deciduous teeth or incompletely formed roots of permanent teeth. These large open areas pave a pathway through which the pulp tissue can be retrieved without splitting the tooth. This is a much safer technique to obtain the pulp tissue without damaging it. After the pulp tissue is accessed and retrieved, it is cut into numerous small pieces for further expansion (Pilbauerova and Suchanek, 2018).
Isolation of dental pulp stem cells
Stem cells account for 1% of the total pulp cell population; hence efficient isolation and expansion are imperative (Smith, 2005). Dental pulp retrieval is done by gently separating the root region to reach the pulp chamber. The pulp tissue can be retrieved either through the root apex of the resorbed roots of deciduous teeth or through the immature open apex of the permanent teeth. The pulp tissue is harvested with a sterile barbed broach or by slicing the dentinal wall with the diamond disc in the presence of a coolant (Fig. 2). The other approach is opening the pulp chamber by splitting the tooth at the cementoenamel junction. The retrieved pulp tissue is minced into small pieces and stored (Spath et al., 2010; Ferro et al., 2012).
Figure 2: A schematic of the dental pulp stem cell isolation and storage processes.
The DPSCs can be isolated either by the enzymatic digestion (ED) technique or the outgrowth from tissue explants (OG) technique, also known as the spontaneous growth technique (Gronthos et al., 2000; Spath et al., 2010). Researchers have tested both techniques to evaluate the efficiency of cell proliferation rate or unchanged morphological and phenotypic properties of isolated stem cells. Some researchers have reported that the stem cells isolated by the OG technique have a lower proliferation rate and weaker stem cell marker expression (Karamzadeh et al., 2012). Others found that DPSCs isolated by the ED technique displayed a higher proliferation rate, differentiation, and expressed other surface markers when compared with the cells isolated by the OG technique. In contrast, dental pulp immature stem cells (IDPSCs) from deciduous teeth isolated using the OG method showed that the cell culture promoted the selective proliferation of IDPSCs, preventing premature differentiation (Kerkis et al., 2006).
In this technique, the minced pulp tissue pieces are submerged in a cocktail of two or more enzymes. ED allows segregation of single-cell suspensions by enzymatic digestion of minced primary pulp tissues. Single cells in suspension are obtained by physically straining the digested tissue, and the cells are then allowed to grow in culture media. There are several digestion protocols developed for pulp tissue isolation. However, the combination of 3 mg/mL collagenase type I and 4 mg/mL dispase has been used widely (Rodas-Junco and Villicana, 2017). Other enzyme combinations and mechanical isolation of DPSCs could be assessed for the increase in survival of the cells during the isolation process. Successful isolation with a blend was achieved with 0.1% collagenase IV in 0.25% trypsin EDTA and 5 mg/mL collagenase type II in 2.5 mg/mL dispase (Lucaciu et al., 2015). A mechanical device, MACSTM dissociator (Miltenyi, Biotec), could be used to isolate the DPSCs mechanically.
Outgrowth from tissue explants technique
The OG technique is simple and fast and involves the direct placement of the minced pulp tissues, of about 1 to 2 mm3 size, into culture plates with a proliferation medium. This allows them to grow from the pulp tissue explants (Hilkens et al., 2013). The culture plates are incubated at 37°C in 5% CO2. The proliferation medium changes after outgrowths are observed, and the cells can be sub-cultured further (Karamzadeh et al., 2012). Stem cells obtained at the end of the subculture from both techniques are assessed for their survival rates and expression of cell markers by running them through flow cytometric analysis. When the cells are deemed sterile and viable with the desired surface markers, they are ready for cryogenic preservation.
Purification of dental pulp stem cells
Purification of DPSC is pivotal for isolating highly regenerative cells. This requires the utility of specific cell markers. Markers LNGFR and THY-1 are routinely used to label MSCs as they can validate the phenotypic characters (Yasui et al., 2017). Cell selection is performed either with fluorescence or magnetic-activated cell sorting methods. In addition to the markers mentioned above, CD73, CD90, CD105, CD29, CD44, CD166, STRO-1, CD13, CD146, CD117, SSEA-3, SSEA-4, TRA-1-60, TRA-1-81, NANOG, Oct4, Sox2, and HLA-DR surface antigens are also used (Rodas-Junco and Villicana, 2017). The need for multiple markers for selection is due to the heterogeneous nature of the DSCs. To date, there is no single marker that can delineate the subpopulations. Hoechst dye has exhibited reasonable success in delineating DSCs with angiogenic potential; however, it is not advocated in therapeutic cell isolation due to its carcinogenic and growth-inhibiting properties.
Cryopreservation of dental pulp stem cells
Once stem cells have been extracted via enzyme digestion or tissue outgrowth, they are ready for cryopreservation to maintain their vitality, phenotypic stability, and differentiation capacity over the long term. This procedure is crucial as the differentiation capacity changes with cell passaging (Yu et al., 2010). Cryopreservation is the application of low temperatures to preserve the structural and functional integrity of cells and tissues. During the process, the aqueous phase changes to a solid form of ice. The stability of the cells and tissue is maintained by storage at an ultra-low temperature of –196°C (Hunt, 2019). While most banking facilities prefer to store only the isolated cells, there are facilities, like a store-a tooth, which freezes a part of the original pulp tissue along with the isolated cells (Zeitlin, 2020). The ideal cell concentration to be cryopreserved is reported to be 1.0–1.5 × 106 cell concentration to reap a maximum number of viable cells after thawing to produce optimal results (Woods et al., 2009).
While considering cryopreservation, the rate at which the cells are cooled must be addressed with caution. This is because of the water content within the cells; freezing the cells rapidly causes the formation of ice crystals because of insufficient time for the water to leave the cells, which can cause rupture of the cell membranes and eventual structural and mechanical damage to the cells. On the other hand, freezing them slowly can cause dehydration of the cells, which increases the cytosol concentration of solutes leading to alteration in the osmotic gradient, thereby damaging the cell. (Zhurova et al., 2010). To overcome these temperature-related cellular damages, cryoprotectants (CPAs) are added to the cryopreservative medium, which prevents the formation of the ice crystals due to optimal freezing rate and reduces freeze-thaw points (Stolzing et al., 2012). CPAs can be grouped under two categories based on their molecular weights and mode of action. The low molecular weight CPAs exert their action intracellularly by penetration through the cell membrane and preventing the initiation of ice crystal nucleation and growth (Zambelli et al., 1998). The high molecular weight CPAs do not cross the cell membrane but facilitate dehydration of the cell, reduce intracellular ice crystal formation, and maintain the integrity of the cell membrane (Stolzing et al., 2012). The most widely used CPA is dimethyl sulfoxide (DMSO), a low molecular weight agent, documented for its efficacy in cellular protection and maintenance of cellular viability (Naaldijk et al., 2012). Once the cells are suspended in the appropriate cocktail of the cryopreservation medium and CPA, they are transferred into high-density polypropylene cryovials and stored in liquid nitrogen (LN2) canisters.
The process of cryopreservation
The isolated cells are suspended in a preservation medium consisting of growth factors and a cryoprotective agent capable of inhibiting crystal growth and dehydration. Then, the cells are transferred to specialized high-density polypropylene cryovials and frozen containing LN2. For safe storage of the stem cells and their future clinical use, the choice of the cryopreservation method is crucial. Therefore, various freezing methods are used, such as rapid freezing (vitrification), controlled-rate freezing (slow freezing), uncontrolled freezing, and magnetic freezing.
Rapid freezing method (vitrification)
Vitrification is a simple process of cooling cells at ultrahigh speeds of freezing. This eliminates the formation of ice crystals and creates a glass-like (vitreous) state of the freezing solution. It involves direct contact between the vitrification solution containing the cryoprotectant agents and LN2. The vitrification process allows cells to be placed directly into the cryoprotectant, stored within small carriers, and then plunged into LN2 (Liebermann et al., 2002). Although the process uses a high concentration of cryoprotectant, rapid cooling reduces the toxicity induced by the CPA. The cooling rate is dependent on the structure of vitrification carriers and the manipulation procedures. Generally, an increased cooling rate is achieved by plunging small-volume samples inside small vitrification carriers, such as miniStraw, cryoleaf, and cryoloop, directly into LN2 (Li et al., 2010). Therefore, only small volumes of samples and a relatively high volume of LN2 for each vitrification cycle are needed, limiting the potential use of this technique.
While the viability and growth rate of post-vitrification thawing of stem cells have been studied, only a few studies have reported on the viability of dental stem cells following the vitrification method. One study compared the cell survival, and proliferation of DPSCs cryopreserved by vitrification and controlled-rate freezing method in 5% and 10% DMSO for six months. The dental stem cells cryopreserved by the controlled rate cryopreservation showed significant improvement in cell survival and proliferation rate. However, the vitrified stem cells did not regrow after thawing. Additionally, irrespective of the six-month DMSO concentration, cryopreserved DPSCs could not grow after thawing (Huynh et al., 2017).
Controlled-rate cryopreservation
In this cryopreservation method, the freezing process and storage comprise a gradual freezing process and storage in LN2 (−196°C). The temperature at which the cells are cooled per minute can be controlled. The two widely used controlled-rate freezing methods are the slow freezing rate and the ultra-slow freezing rate cryopreservation. The rationale behind these methods is the elimination or reduction of cellular damage caused due to ice crystal formation or dehydration that can be encountered while freezing cells. The optimal rate of slow freezing at which the viability and growth potential of the cells can be maintained and with minimal or no damage is 1°C to 2°C per min, and that of ultra-slow freezing is 0.3°C to 0.6°C per min (Pilbauerova et al., 2021). Some studies reported that these methods show better results in post-thaw cell viability and growth rate than the vitrification technique (Huynh et al., 2017). However, other studies claim no differences in cell viability and growth potential of the post-thaw cells obtained from either the controlled rate freezing or vitrification method (Naaldijk et al., 2012). The uncontrolled-rate cryopreservation is a more straightforward and cost-effective method when compared to controlled-rate freezing. The cells are suspended in the cryopreserved medium with CPA and precooled to 4°C. This is followed by freezing the cells at −80°C or storing them directly into LN2 (Davies et al., 2014). Studies have shown that DPSCs cryopreserved by this method have shown good viability and growth rate of the cells (Kumar et al., 2015).
Cryopreservation can also be done using an uncontrolled rate freezing protocol. In this method, CPA and stem cell samples are cooled to 4°C and placed directly into a freezer at 80°C or LN2 (Davies et al., 2014). Uncontrolled rate freezing is an efficient method for long-term cryopreservation of DPSCs without any post-thaw effect on cell viability, multipotency, proliferation, and differentiation (Kumar et al., 2015).
Magnetic cryopreservation involves subjecting cells and tissues to a low-intensity magnetic field which reduces the freezing point by up to 6°C to 7°C. The concept applies the utilization of a slow controlled-rate magnetic field of 0.01 mT in a programmed freezer. The magnetic field lowers water accumulation during freezing, thereby eliminating the chances of ice formation and undesirable electric current generation (Lee et al., 2012). Freezing occurs at a slower pace of 0.5 °C/min up to −32°C, after which the frozen cells are stored in a −150°C freezer (Lee et al., 2012). The advantage of magnetic cryopreservation over other methods is that thawed cells present superior biological activity due to a low concentration of DMSO (3%) and minimal pre-equilibration time. Magnetic cryopreserved DPSCs have improved cell survival and more expansion and differentiation than other methods. Additionally, they also exhibit better cell surface markers (Lee et al., 2012).
Cryopreservation of whole teeth
Isolation of DPSC from freshly extracted or exfoliated teeth is possible, although contamination and damage during cryopreservation might affect the viability of dental pulp stem cells. Also, the isolation and expansion of DPSC in a laboratory environment is expensive, time-consuming, and poses a risk of contamination and spontaneous differentiation (Lindemann et al., 2014). Therefore, preservation as a whole tooth and deferring isolation and expansion when required have been proven to be more viable methods.
Cryopreservation maintains the viability of cells by freezing and storing them at sub-zero temperatures, thereby preventing biochemical reactions (Mullen and Critser, 2007). A tissue that undergoes cryopreservation could sustain freezing injuries during the freezing or thawing process. Most likely, the injuries are due to ice crystals formed either within the cells (intracellularly) or outside the cells (extracellularly). Injuries to the cells can also happen due to the release of reactive oxygen species (ROS), which can trigger apoptosis and cause cell death (Mazzilli et al., 1995). A cryoprotective agent (CPA) is added to the freezing medium to protect the cells from sustaining these injuries. CPAs slow the growth and formation of ice crystals, thereby inhibiting the binding of intracellular nuclei (Stolzing et al., 2012).
The idea of preserving whole teeth cryogenically rather than the DPSCs has been explored. The rationale behind the concept is to isolate and expand the DPSCs when the future demands it. Both deciduous and permanent teeth have been attempted to be preserved cryogenically, and the process has been met with limitations. Isolation and expansion of DPSCs can be costly and time-consuming with risks of contamination and spontaneous differentiation (Lindemann et al., 2014). When the whole tooth is cryopreserved, it has to undergo a “thawing” process when retrieving the DPSCs from it. A low number of viable cells, morphological changes, and low rate of isolation are some of the drawbacks faced while dealing with DPSCs obtained from cryopreserved whole teeth. The morphology of the roots of cryopreserved teeth might play a role in these defects. When the roots are fully formed, like in a permanent tooth or deciduous tooth with no root resorption, the extent of penetration of the CPA through the tiny apical foramen into the pulp tissue is less. This could reduce the protective effect of the CPA on the pulp during the freezing and thawing processes. Hence one would expect a better proliferation rate of the DPSCs in teeth with open root apices of permanent teeth or significantly resorbed deciduous teeth because of the ease of penetration of the CPA into the pulpal area and the protective effect it could render. However, this was not the case, and the proliferation rate of the DPSCs from such teeth remained low compared to non-cryopreserved teeth (Pilbauerova and Suchanek, 2018). One study reported that the percentage of viable stem cells retrieved from cryopreserved and thawed teeth is as low as 30% compared to that from non-cryopreserved teeth (61%) (Lindemann et al., 2014). Morphological change in a more rounded cytoplasm was found in DPSCs of cryopreserved whole teeth compared to the spindle-shaped ones of the non-cryopreserved teeth. A novel technique of laser piercing on the tooth to create micro-holes was attempted to enhance the CPA’s penetration. However, the DPSCs showed a similar isolation rate as those of the non-cryopreserved teeth (Gioventu et al., 2012). Based on the data published regarding cryopreservation of whole teeth, it can be concluded that there are no significant benefits of cryopreserving whole teeth. There are, however, several disadvantages of this process.
A water bath maintained at 37°C is the most standard method, which is excellent at removing the ice crystals without any cell damage. The only drawback is that the contact with water may lead to a high bacterial or fungicidal contamination risk. The application of dry heat is another technique comparable to those thawed using the standard water procedure. The cryoprotective agent must be washed out of the cryovials after thawing to avoid any cytotoxic effect (Triana et al., 2013).
DSCs have a wide range of therapeutic benefits and applications in regenerative medicine. The preservation of DSCs necessitated a “tooth bank” for long-term preservation and retrieval for future regenerative therapies. This facility is essential for storing varied cells obtained from dental pulp, tooth apex, periodontal ligament, follicle, and gingiva. Cryopreservation or magnetic freezing are used to preserve dental stem cells (Oh et al., 2005; Tamaoki et al., 2010; Gioventu et al., 2012).
Despite tooth bank’s enormous sources and prospects, the concept is yet to gain complete global acceptance. Developed countries have established tooth banks with extended centers in other countries. BioEden® (Austin, Texas, USA), Store-A-Tooth® (Provia Laboratories, Littleton, Massachusetts, USA), and StemSave® (Stemsave Inc., New York, USA) are a few of the prominent tooth banking facilities which also have their branches in the United Kingdom and Thailand.
Tooth banking is a process that entails tooth collection, stem cell isolation, and storage. Banking dental pulp stem cells is a relatively new concept that is rapidly gaining traction in several countries. The establishment of the world’s first tooth bank is credited to Hiroshima University in Japan in 2004, and the company was renamed “Three Brackets” in 2005. In 2008 (Kaku et al., 2007), Hiroshima University and Taipei Medical University established Taipei’s first tooth bank as a source for natural implants. BioEden®, National Dental Pulp Laboratory, Oothy, Stemodontics, Stem Save, Store-A-Tooth/Provia Labs, and Tooth bank/Cryopoint are just a few of the companies located in the United States of America that offer dental stem cell storage services, with several of them expanding internationally (Zeitlin, 2020).
BioEden® has laboratories in the United Kingdom and Thailand and is expanding globally. The Norwegian Tooth Bank, established in 2008 in collaboration with the Norwegian Institute of Public Health’s Department of Clinical Dentistry & University of Bergen, has a collection of 100,000 children as part of “The Norwegian Mother and Child Cohort Study.” In this cohort study, Tooth Bank is a sub-project. This is intended to grow into the world’s largest tooth bank for research purposes. Cryocord is Southeast Asia’s premier stem cell bank, established in Malaysia in 2003. Cryocord operates nationwide with regional offices in the Philippines, Brunei, Indonesia, and Thailand. Cryocord offers tooth banking services in addition to stem cell storage. DentCell, based in Mexico, Future Cell Biobank, based in the United Kingdom, Mothercell, Reelabs, Stemade, and Store Your Cells, based in India, are a few other companies that provide dental pulp stem cell banking (Zeitlin, 2020).
The dental pulp stem cells are capable of extensive proliferation and differentiation, which make them an essential resource of stem cells for regeneration and repair of diseased and injured organs and tissues. DPSC can be obtained from extracted vital teeth or deciduous teeth after shedding. Combining growth factors, small molecules, scaffold materials, and optimal culture conditions are essential for the proper differentiation of DPSCs and the release of their regenerative potential. The comprehensive knowledge regarding the biological characteristics and origin of DPSCs, their identification and harvesting, critical aspects related to their characterization, their multilineage differentiation potential, and current clinical applications would contribute to their potential use in regenerative medicine. Regarding cell banking processes, cryopreservation media and methods must be developed to retain the characteristics and viability of these cells for future application. Both cryopreserved intact tooth and dental stem cells can be used at a later stage in individual regenerative cell and tissue therapy. Even though significant progress has been made in the isolation, expansion, and preservation of DPSCs, further optimization is still required, particularly to follow more homogenous rules to limit phenotypic variance and address safety concerns to generate DPSCs for cellular treatment. Further research and clinical trials should focus on developing novel techniques to advance the use of DPSCs in stem cell-based therapy.
Author Contribution: The authors confirm their contribution to the paper as follows: Study conception and design: SA, RR, and VKS; draft manuscript preparation SA, RR, NGT, JMG, and VKS. All authors reviewed the results and approved the final version of the manuscript.
Ethics Approval: Not applicable.
Funding Statement: The authors received no specific funding for this study.
Conflicts of Interest: The authors declare that they have no conflicts of interest to report regarding the present study.
References
Ahmed Nel M, Murakami M, Hirose Y, Nakashima M (2016). Therapeutic potential of dental pulp stem cell secretome for alzheimer’s disease treatment: An in vitro study. Stem Cells International 2016: 8102478. DOI 10.1155/2016/8102478. [Google Scholar] [CrossRef]
Akkouch A, Zhang Z, Rouabhia M (2014). Engineering bone tissue using human dental pulp stem cells and an osteogenic collagen-hydroxyapatite-poly (L-lactide-co-epsilon-caprolactone) scaffold. Journal of Biomaterials Applications 28: 922–936. DOI 10.1177/0885328213486705. [Google Scholar] [CrossRef]
Andersson L, Andreasen JO, Day P, Heithersay G, Trope M, Diangelis AJ, Kenny DJ, Sigurdsson A, Bourguignon C, Flores MT et al. (2012). International association of dental traumatology guidelines for the management of traumatic dental injuries: 2. Avulsion of permanent teeth. Dental Traumatology 28: 88–96. DOI 10.1111/j.1600-9657.2012.01125.x. [Google Scholar] [CrossRef]
Augustine R, Dan P, Hasan A, Khalaf IM, Prasad P, Ghosal K, Gentile C, McClements L, Maureira P (2021). Stem cell-based approaches in cardiac tissue engineering: Controlling the microenvironment for autologous cells. Biomedicine and Pharmacotherapy 138: 111425. DOI 10.1016/j.biopha.2021.111425. [Google Scholar] [CrossRef]
Bernardi L, Luisi SB, Fernandes R, Dalberto TP, Valentim L, Bogo Chies JA, Medeiros Fossati AC, Pranke P (2011). The isolation of stem cells from human deciduous teeth pulp is related to the physiological process of resorption. Journal of Endodontics 37: 973–979. DOI 10.1016/j.joen.2011.04.010. [Google Scholar] [CrossRef]
Carnevale G, Riccio M, Pisciotta A, Beretti F, Maraldi T, Zavatti M, Cavallini GM, La Sala GB, Ferrari A, de Pol A (2013). In vitro differentiation into insulin-producing beta-cells of stem cells isolated from human amniotic fluid and dental pulp. Digestive and Liver Disease 45: 669–676. DOI 10.1016/j.dld.2013.02.007. [Google Scholar] [CrossRef]
Chalisserry EP, Nam SY, Park SH, Anil S (2017). Therapeutic potential of dental stem cells. Journal of Tissue Engineering 8: 2041731417702531. DOI 10.1177/2041731417702531. [Google Scholar] [CrossRef]
Chen YK, Huang AH, Chan AW, Lin LM (2016). Human dental pulp stem cells derived from cryopreserved dental pulp tissues of vital extracted teeth with disease demonstrate hepatic-like differentiation. Journal of Tissue Engineering and Regenerative Medicine 10: 475–485. DOI 10.1002/term.1763. [Google Scholar] [CrossRef]
Chen YK, Huang AH, Chan AW, Shieh TY, Lin LM (2011). Human dental pulp stem cells derived from different cryopreservation methods of human dental pulp tissues of diseased teeth. Journal of Oral Pathology and Medicine 40: 793–800. DOI 10.1111/j.1600-0714.2011.01040.x. [Google Scholar] [CrossRef]
Cordeiro MM, Dong Z, Kaneko T, Zhang Z, Miyazawa M, Shi S, Smith AJ, Nor JE (2008). Dental pulp tissue engineering with stem cells from exfoliated deciduous teeth. Journal of Endodontics 34: 962–969. DOI 10.1016/j.joen.2008.04.009. [Google Scholar] [CrossRef]
Couble ML, Farges JC, Bleicher F, Perrat-Mabillon B, Boudeulle M, Magloire H (2000). Odontoblast differentiation of human dental pulp cells in explant cultures. Calcified Tissue International 66: 129–138. DOI 10.1007/PL00005833. [Google Scholar] [CrossRef]
Davies OG, Smith AJ, Cooper PR, Shelton RM, Scheven BA (2014). The effects of cryopreservation on cells isolated from adipose, bone marrow and dental pulp tissues. Cryobiology 69: 342–347. DOI 10.1016/j.cryobiol.2014.08.003. [Google Scholar] [CrossRef]
Ferro F, Spelat R, Beltrami AP, Cesselli D, Curcio F (2012). Isolation and characterization of human dental pulp derived stem cells by using media containing low human serum percentage as clinical grade substitutes for bovine serum. PLoS One 7: e48945. DOI 10.1371/journal.pone.0048945. [Google Scholar] [CrossRef]
Galler KM, Eidt A, Schmalz G (2014). Cell-free approaches for dental pulp tissue engineering. Journal of Endodontics 40: S41–S45. DOI 10.1016/j.joen.2014.01.014. [Google Scholar] [CrossRef]
Gandia C, Arminan A, Garcia-Verdugo JM, Lledo E, Ruiz A et al. (2008). Human dental pulp stem cells improve left ventricular function, induce angiogenesis, and reduce infarct size in rats with acute myocardial infarction. Stem Cells 26: 638–645. DOI 10.1634/stemcells.2007-0484. [Google Scholar] [CrossRef]
Gioventu S, Andriolo G, Bonino F, Frasca S, Lazzari L, Montelatici E, Santoro F, Rebulla P (2012). A novel method for banking dental pulp stem cells. Transfusion and Apheresis Science 47: 199–206. DOI 10.1016/j.transci.2012.06.005. [Google Scholar] [CrossRef]
Gronthos S, Mankani M, Brahim J, Robey PG, Shi S (2000). Postnatal human dental pulp stem cells (DPSCs) in vitro and in vivo. Proceedings of the National Academy of Sciences 97: 13625–13630. DOI 10.1073/pnas.240309797. [Google Scholar] [CrossRef]
Hao X, Li D, Zhang D, Jia L (2022). Microarray analysis of long non-coding RNAs related to osteogenic differentiation of human dental pulp stem cells. Journal of Dental Sciences 17: 733–743. DOI 10.1016/j.jds.2021.10.014. [Google Scholar] [CrossRef]
Hata M, Omi M, Kobayashi Y, Nakamura N, Miyabe M et al. (2020). Transplantation of human dental pulp stem cells ameliorates diabetic polyneuropathy in streptozotocin-induced diabetic nude mice: The role of angiogenic and neurotrophic factors. Stem Cell Research & Therapy 11: 236. DOI 10.1186/s13287-020-01758-9. [Google Scholar] [CrossRef]
Hilkens P, Gervois P, Fanton Y, Vanormelingen J, Martens W, Struys T, Politis C, Lambrichts I, Bronckaers A (2013). Effect of isolation methodology on stem cell properties and multilineage differentiation potential of human dental pulp stem cells. Cell and Tissue Research 353: 65–78. DOI 10.1007/s00441-013-1630-x. [Google Scholar] [CrossRef]
Hirose Y, Yamamoto T, Nakashima M, Funahashi Y, Matsukawa Y, Yamaguchi M, Kawabata S, Gotoh M (2016). Injection of dental pulp stem cells promotes healing of damaged bladder tissue in a rat model of chemically induced cystitis. Cell Transplantation 25: 425–436. DOI 10.3727/096368915X689523. [Google Scholar] [CrossRef]
Huang AH, Chen YK, Lin LM, Shieh TY, Chan AW (2008). Isolation and characterization of dental pulp stem cells from a supernumerary tooth. Journal of Oral Pathology and Medicine 37: 571–574. DOI 10.1111/j.1600-0714.2008.00654.x. [Google Scholar] [CrossRef]
Huang X, Li Z, Liu A, Liu X, Guo H, Wu M, Yang X, Han B, Xuan K (2021). Microenvironment influences odontogenic mesenchymal stem cells mediated dental pulp regeneration. Frontiers in Physiology 12: 656588. DOI 10.3389/fphys.2021.656588. [Google Scholar] [CrossRef]
Hunt CJ (2019). Technical considerations in the freezing, low-temperature storage and thawing of stem cells for cellular therapies. Transfusion Medicine and Hemotherapy 46: 134–150. DOI 10.1159/000497289. [Google Scholar] [CrossRef]
Huynh NC, Le SH, Doan VN, Ngo LTQ, Tran HLB (2017). Simplified conditions for storing and cryopreservation of dental pulp stem cells. Archives of Oral Biology 84: 74–81. DOI 10.1016/j.archoralbio.2017.09.002. [Google Scholar] [CrossRef]
Iohara K, Murakami M, Takeuchi N, Osako Y, Ito M, Ishizaka R, Utunomiya S, Nakamura H, Matsushita K, Nakashima M (2013). A novel combinatorial therapy with pulp stem cells and granulocyte colony-stimulating factor for total pulp regeneration. Stem Cells Translational Medicine 2: 521–533. DOI 10.5966/sctm.2012-0132. [Google Scholar] [CrossRef]
Ishkitiev N, Yaegaki K, Imai T, Tanaka T, Nakahara T, Ishikawa H, Mitev V, Haapasalo M (2012). High-purity hepatic lineage differentiated from dental pulp stem cells in serum-free medium. Journal of Endodontics 38: 475–480. DOI 10.1016/j.joen.2011.12.011. [Google Scholar] [CrossRef]
Kaku M, Kamata H, Kawata T, Tai M, Kohon S, Motokawa M, Tohma Y, Fujita T, Ohtani J, Tsuka N (2007). Cryopreservation of PDL cells by use of program freezer with magnetic field for teeth banking. Dentistry in Japan 43: 82–86. [Google Scholar]
Kanafi MM, Rajeshwari YB, Gupta S, Dadheech N, Nair PD, Gupta PK, Bhonde RR (2013). Transplantation of islet-like cell clusters derived from human dental pulp stem cells restores normoglycemia in diabetic mice. Cytotherapy 15: 1228–1236. DOI 10.1016/j.jcyt.2013.05.008. [Google Scholar] [CrossRef]
Karamzadeh R, Eslaminejad MB, Aflatoonian R (2012). Isolation, characterization and comparative differentiation of human dental pulp stem cells derived from permanent teeth by using two different methods. Journal of Visualized Experiments 10: 3791–4372. DOI 10.3791/4372. [Google Scholar] [CrossRef]
Kerkis I, Kerkis A, Dozortsev D, Stukart-Parsons GC, Gomes Massironi SM, Pereira LV, Caplan AI, Cerruti HF (2006). Isolation and characterization of a population of immature dental pulp stem cells expressing OCT-4 and other embryonic stem cell markers. Cells Tissues Organs 184: 105–116. DOI 10.1159/000099617. [Google Scholar] [CrossRef]
Kumar A, Bhattacharyya S, Rattan V (2015). Effect of uncontrolled freezing on biological characteristics of human dental pulp stem cells. Cell and Tissue Banking 16: 513–522. DOI 10.1007/s10561-015-9498-5. [Google Scholar] [CrossRef]
Ledesma-Martinez E, Mendoza-Nunez VM, Santiago-Osorio E (2016). Mesenchymal stem cells derived from dental pulp: A review. Stem Cells International 2016: 4709572. DOI 10.1155/2016/4709572. [Google Scholar] [CrossRef]
Lee SY, Huang GW, Shiung JN, Huang YH, Jeng JH, Kuo TF, Yang JC, Yang WC (2012). Magnetic cryopreservation for dental pulp stem cells. Cells Tissues Organs 196: 23–33. DOI 10.1159/000331247. [Google Scholar] [CrossRef]
Li T, Mai Q, Gao J, Zhou C (2010). Cryopreservation of human embryonic stem cells with a new bulk vitrification method. Biology of Reproduction 82: 848–853. DOI 10.1095/biolreprod.109.080713. [Google Scholar] [CrossRef]
Liebermann J, Nawroth F, Isachenko V, Isachenko E, Rahimi G, Tucker MJ (2002). Potential importance of vitrification in reproductive medicine. Biology of Reproduction 67: 1671–1680. DOI 10.1095/biolreprod.102.006833. [Google Scholar] [CrossRef]
Lindemann D, Werle SB, Steffens D, Garcia-Godoy F, Pranke P, Casagrande L (2014). Effects of cryopreservation on the characteristics of dental pulp stem cells of intact deciduous teeth. Archives of Oral Biology 59: 970–976. DOI 10.1016/j.archoralbio.2014.04.008. [Google Scholar] [CrossRef]
Loo ZX, Kunasekaran W, Govindasamy V, Musa S, Abu Kasim NH (2014). Comparative analysis of cardiovascular development related genes in stem cells isolated from deciduous pulp and adipose tissue. Scientific World Journal 2014: 186508. DOI 10.1155/2014/186508. [Google Scholar] [CrossRef]
Lucaciu O, Soritau O, Gheban D, Ciuca DR, Virtic O et al. (2015). Dental follicle stem cells in bone regeneration on titanium implants. BMC Biotechnology 15: 114. DOI 10.1186/s12896-015-0229-6. [Google Scholar] [CrossRef]
Malekfar A, Valli KS, Kanafi MM, Bhonde RR (2016). Isolation and characterization of human dental pulp stem cells from cryopreserved pulp tissues obtained from teeth with irreversible pulpitis. Journal of Endodontics 42: 76–81. DOI 10.1016/j.joen.2015.10.001. [Google Scholar] [CrossRef]
Mazzilli F, Rossi T, Sabatini L, Pulcinelli FM, Rapone S, Dondero F, Gazzaniga PP (1995). Human sperm cryopreservation and reactive oxygen species (ROS) production. Acta Europaea Fertilitatis 26: 145–148. [Google Scholar]
Mead B, Logan A, Berry M, Leadbeater W, Scheven BA (2014). Paracrine-mediated neuroprotection and neuritogenesis of axotomised retinal ganglion cells by human dental pulp stem cells: Comparison with human bone marrow and adipose-derived mesenchymal stem cells. PLoS One 9: e109305. DOI 10.1371/journal.pone.0109305. [Google Scholar] [CrossRef]
Meng H, Hu L, Zhou Y, Ge Z, Wang H, Wu CT, Jin J (2020). A sandwich structure of human dental pulp stem cell sheet, treated dentin matrix, and matrigel for tooth root regeneration. Stem Cells and Development 29: 521–532. DOI 10.1089/scd.2019.0162. [Google Scholar] [CrossRef]
Miura M, Gronthos S, Zhao M, Lu B, Fisher LW, Robey PG, Shi S (2003). SHED: Stem cells from human exfoliated deciduous teeth. Proceedings of the National Academy of Sciences 100: 5807–5812. DOI 10.1073/pnas.0937635100. [Google Scholar] [CrossRef]
Moazami F, Mirhadi H, Geramizadeh B, Sahebi S (2012). Comparison of soymilk, powdered milk, Hank’s balanced salt solution and tap water on periodontal ligament cell survival. Dental Traumatology 28: 132–135. DOI 10.1111/j.1600-9657.2011.01054.x. [Google Scholar] [CrossRef]
Mullen SF, Critser JK (2007). The science of cryobiology. Cancer Treatment and Research 138: 83–109. DOI 10.1007/978-0-387-72293-1. [Google Scholar] [CrossRef]
Naaldijk Y, Staude M, Fedorova V, Stolzing A (2012). Effect of different freezing rates during cryopreservation of rat mesenchymal stem cells using combinations of hydroxyethyl starch and dimethylsulfoxide. BMC Biotechnology 12: 49. DOI 10.1186/1472-6750-12-49. [Google Scholar] [CrossRef]
Nito C, Suda S, Nitahara-Kasahara Y, Okada T, Kimura K (2022). Dental-pulp stem cells as a therapeutic strategy for ischemic stroke. Biomedicines 10: 737. DOI 10.3390/biomedicines10040737. [Google Scholar] [CrossRef]
Oh YH, Che ZM, Hong JC, Lee EJ, Lee SJ, Kim J (2005). Cryopreservation of human teeth for future organization of a tooth bank–A preliminary study. Cryobiology 51: 322–329. DOI 10.1016/j.cryobiol.2005.08.008. [Google Scholar] [CrossRef]
Pereira LO, Rubini MR, Silva JR, Oliveira DM, Silva IC, Pocas-Fonseca MJ, Azevedo RB (2012). Comparison of stem cell properties of cells isolated from normal and inflamed dental pulps. International Endodontic Journal 45: 1080–1090. DOI 10.1111/j.1365-2591.2012.02068.x. [Google Scholar] [CrossRef]
Perry BC, Zhou D, Wu X, Yang FC, Byers MA, Chu TM, Hockema JJ, Woods EJ, Goebel WS (2008). Collection, cryopreservation, and characterization of human dental pulp-derived mesenchymal stem cells for banking and clinical use. Tissue Engineering Part C: Methods 14: 149–156. DOI 10.1089/ten.tec.2008.0031. [Google Scholar] [CrossRef]
Pilbauerova N, Schmidt J, Soukup T, Koberova Ivancakova R, Suchanek J (2021). The effects of cryogenic storage on human dental pulp stem cells. International Journal of Molecular Sciences 22: 4432. DOI 10.3390/ijms22094432. [Google Scholar] [CrossRef]
Pilbauerova N, Suchanek J (2018). Cryopreservation of dental stem cells. Acta Medica (Hradec Kralove) 61: 1–7. DOI 10.14712/18059694.2018.16. [Google Scholar] [CrossRef]
Potdar PD, Jethmalani YD (2015). Human dental pulp stem cells: Applications in future regenerative medicine. World Journal of Stem Cells 7: 839–851. DOI 10.4252/wjsc.v7.i5.839. [Google Scholar] [CrossRef]
Rodas-Junco BA, Villicana C (2017). Dental pulp stem cells: Current advances in isolation, expansion and preservation. Tissue Engineering and Regenerative Medicine 14: 333–347. DOI 10.1007/s13770-017-0036-3. [Google Scholar] [CrossRef]
Smith AJ, Patel M, Graham L, Sloan AJ, Cooper PR (2005). Dentine regeneration: key roles for stem cells and molecular signalling. Oral Biosciences & Medicine 2: 127–132. [Google Scholar]
Spath L, Rotilio V, Alessandrini M, Gambara G, de Angelis L et al. (2010). Explant-derived human dental pulp stem cells enhance differentiation and proliferation potentials. Journal of Cellular and Molecular Medicine 14: 1635–1644. DOI 10.1111/j.1582-4934.2009.00848.x. [Google Scholar] [CrossRef]
Stolzing A, Naaldijk Y, Fedorova V, Sethe S (2012). Hydroxyethylstarch in cryopreservation-mechanisms, benefits and problems. Transfusion and Apheresis Science 46: 137–147. DOI 10.1016/j.transci.2012.01.007. [Google Scholar] [CrossRef]
Tamaoki N, Takahashi K, Tanaka T, Ichisaka T, Aoki H et al. (2010). Dental pulp cells for induced pluripotent stem cell banking. Journal of Dental Research 89: 773–778. DOI 10.1177/0022034510366846. [Google Scholar] [CrossRef]
Temmerman L, Beele H, Dermaut LR, Van Maele G, De Pauw GA (2010). Influence of cryopreservation on the pulpal tissue of immature third molars in vitro. Cell and Tissue Banking 11: 281–289. DOI 10.1007/s10561-009-9148-x. [Google Scholar] [CrossRef]
Triana E, Ortega S, Azqueta C, Pomares H, Valdivia E, Duarte R, Massuet L, Martin-Henao GA (2013). Thawing of cryopreserved hematopoietic progenitor cells from apheresis with a new dry-warming device. Transfusion 53: 85–90. DOI 10.1111/j.1537-2995.2012.03669.x. [Google Scholar] [CrossRef]
Tsai AI, Hong HH, Lin WR, Fu JF, Chang CC, Wang IK, Huang WH, Weng CH, Hsu CW, Yen TH (2017). Isolation of mesenchymal stem cells from human deciduous teeth pulp. BioMed Research International 2017: 2851906. DOI 10.1155/2017/2851906. [Google Scholar] [CrossRef]
Wang X, Sha XJ, Li GH, Yang FS, Ji K, Wen LY, Liu SY, Chen L, Ding Y, Xuan K (2012). Comparative characterization of stem cells from human exfoliated deciduous teeth and dental pulp stem cells. Archives of Oral Biology 57: 1231–1240. DOI 10.1016/j.archoralbio.2012.02.014. [Google Scholar] [CrossRef]
Werle SB, Lindemann D, Steffens D, Demarco FF, de Araujo FB, Pranke P, Casagrande L (2016). Carious deciduous teeth are a potential source for dental pulp stem cells. Clinical Oral Investigations 20: 75–81. DOI 10.1007/s00784-015-1477-5. [Google Scholar] [CrossRef]
Woods EJ, Perry BC, Hockema JJ, Larson L, Zhou D, Goebel WS (2009). Optimized cryopreservation method for human dental pulp-derived stem cells and their tissues of origin for banking and clinical use. Cryobiology 59: 150–157. DOI 10.1016/j.cryobiol.2009.06.005. [Google Scholar] [CrossRef]
Xie H, Lin Y, Fang F (2022). Glycogen synthase kinase-3beta inhibitor promotes the migration and osteogenic differentiation of rat dental pulp stem cells via the beta-catenin/PI3K/Akt signaling pathway. Journal of Dental Sciences 17: 802–810. DOI 10.1016/j.jds.2021.09.035. [Google Scholar] [CrossRef]
Xuan K, Li B, Guo H, Sun W, Kou X et al. (2018). Deciduous autologous tooth stem cells regenerate dental pulp after implantation into injured teeth. Science Translational Medicine 10: aaf3227. DOI 10.1126/scitranslmed.aaf3227. [Google Scholar] [CrossRef]
Yasui T, Mabuchi Y, Morikawa S, Onizawa K, Akazawa C, Nakagawa T, Okano H, Matsuzaki Y (2017). Isolation of dental pulp stem cells with high osteogenic potential. Inflammation and Regeneration 37: 8. DOI 10.1186/s41232-017-0039-4. [Google Scholar] [CrossRef]
Yu J, He H, Tang C, Zhang G, Li Y, Wang R, Shi J, Jin Y (2010). Differentiation potential of STRO-1+ dental pulp stem cells changes during cell passaging. BMC Cell Biology 11: 32. DOI 10.1186/1471-2121-11-32. [Google Scholar] [CrossRef]
Zambelli A, Poggi G, da Prada G, Pedrazzoli P, Cuomo A, Miotti D, Perotti C, Preti P, Robustelli della Cuna G (1998). Clinical toxicity of cryopreserved circulating progenitor cells infusion. Anticancer Research 18: 4705–4708. [Google Scholar]
Zeitlin BD (2020). Banking on teeth-stem cells and the dental office. Biomedical Journal 43: 124–133. DOI 10.1016/j.bj.2020.02.003. [Google Scholar] [CrossRef]
Zhai Q, Dong Z, Wang W, Li B, Jin Y (2019). Dental stem cell and dental tissue regeneration. Frontiers of Medicine 13: 152–159. DOI 10.1007/s11684-018-0628-x. [Google Scholar] [CrossRef]
Zhurova M, Woods EJ, Acker JP (2010). Intracellular ice formation in confluent monolayers of human dental tem ells and membrane damage. Cryobiology 61: 133–141. DOI 10.1016/j.cryobiol.2010.06.007. [Google Scholar] [CrossRef]
Cite This Article
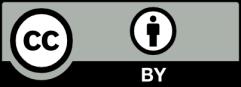