Open Access
ARTICLE
Quercetin induced HepG2 cells apoptosis through ATM/JNK/STAT3 signaling pathways
1 Center Laboratory, Guangzhou Women and Children’s Medical Center, Guangzhou Medical University, Guangzhou, 510120, China
2 College of Light Industry and Food Sciences, Innovation Research Institute of Modern Agricultural Engineering, Zhongkai University of Agriculture and
Engineering, Guangzhou, 510225, China
* Corresponding Authors: Hao Dong, ; Yinghua Li,
# These authors contributed equally to this work
BIOCELL 2023, 47(1), 187-194. https://doi.org/10.32604/biocell.2022.023030
Received 06 April 2022; Accepted 29 June 2022; Issue published 26 September 2022
Abstract
Liver cancer is the seventh most common malignant tumor in the world and is the second highest cause of death due to cancer. Quercetin, a flavonoid with low toxicity, widely exists in various fruits and vegetables. It has the potential to be a therapeutic agent against various cancers. This study aimed to demonstrate the anti-tumor effect of quercetin on HepG2 cells. Quercetin suppressed the HepG2 cell proliferation in a dose-dependent manner in cell viability assay. Induction of cell apoptosis was confirmed by apoptotic cells population (sub-G1 peak) detected by flow cytometer. A decrease in mitochondrial membrane potential and caspase-3 activation were also demonstrated in this study. Furthermore, quercetin induced HepG2 cell apoptosis through ROS-mediated phosphorylated ataxia-telangiectasia mutated, c-Jun Nterminal kinases, signal transducer, and activator of transcription 3 (STAT-3), and Bax signaling pathways. These results suggest that quercetin has the potential to become an effective drug against the tumor.Keywords
Liver cancer is the seventh most common malignant tumor globally and ranks second in deaths due to cancer. Liver cancer cases are increasing most speedily, with an annual increase of 2%–3% from 2007 to 2016 in the USA. The 5-year survival rate has not increased remarkably in the past 20 years In China (Siegel et al., 2020). Various etiologies and inducing factors are explicit (Forner et al., 2018). Hepatitis B infection, hepatitis C infection, alcohol, diabetes and nonalcoholic fatty liver disease are the leading risk factors (Kakehashi et al., 2020). In the clinic, ultrasound and alpha-fetoprotein are recommended for the preliminary screening of liver cancer. However, their underuse limits the detection rate (Yang and Heimbach, 2020). Regarding the therapy, hepatocellular carcinoma (HCC) is insensitive to radiotherapy and chemotherapy (Sheng et al., 2018). Less than 30% of HCC patients are applicable for surgical treatment. Though radiofrequency ablation (RFA) and microwave ablation (MWA) are applied to the treatment of HCC, RFA is still confined to its heat dissipation effect (Han et al., 2020), and the MWA’s long diameter damages the normal liver tissue (Lin et al., 2016). Neither chemical and surgical treatment nor interventional therapy could provide a good prognosis. Therefore, there is an urgency to find an effective way to treat HCC.
Quercetin, 3,3′,4′,5,7-pentahydroxyflavone (C15H10O7), is a kind of antioxidant (Shabbir et al., 2021). Owing to being natural ingredients of the polyphenols that exist in various fruits and vegetables, it is widely accessible (Reyes-Farias and Carrasco-Pozo, 2019). Previous researchers have found that quercetin has lots of functions, such as anti-inflammatory, anti-oxidation, anti-diabetic, and anti-allergic properties (Badolato et al., 2017). Quercetin also potentially has anti-tumor properties (Tang et al., 2020). It inhibits the proliferation of colon cancer (Lin et al., 2020) and can downregulate the expression of MMP-2/-9 protein to restrict the hyperplasia of breast cancer cells named MCF-7 (Ozkan and Bakar-Ates, 2020). Song et al. (2020a) found that the PI3K/Akt/mTOR signaling pathway could be blocked by quercetin for curing human prostate cell carcinoma PC-3 cells. In addition, compared to other present chemical drugs, quercetin was a fascinating anticancer compound owing to its low toxicity (Rauf et al., 2018). In vivo toxicological experiments confirmed that low oral doses of quercetin did not cause significant changes in morphological, behavioral, hematological, serum biochemical, and histopathological parameters in Swiss albino mice, while high oral doses showed mild toxicity (Pal and Tripathi, 2020).
Reactive Oxygen Species (ROS) is a collective term for a series of derivatives of molecular oxygen, including superoxide anion radicals, singlet oxygen, hydroxyl radicals, and so on (Zhu et al., 2016). Although ROS occurs as a common attribute of aerobic life (Sies and Jones, 2020), the redox imbalance is associated with many diseases, such as prostate cancer, Alzheimer’s disease, cardiac and pulmonary diseases (Climent et al., 2020; Tonnies and Trushina, 2017; Ward et al., 2018). It is also related to many intracellular processes, such as cell apoptosis, DNA damage and mitochondria damage (Kang et al., 2019; Sreedhar et al., 2020; Srinivas et al., 2019). The increasing ROS levels can activate anti-tumourigenic signaling, causing death to the oxidative stress induced-cancer cells (Moloney and Cotter, 2018). Quercetin induces apoptosis by producing intracellular ROS (Kim et al., 2013; Kim et al., 2017). The relationship between quercetin and oxidative stress has been reported (Bishayee et al., 2015), but its specific mechanisms and pathways of anti-cancer effect are still not clear. This study was conducted to understand how quercetin-related changes induce cell apoptosis through ROS-associated signaling pathways.
HepG2 cells were bought from American Type Culture Collection (Manassas, Virginia). Dulbecco’s modified eagle’s medium (DMEM) and fetal bovine serum (FBS) were obtained from Gibco. Cell counting kit-8 (CCK-8), mitochondrial membrane potential assay kit with JC-1, caspase-3, cell cycle and apoptosis analysis kit, and ROS assay kit were purchased from Beyotime Biotechnology. Phosphorylated ataxia-telangiectasia mutated (P-ATM), signal transducer and activator of transcription 3 (STAT-3), c-Jun N-terminal kinases (JNK), Bax, and β-actin were obtained from Cell Signaling Technology. Quercetin was provided by Professor Dong Hao from Zhongkai University of Agriculture and Engineering.
Cell culture and viability assay
The cytotoxicity of quercetin was measured by CCK-8 assay (Gong et al., 2018). First, HepG2 cells were incubated in DMEM supplemented with 10% FBS in 96-well plates at a density of 4 × 104 cells/well for 24 h in a 5% CO2 atmosphere at 37°C. Then, quercetin was added at different concentrations to HepG2 cells incubated in DMEM with 1% FBS for 24 h. Then, 10 μL of CCK-8 solution was added per well and incubated for different durations to detect the absorbance. The absorbance, which indicated the cell viability, was measured at 450 nm via a microplate spectrophotometer. The assay was repeated thrice.
Determination of cell cycle and apoptosis
HepG2 cells with a density of 4 × 104 were planted in 6-well plates. After 24 h, the original medium was discarded, and quercetin with different concentrations (10/20/40 μmol/L) was added for 24 h. The pretreated HepG2 cells were trypsinized and resuspended with PBS, then immobilized with 70% precooled ethanol. After incubating with propidium iodide (PI) at 37°C, HepG2 cells were then analyzed by flow cytometry (Xiong et al., 2019).
Mitochondrial membrane potential staining assay
HepG2 cells treated with different concentrations (10/20/40 μmol/L) of quercetin were washed with PBS. The prepared JC-1 staining solution was added to the above cells and incubated at 37°C, then washed with JC-1 buffer solution thrice. Finally, stained HepG2 cells were observed under a fluorescence microscope (Zeng et al., 2018).
HepG2 was grown overnight to a density of 8 × 104 in a 10 cm petri dish. After 24 h of treatment with quercetin at the above different concentrations, cells were lysed by radioimmunoprecipitation assay (RIPA) buffer and the supernatant was obtained by centrifugation at 4°C. The cellular lysate liquid and testing buffer solution were kept on standby before the testing reagent was added to various samples. The absorbance was measured at 405 nm wavelength using a microplate spectrophotometer (Thermo Fisher Scientific, America) to determine the protein concentration of samples when analyzing the caspase-3 activity (Gu et al., 2018).
Detection of reactive oxygen species generation
HepG2 cells were treated with quercetin at different concentrations for 24 h, then stained with (5(6)-Carboxy-2′,7′-dichlorofluorescein), amine-reactive green fluorophore (DCFA) probe and observed under an inverted fluorescence microscope (Leica, Germany). The level of reactive oxygen species (ROS) was determined by the fluorescence intensity. Excitation wavelength (488 nm) and emission wavelength (525 nm) were used to detect the fluorescence intensity (Deng et al., 2018).
HepG2 was grown at a density of 8 × 104 in a 10 cm petri dish overnight. After 24 h of treatment with quercetin at the above different concentrations, cells were lysed by RIPA, and the supernatant was obtained by centrifugation at 4°C. Each protein was quantified by a BCA assay. X-ray film was used to monitor the target protein (Mishra et al., 2017; Xu et al., 2022).
All the data are shown as mean ± SD. Differences between two groups were analyzed by one-way ANOVA. A probability of p < 0.05 (*) or p < 0.01 (**) indicated statistical significance.
In vitro anticancer activity of quercetin
The viability of quercetin-treated cells was measured by CCK-8 assay. As shown in Fig. 1A, HepG2 cells treated with 10 μM quercetin for 24 h exhibited diminished cell viability to 84%; at 20 and 40 μM concentration the cell viability was dramatically reduced to 56% and 47%, respectively. As shown in Fig. 1B, the effect of quercetin on HepG2 was manifested as a reduction in the number of viable cells, cytoplasm pyknosis, and loss of cell connections.
Figure 1: Effects of quercetin on the proliferation of HepG2 cells by CCK-8 assay. (A) The cell viability was monitored by quercetin at the different concentrations for 24 h. (B) Morphological changes in HepG2 cells were observed by phase-contrast microscopy. Bars with different characters are statistically different at *p < 0.05 or **p < 0.01 (n = 3).
Induction of cell apoptosis by quercetin
Apoptosis makes a momentous difference in many aspects, such as organ development, anti-tumor, and treatment of cardiovascular diseases (Codispoti et al., 2019; Dong et al., 2019; Pistritto et al., 2016). The effect of quercetin on cell apoptosis was detected by flow cytometry. The flow cytometer can detect DNA fragmentation that takes the shape of a sub-G1 peak. The blue low peak ahead of the G0/G1 peak indicates the apoptosis proportion. According to Fig. 2, the control group showed 1.03% apoptosis rate. Compared with HepG2 cells treated with 10 μM (4.49%) and 20 μM (5.84%), the rate of apoptosis was higher (16.41%) when exposed to 40 μM quercetin.
Figure 2: Flow cytometric analysis of HepG2 cells after treatment with quercetin for 24 h incubated with PI after immobilization with 70% precooled ethanol. The cell cycle distribution and apoptotic cell population were determined by a flow cytometer.
Depletion of mitochondrial membrane potential (ΔΨm)
As shown in Fig. 3, a bright field revealed that quercetin decreased the number of alive cells and caused more death as its concentration increased. When the mitochondrial membrane potential was high, JC-1 monomers assembled to form J-aggregates emitting red fluorescence. In contrast, green fluorescence was generated when the potential was low, which is a symbolic event in the early stage of apoptosis. As shown in Fig. 3A, with the increase in concentration, the mitochondrial membrane potential in the cell gradually decreases. Fig. 3B shows the result of the quantization of green fluorescence intensity using IMAGE J. The green fluorescence increased gradually with concentration, suggesting that Quercetin could down-regulate mitochondrial membrane potential.
Figure 3: The mitochondrial membrane potential of quercetin-treated cells for 24 h was detected with JC-1 fluorescent probe under a fluorescent microscope. (A) Fluorescence microscope photograph of JC-1 staining. (B) IMAGE J software was used to quantify the green fluorescence of the JC-1 monomer (n = 3).
Induction of caspase cleavage by quercetin
Caspase-3 plays a pivotal role in the process of cell apoptosis (Jiang et al., 2020). As shown in Fig. 4, the caspase-3 activity of the control group was 100%, while HepG2 treated with 10, 20, and 40 μM quercetin, exhibited 115%, 143%, and 231% caspase-3 activity. These data indicated that quercetin markedly activated caspase-3 activity and promoted the rate of apoptosis.
Figure 4: The activation of caspase-3 was triggered by different concentrations of quercetin, as examined via the synthetic fluorogenic substrate. Bars with different characters are statistically different at *p < 0.05, **p < 0.01 or ***p < 0.001 (n = 3).
Induction of reactive oxygen species generation by quercetin
ROS plays an important role in cell apoptosis. The ROS generation was detected by DCFA fluorescence assay. As shown in Fig. 5A, a remarkably increased accumulation of the intracellular ROS was observed as the concentration of quercetin increased (control 100%, 10 μM 124%, 20 μM 137%, 40 μM 192%), consistent with a previous study (Kim et al., 2014). The fluorescence intensity of the quercetin-treated HepG2 cells is shown in Fig. 5B.
Figure 5: The overproduction of ROS induced by quercetin. (A) ROS levels were detected by 10 μM DCF fluorescence using a microplate spectrophotometer. (B) The intracellular fluorescence intensity was observed by a fluorescent microscope. Bars with different characters are statistically different at *p < 0.05 or **p < 0.01 (n = 3).
Activation of reactive-oxygen species-mediated apoptotic signaling pathways by quercetin
Excess generation of ROS can cause DNA damage, induce the expression of pro-apoptotic proteins, and restrict the anti-apoptotic protein. The effects of quercetin on the expression of these proteins were detected by western blotting. As shown in Fig. 6A, the expression of P-ATM, JNK, and Bax were distinctly activated, and STAT-3 was significantly suppressed with a gradually increased concentration of quercetin. Collectively, these data demonstrated that quercetin induced HepG2 cell apoptosis through ROS-mediated P-ATM, JNK, Bax, and STAT-3 signaling pathways, as shown in Fig. 6B.
Figure 6: The apoptotic signaling pathways were activated by quercetin. (A) The HepG2 cells exposed to different concentrations of quercetin expressed the pro-apoptotic and anti-apoptotic protein tracked by western blotting. β-actin was used as the loading control. (B) The apoptotic signaling pathways induced by quercetin include ROS-mediated P-ATM, JNK, Bax and STAT-3 signaling pathways.
Quercetin is the major representative of the important bioactive flavonoids and possesses anti-inflammatory (Hou et al., 2019), anti-oxidation (Song et al., 2020b), anti-diabetic (Bule et al., 2019), and anti-allergic properties. Quercetin exerts anti-inflammatory effects by reducing the expression of inflammatory factors such as interferon-γ and tumor necrosis factor-α on the skin of mice with specific dermatitis. Quercetin acts as an antioxidant by interacting with DNA. In terms of the anti-diabetic role, quercetin reduces serum glucose mainly through antioxidant action and regulation of liver gene expression. In terms of anti-allergy, quercetin alleviates the inflammatory response of allergic conjunctivitis by inhibiting the membrane-associated protein tyrosine kinase (Lyn) (Ding et al., 2020). In previous studies, the anti-tumor activity of quercetin was reported (Azeem et al., 2022), which inhibits tumor cell proliferation and downregulates tumor protein expression (Ozkan and Bakar-Ates, 2020; Song et al., 2020a). In vitro experiments showed that quercetin alone can induce apoptosis of 13 hepatocellular carcinoma cell lines. On the other hand, quercetin conjugator quercetin-3-O-glucoside (Q3G) shows a strong ability to inhibit DNA topoisomerase II and induces apoptosis of HepG2 cells by activating caspase-3. Q3G is also a potential anticancer agent for liver cancer, and its mechanism of action may be cell cycle arrest and apoptosis induction (Sudan and Rupasinghe, 2014).
Here, we have examined the effect of quercetin in inducing apoptosis of tumor cells. Assessment of the in vitro anticancer activity of quercetin revealed that as the concentration of quercetin increased, the cell viability decreased, and the number of cells with normal morphology decreased. This suggests that quercetin distinctly suppressed HepG2 cell proliferation. Cell cycle and apoptosis can be observed directly by flow cytometry. The increase in apoptotic peaks indicated that quercetin inhibited cell proliferation through apoptosis in a concentration-dependent manner. The early apoptosis can be reflected by the depletion of mitochondrial membrane potential and is revealed by green fluorescence in the JC-1 experiment. The results further demonstrate that quercetin contributed to HepG2 apoptosis and had an impact on mitochondria.
ROS is related to tumors, nervous diseases, cardiovascular diseases, etc. (Climent et al., 2020; Kang et al., 2019; Tonnies and Trushina, 2017; Liu et al., 2021). It is involved in the process of apoptosis, DNA damage, and mitochondrial damage (Moloney and Cotter, 2018; Sreedhar et al., 2020; Srinivas et al., 2019). When HepG2 cells were treated with increasing concentration of quercetin, the production and fluorescence intensity of ROS increased, which collectively indicated that quercetin induced ROS generation. Excessive production of ROS can activate the anti-tumor signaling pathway, leading to oxidative stress-induced apoptosis. Western blotting showed that P-ATM, JNK, Bax, and STAT-3 were involved. The expression of P-ATM, JNK, and Bax was up-regulated, which suggested that they promote apoptosis through ROS generation downstream (Niu et al., 2021; Dandoti, 2021). The expression of STAT-3 was decreased, indicating the inhibition of its cell growth and survival promotion function.
Research into new biomaterial technologies such as drug-supported nanomaterials and nano-antioxidants is increasing. Quercetin-loaded nano-lipid structure showed protective and antioxidant activity against paraquat-induced cytotoxicity, and quercetin nanoparticles showed a protective effect against aflatoxin B1-induced hepatotoxicity (Ahmadian et al., 2020; Eftekhari et al., 2018). Therefore, quercetin can be combined with nanomaterials for the synergistic treatment of liver diseases.
In summary, quercetin dramatically inhibited the dose-dependent proliferation of HepG2 cells. Additionally, the treatment enhanced the proportion of apoptotic cells, shown in the sub-G1 peak. The intracellular quercetin attacked mitochondria and caused repression of mitochondrial membrane potential, which triggered excess ROS production. The expression of the pro-apoptotic protein was activated through ROS downstream P-ATM, JNK, and Bax signaling pathways. Therefore, our study suggests that quercetin with anti-tumor properties may be a promising flavonol compound in the treatment of HCC.
Availability of Data and Material: All data generated or analyzed during this study are included in this published article and its supplementary information files.
Author Contribution: Draft manuscript preparation: WTL; Experiments carry out: DYC and JYS; Analysis of results: RLZ; Data collection RK; Study design: BZ, HD, and YHL. All authors reviewed the final version of the manuscript.
Ethics Approval: This project was approved by the Ethics Committee of Guangzhou Women and Children Medical Center (Approval No. 2017021803).
Funding Statement: This work was supported by the Guangdong Natural Science Foundation (2020A1515110648), the Open Fund of Guangdong Provincial Key Laboratory of Functional Supramolecular Coordination Materials and Applications (2020A03), the Technology Planning Project of Guangzhou (202102010202 and 202201020655), the Open Project of Guangdong Key Laboratory of Marine Materia Medica (LMM2020-7) and the Guangzhou Medical University Students’ Science and Technology Innovation Project (02-408-2203-2079, 2021AEK119, 2021AEK122, 2021AEK125 and 2021AEK128).
Conflicts of Interest: The authors declare that they have no conflicts of interest to report regarding the present study.
References
Azeem M, Hanif M, Mahmood K, Ameer N, Chughtai F, Abid U (2022). An insight into anticancer, antioxidant, antimicrobial, antidiabetic and anti-inflammatory effects of quercetin: A review. Polymer Bulletin 1: 22. DOI 10.1007/s00289-022-04091-8. [Google Scholar] [CrossRef]
Ahmadian E, Eftekhari A, Kavetskyy T, Khosroushahi AY, Turksoy VA, Khalilov R (2020). Effects of quercetin loaded nanostructured lipid carriers on the paraquat-induced toxicity in human lymphocytes. Pesticide Biochemistry and Physiology 167: 104586. DOI 10.1016/j.pestbp.2020.104586. [Google Scholar] [CrossRef]
Badolato M, Carullo G, Perri M, Cione E, Manetti F, Di Gioia ML, Brizzi A, Caroleo MC, Aiello F (2017). Quercetin/oleic acid-based G-protein-coupled receptor 40 ligands as new insulin secretion modulators. Future Medicinal Chemistry 9: 1873–1885. DOI 10.4155/fmc-2017-0113. [Google Scholar] [CrossRef]
Bishayee K, Khuda-Bukhsh AR, Huh SO (2015). PLGA-loaded gold-nanoparticles precipitated with quercetin downregulate HDAC-Akt activities controlling proliferation and activate p53-ROS crosstalk to induce apoptosis in hepatocarcinoma cells. Molecules and Cells 38: 518–527. DOI 10.14348/molcells.2015.2339. [Google Scholar] [CrossRef]
Bule M, Abdurahman A, Nikfar S, Abdollahi M, Amini M (2019). Antidiabetic effect of quercetin: A systematic review and meta-analysis of animal studies. Food and Chemical Toxicology 125: 494–502. DOI 10.1016/j.fct.2019.01.037. [Google Scholar] [CrossRef]
Climent M, Viggiani G, Chen YW, Coulis G, Castaldi A (2020). MicroRNA and ROS crosstalk in cardiac and pulmonary diseases. International Journal of Molecular Sciences 21: 1–34. DOI 10.3390/ijms21124370. [Google Scholar] [CrossRef]
Codispoti B, Makeeva I, Sied J, Benincasa C, Scacco S, Tatullo M (2019). Should we reconsider the apoptosis as a strategic player in tissue regeneration? International Journal of Molecular Sciences 15: 2029–2036. DOI 10.7150/ijbs.36362. [Google Scholar] [CrossRef]
Dandoti S (2021). Mechanisms adopted by cancer cells to escape apoptosis–A review. BIOCELL 45: 863–884. DOI 10.32604/biocell.2021.013993. [Google Scholar] [CrossRef]
Deng X, Huang W, Peng J, Zhu TT, Sun XL et al. (2018). Irisin alleviates advanced glycation end products-induced inflammation and endothelial dysfunction via inhibiting ROS-NLRP3 inflammasome signaling. Inflammation 41: 260–275. DOI 10.1007/s10753-017-0685-3. [Google Scholar] [CrossRef]
Ding Y, Li C, Zhang Y, Ma P, Zhao T et al. (2020). Quercetin as a Lyn kinase inhibitor inhibits IgE-mediated allergic conjunctivitis. Food and Chemical Toxicology 135: 110924. DOI 10.1016/j.fct.2019.110924. [Google Scholar] [CrossRef]
Dong Y, Chen H, Gao J, Liu Y, Li J, Wang J (2019). Molecular machinery and interplay of apoptosis and autophagy in coronary heart disease. Journal of Molecular and Cellular Cardiology 136: 27–41. DOI 10.1016/j.yjmcc.2019.09.001. [Google Scholar] [CrossRef]
Eftekhari A, Ahmadian E, Panahi-Azar V, Hosseini H, Tabibiazar M, Maleki DS (2018). Hepatoprotective and free radical scavenging actions of quercetin nanoparticles on aflatoxin B1-induced liver damage: In vitro/in vivo studies. Artificial Cells Nanomedicine and Biotechnology 46: 411–420. DOI 10.1080/21691401.2017.1315427. [Google Scholar] [CrossRef]
Forner A, Reig M, Bruix J (2018). Hepatocellular carcinoma. Lancet 391: 1301–1314. DOI 10.1016/S0140-6736(18)30010-2. [Google Scholar] [CrossRef]
Gong J, Jin J, Zhao L, Li Y, Li Y, He Q (2018). Tripterygium glycoside protects against puromycin amino nucleosideinduced podocyte injury by upregulating autophagy. International Journal of Molecular Medicine 42: 115–122. DOI 10.3892/ijmm.2018.3598. [Google Scholar] [CrossRef]
Gu C, Chen C, Wu R, Dong T, Hu X, Yao Y, Zhang Y (2018). Long noncoding RNA EBF3-AS promotes neuron apoptosis in alzheimer’s disease. Dna and Cell Biology 37: 220–226. DOI 10.1089/dna.2017.4012. [Google Scholar] [CrossRef]
Han J, Fan YC, Wang K (2020). Radiofrequency ablation versus microwave ablation for early stage hepatocellular carcinoma: A PRISMA-compliant systematic review and meta-analysis. Medicine 99: e22703. DOI 10.1097/MD.0000000000022703. [Google Scholar] [CrossRef]
Hou D, Zhang W, Gao Y, Sun Y, Wang H, Qi R, Chen H, Gao X (2019). Anti-inflammatory effects of quercetin in a mouse model of MC903-induced atopic dermatitis. International Immunopharmacology 74: 105676. DOI 10.1016/j.intimp.2019.105676. [Google Scholar] [CrossRef]
Jiang M, Qi L, Li L, Li Y (2020). The caspase-3/GSDME signal pathway as a switch between apoptosis and pyroptosis in cancer. Cell Death Discovery 6: 112. DOI 10.1038/s41420-020-00349-0. [Google Scholar] [CrossRef]
Kakehashi A, Suzuki S, Ishii N, Okuno T, Kuwae Y, Fujioka M, Gi M, Stefanov V, Wanibuchi H (2020). Accumulation of 8-hydroxydeoxyguanosine, l-arginine and glucose metabolites by liver tumor cells are the important characteristic features of metabolic syndrome and non-alcoholic steatohepatitis-associated hepatocarcinogenesis. International Journal of Molecular Sciences 21: 1–23. DOI 10.3390/ijms21207746. [Google Scholar] [CrossRef]
Kang R, Li R, Dai P, Li Z, Li Y, Li C (2019). Deoxynivalenol induced apoptosis and inflammation of IPEC-J2 cells by promoting ROS production. Environmental Pollution 251: 689–698. DOI 10.1016/j.envpol.2019.05.026. [Google Scholar] [CrossRef]
Kim GT, Lee SH, Kim JI, Kim YM (2014). Quercetin regulates the sestrin 2-AMPK-p38 MAPK signaling pathway and induces apoptosis by increasing the generation of intracellular ROS in a p53-independent manner. International Journal of Molecular Medicine 33: 863–869. DOI 10.3892/ijmm.2014.1658. [Google Scholar] [CrossRef]
Kim GT, Lee SH, Kim YM (2013). Quercetin regulates sestrin 2-AMPK-mTOR signaling pathway and induces apoptosis via increased intracellular ROS in HCT116 colon cancer cells. Journal of Cancer Prevention 18: 264–270. DOI 10.15430/JCP.2013.18.3.264. [Google Scholar] [CrossRef]
Kim SY, Jeong HC, Hong SK, Lee MO, Cho SJ, Cha HJ (2017). Quercetin induced ROS production triggers mitochondrial cell death of human embryonic stem cells. Oncotarget 8: 64964–64973. DOI 10.18632/oncotarget.11070. [Google Scholar] [CrossRef]
Lin R, Piao M, Song Y, Liu C (2020). Quercetin suppresses AOM/DSS-induced colon carcinogenesis through its anti-inflammation effects in mice. Journal of Immunology Research 2020: 9242601. DOI 10.1155/2020/9242601. [Google Scholar] [CrossRef]
Lin ZY, Li GL, Chen J, Chen ZW, Chen YP, Lin SZ (2016). Effect of heat sink on the recurrence of small malignant hepatic tumors after radiofrequency ablation. Journal of Cancer Research and Therapeutics 12: C153–C158. DOI 10.4103/jcrt.JCRT_959_16. [Google Scholar] [CrossRef]
Liu C, Kong Q, Pan F, Jiang S, Meng L, Huang G, Lu L, Li S, Liu Y (2021). Hellebrigenin induces apoptosis in colorectal cancer Cells through induction of excessive reactive oxygen species. BIOCELL 45: 943–951. DOI 10.32604/biocell.2021.015836. [Google Scholar] [CrossRef]
Niu H, Chen X, Fu X, Zhang J, Li G, et al. (2021). SOD1G93A induces a unique PSAP-dependent mitochondrial apoptosis pathway via bax-bak interaction. BIOCELL 45: 963–970. DOI 10.32604/biocell.2021.015297. [Google Scholar] [CrossRef]
Mishra M, Tiwari S, Gomes AV (2017). Protein purification and analysis: Next generation Western blotting techniques. Expert Review of Proteomics 14: 1037–1053. DOI 10.1080/14789450.2017.1388167. [Google Scholar] [CrossRef]
Moloney JN, Cotter TG (2018). ROS signalling in the biology of cancer. Seminars in Cell & Developmental Biology 80: 50–64. DOI 10.1016/j.semcdb.2017.05.023. [Google Scholar] [CrossRef]
Ozkan E, Bakar-Ates F (2020). Potentiation of the effect of Lonidamine by Quercetin in MCF-7 human breast cancer cells through downregulation of MMP-2/9 mRNA expression. Anais da Academia Brasileira de Ciencias 92: e20200548. DOI 10.1590/0001-3765202020200548. [Google Scholar] [CrossRef]
Pal A, Tripathi A (2020). Toxicological and behavioral study of two potential antibacterial agents: 4-chloromercuribenzoic acid and quercetin on Swiss-albino mice. Drug and Chemical Toxicology 43: 645–655. DOI 10.1080/01480545.2018.1517774. [Google Scholar] [CrossRef]
Pistritto G, Trisciuoglio D, Ceci C, Garufi A, D’Orazi G (2016). Apoptosis as anticancer mechanism: Function and dysfunction of its modulators and targeted therapeutic strategies. Sedentary Life and Nutrition 8: 603–619. DOI 10.18632/aging.100934. [Google Scholar] [CrossRef]
Rauf A, Imran M, Khan IA, Ur-Rehman M, Gilani SA, Mehmood Z, Mubarak MS (2018). Anticancer potential of quercetin: A comprehensive review. Phytotherapy Research 32: 2109–2130. DOI 10.1002/ptr.6155. [Google Scholar] [CrossRef]
Reyes-Farias M, Carrasco-Pozo C (2019). The anti-cancer effect of quercetin: Molecular implications in cancer metabolism. International Journal of Molecular Sciences 20: 3177. DOI 10.3390/ijms20133177. [Google Scholar] [CrossRef]
Shabbir U, Rubab M, Daliri EB, Chelliah R, Javed A, Oh DH (2021). Curcumin, quercetin, catechins and metabolic diseases: The role of gut microbiota. Nutrients 13: 1–23. DOI 10.3390/nu13010206. [Google Scholar] [CrossRef]
Sheng J, Qin H, Zhang K, Li B, Zhang X (2018). Targeting autophagy in chemotherapy-resistant of hepatocellular carcinoma. American Journal of Cancer Research 8: 354–365. ISSN:2156-6976/ajcr0073478. [Google Scholar]
Siegel RL, Miller KD, Jemal A (2020). Cancer statistics, 2020. CA-A Cancer Journal for Clinicians 70: 7–30. DOI 10.3322/caac.21590. [Google Scholar] [CrossRef]
Sies H, Jones DP (2020). Reactive oxygen species (ROS) as pleiotropic physiological signalling agents. Nature Reviews Molecular Cell Biology 21: 363–383. DOI 10.1038/s41580-020-0230-3. [Google Scholar] [CrossRef]
Song J, Bai J, Wang S, Liu L, Zhao Z (2020a). Effects of quercetin on autophagy and phosphatidylinositol 3-kinase/Protein kinase B/Mammalian target of rapamycin signaling pathway in human prostate cancer PC-3 cells. Zhongguo Yi Xue Ke Xue Yuan Xue Bao. Acta Academiae Medicinae Sinicae 42: 578–584. DOI 10.3881/j.issn.1000-503X.12361. [Google Scholar] [CrossRef]
Song X, Wang Y, Gao L (2020b). Mechanism of antioxidant properties of quercetin and quercetin-DNA complex. Journal of Molecular Modeling 26: 133. DOI 10.1007/s00894-020-04356-x. [Google Scholar] [CrossRef]
Sreedhar A, Aguilera-Aguirre L, Singh KK (2020). Mitochondria in skin health, aging, and disease. Cell Death & Disease 11: 444. DOI 10.1038/s41419-020-2649-z. [Google Scholar] [CrossRef]
Srinivas US, Tan B, Vellayappan BA, Jeyasekharan AD (2019). ROS and the DNA damage response in cancer. Redox Biology 25: 101084. DOI 10.1016/j.redox.2018.101084. [Google Scholar] [CrossRef]
Sudan S, Rupasinghe HP (2014). Quercetin-3-O-glucoside induces human DNA topoisomerase II inhibition, cell cycle arrest and apoptosis in hepatocellular carcinoma cells. Anticancer Research 34: 1691–1699. [Google Scholar]
Tang SM, Deng XT, Zhou J, Li QP, Ge XX, Miao L (2020). Pharmacological basis and new insights of quercetin action in respect to its anti-cancer effects. Biomedicine & Pharmacotherapy 121: 109604. DOI 10.1016/j.biopha.2019.109604. [Google Scholar] [CrossRef]
Tonnies E, Trushina E (2017). Oxidative stress, synaptic dysfunction, and alzheimer’s disease. Journal of Alzheimers Disease 57: 1105–1121. DOI 10.3233/JAD-161088. [Google Scholar] [CrossRef]
Ward AB, Mir H, Kapur N, Gales DN, Carriere PP, Singh S (2018). Quercetin inhibits prostate cancer by attenuating cell survival and inhibiting anti-apoptotic pathways. World Journal of Surgical Oncology 16: 108. DOI 10.1186/s12957-018-1400-z. [Google Scholar] [CrossRef]
Xiong Y, Li T, Assani G, Ling H, Zhou Q, Zeng Y, Zhou F, Zhou Y (2019). Ribociclib, a selective cyclin D kinase 4/6 inhibitor, inhibits proliferation and induces apoptosis of human cervical cancer in vitro and in vivo. Biomedicine & Pharmacotherapy 112: 108602. DOI 10.1016/j.biopha.2019.108602. [Google Scholar] [CrossRef]
Xu T, Zheng R, Chen D, Chen H, Zhao M et al. (2022). Inhibitory of EV-A71 virus-induced apoptosis by ZVAD through ROS mediated signaling pathways. BIOCELL 46: 1033–1039. DOI 10.32604/biocell.2022.017757. [Google Scholar] [CrossRef]
Yang JD, Heimbach JK (2020). New advances in the diagnosis and management of hepatocellular carcinoma. BMJ 371: m3544. DOI 10.1136/bmj.m3544. [Google Scholar] [CrossRef]
Zeng X, Chen S, Lin Y, Ke Z (2018). Acylated and unacylated ghrelin inhibit apoptosis in myoblasts cocultured with colon carcinoma cells. Oncology Reports 39: 1387–1395. DOI 10.3892/or.2018.6213. [Google Scholar] [CrossRef]
Zhu B, Li Y, Lin Z, Zhao M, Xu T, Wang C, Deng N (2016). Silver nanoparticles induce HePG-2 cells apoptosis through ROS-mediated signaling pathways. Nanoscale Research Letters 11: 198. DOI 10.1186/s11671-016-1419-4. [Google Scholar] [CrossRef]
Cite This Article
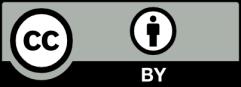