Open Access
ARTICLE
Advanced glycation end-products change placental barrier function and tight junction in rats with gestational diabetes mellitus via the receptor for advanced glycation end products/nuclear factor-κB pathway
1 Department of Obstetrics and Gynaecology, Zhongda Hospital, School of Medicine, Southeast University, Nanjing, 210023, China
2 School of Integrated Chinese and Western Medicine, Nanjing University of Chinese Medicine, Nanjing, 210023, China
3 School of Pharmacy, Nanjing University of Chinese Medicine, Nanjing, 210023, China
* Corresponding Author: Hong Yu,
# Authors contributed equally to this work
(This article belongs to the Special Issue: Reproductive Health and Embryonic Development)
BIOCELL 2023, 47(1), 165-173. https://doi.org/10.32604/biocell.2022.023043
Received 07 April 2022; Accepted 16 June 2022; Issue published 26 September 2022
Abstract
The placenta plays an important role in nutrient transport to maintain the growth and development of the embryo. Gestational diabetes mellitus (GDM), the most common complication during pregnancy, highly affects placental function in late gestation. Advanced glycation end-products (AGEs), a complex and heterogeneous group of compounds engaged by the receptor for AGEs (RAGE), are closely associated with diabetes-related complications. In this study, AGEs induced a decrease in the expression of tight junction (TJ) proteins in BeWo cells and increased the paracellular permeability of trophoblast cells by regulating RAGE/NF-κB. Sprague-Dawley (SD) rats injected with 100 mg/kg AGEs-rat serum albumin (RSA) via the tail vein from embryo day 2 were set as the placental barrier dysfunction model group (n = 10). The effect of AGEs on placental permeability was determined using the EvansBlue dye extravasation method. The ultrastructure of the placenta samples was observed by transmission electron microscopy. The effects of AGEs on the placenta were confirmed by treating rats with RAGE antagonist FPS-ZM1 and soluble forms of RAGE (sRAGE). AGEs treatment increased placental permeability and disrupted the tight junctions in pregnant rat placenta, but has no effect on blood glucose. The expression of TJ-related proteins, including ZO-1, Occludin, and Claudin 5, were downregulated after AGEs treatment. Further, AGEs treatment increased the expression of RAGE and nuclear factor-κB in the placenta of rats and upregulated the levels of vascular endothelial growth factor. The effects of AGEs on the placenta were blocked by RAGE antagonist FPS-ZM1 and sRAGE. This study demonstrates the mechanism underlying AGEs-induced disturbance in placental function in pregnant rats and highlights the potential of AGEs in the treatment of GDM.Keywords
Structural and functional abnormalities of the placental barrier are often present in patients with gestational diabetes and are associated with adverse pregnancy outcomes (Aires and Dos Santos, 2015; Jarmuzek et al., 2015). The placental barrier mainly comprises the vasculo-syncytial membranes (VSM), and the solute molecules pass the VSM mainly via transcytosis and the paracellular pathway. Researches have confirmed the existence of the paracellular pathway in the human placenta (Sibley et al., 2018), and the path is closely associated with the transport of a variety of solutes. The work of the paracellular pathway is determined by the tight junctions (TJs). The presence of tight junction structures has been reported in both trophoblast cells of the placenta and fetal vascular epithelial cells (Leach et al., 2000). The main functions of TJs include acting as a barrier in the vasculature, controlling the permeability of the endothelial cells by regulating the diffusion of fluid, ions, and small plasma proteins, and the infiltration of cells such as leukocytes, neutrophils, and lymphocytes (Shen et al., 2011), maintaining cell polarity by forming a “fence” to prevent the diffusion of apical and basolateral membrane proteins and lipids (van Meer and Simons, 1986), and participating in intercellular and endosomal signal transduction by interacting with some intracellular cytokines to regulate cell differentiation, proliferation, and apoptosis (Citi et al., 2014). TJs, therefore, are essential for maintaining the placental barrier functions. TJs include Claudin and Occludin proteins and consist of transmembrane proteins, cytoplasmic scaffolding, bridging proteins, and related signal proteins; the main components of TJ are transmembrane proteins. Claudin proteins have several isoforms. Claudin-5 is the most abundant endothelial-specific closure protein in endothelial cells (Ohtsuki et al., 2007). The main role of Claudin proteins is to form the core-TJ chain of the TJ structure (Furuse et al., 1998). The role of Occludin in the TJ is to maintain the stability and barrier function of TJ (Saitou et al., 2000). The cytoplasmic scaffolding proteins of the TJ are composed of ZO proteins (ZO-1, ZO-2, and ZO-3), which not only bind to TJ transmembrane proteins but also connect to the cytoskeleton, and are involved in the regulation of cellular signaling (Citi et al., 2014).
Research from Hayward et al. (van der Aa et al., 1998) showed that placental transport of glucose and neutral amino acids were altered in the gestational diabetes mellitus (GDM) state. However, the changes that the placenta makes to macromolecules such as albumin in the GDM state is yet unclear. In this study, the Evans-Blue (EB) assay was used to assess the permeability of the placenta, where EB enters the body and binds to albumin. The detection of EB in the placenta could provide evidence for the transport of albumin through the placenta (Radu and Chernoff, 2013). Most previous studies concluded that placental dysfunction in GDM patients is caused by hyperglycemia (Leach et al., 2009; Sobrevia et al., 2016), whereas some studies have found that dietary control or insulin treatment could decrease the blood glucose of pregnant women and newborns to the normal level. However, the above-mentioned therapies could not improve fetal or neonatal situations (Subiabre et al., 2018), meanwhile, pregnant women and newborns still suffer the dysfunction in placental microvascular and macrovascular endothelial cells (Sobrevia et al., 2015), which even leads to alterations in placental structure and function (Baumüller et al., 2015). Evidence indicated that factors other than hyperglycemia may be involved in the pathophysiologic development of GDM.
In many studies, advanced glycation end-products (AGEs) were confirmed at high levels in women with GDM (Bartakova et al., 2016; Harsem et al., 2008; Shi et al., 2020a), while AGEs remained at high levels even after normal glycemic control and were associated with poor fetal prognosis (Guosheng et al., 2009). AGEs exert deleterious effects by directly damaging cells or binding to specific receptors of AGEs (RAGE). The binding of AGEs with RAGE leads to activation of the nuclear factor (NF)-κB pathway (Xie et al., 2013; Shi et al., 2020b) and enhances the release of vascular endothelial growth factor (VEGF) (Boulanger et al., 2007), which is a very important cell-permeable molecule. Thus, AGEs are one of the factors that are worth investigating in the etiology of placental barrier dysfunction in GDM patients. Accumulation of AGEs may lead to placental oxidation and placental vascular bed disorders, which in turn deteriorate the development of preeclampsia. Studies suggested that AGEs are associated with a variety of barrier structures and dysfunctions in vivo, but the relationship between AGEs on the placental barriers is still unclear. In this study, we confirmed the altered placental barrier function in the gestational state by assessing the placental permeability (EB staining assay), placental ultrastructure, and changes in the expression of TJ factors (ZO-1, Occludin, Claudin5) in the placenta of gestational rats. The changes in placental permeability, placental ultrastructure, and placental TJ factor (ZO-1, Occludin, Claudin5) in GDM rats in late pregnancy were observed from the intervention of rats with RAGE antagonist FPS-ZM1 and soluble forms of RAGE (sRAGE), respectively. The (sRAGE) bind to the ligand of RAGE but do not have the intracellular effects of RAGE, thus playing a competitive role in the inhibition of RAGE. To investigate the causes of abnormal placental barrier function in the gestational state, we also examined the changes in placental RAGE and NF-κB expression.
The antibodies used in this study were: rabbit anti-zonular occludens-1 (ZO-1) (61-7300, Invitrogen, Thermo Fisher Scientific, Inc.), rabbit anti-Occludin (ab216327, Abcam), rabbit anti-NF-κB p65 (ab16502, Abcam), rabbit anti-GAPDH (ab9485, Abcam) and goat anti-rabbit APC secondary antibody (ab130805, Abcam).
Preparation of AGEs-modified rat serum albumin (AGEs-RSA) solution
Phosphate buffer saline (PBS, 0.2 mol/L, pH 7.4) containing RSA (50 g/L), glucose (500 mmol/L), penicillin (100 U/mL), and gentamicin (70 μg/mL) was stirred thoroughly and adjusted at the pH of 7.4, then kept at room temperature for 24 h. The solution was incubated for one week at 50°C under sterile conditions, and unincorporated glucose was removed. A glucose-free RSA incubation solution was prepared under the same conditions and used as a control. The protein content was determined through the Coomassie brilliant blue method, and the AGEs content was determined using a fluorescence spectrophotometer (excitation at 370 nm and emission at 440 nm). The concentrations of AGEs in the prepared AGEs-RSA solution and RSA control were 28 mg/mL and 0.8 mg/mL, respectively. The endotoxin content of all samples was below 0.5 kEU/L.
Sprague-Dawley (SD) rats (8–10-week-old) were acquired from Beijing Vital River Laboratory Animal Technology Co., Ltd., China. Animals were kept under standard laboratory conditions (temperature 21 ± 1°C, relative humidity 55% ± 10%, 12 h:12 h alternating light and dark). Animals were fed with standard laboratory diet with free access to water and food. The study protocol was approved by the Ethics Committee of Southeast University.
10–12-week-old SD female rats were randomly categorized into four groups (n = 10 in each group). Two female rats in individual cages were mated with one male rat. (1) Female rats were injected with RSA (100 mg/kg) through the tail vein every other day from day 2 of pregnancy (E2) until execution at E16 (RSA group). (2) Female rats were injected with AGEs-RSA (100 mg/kg) through the tail vein every other day from E2 until execution at E16 (AGEs-RSA group). (3) Female rats were injected intraperitoneally with FPS-ZM1 (1 mg/kg/d) from 1 week before mating with male rats until the day of delivery and injected with AGEs-RSA 100 mg/kg through the tail vein every other day from E2 until execution at E16 (FPS-ZM1 group) (4) Female rats were injected with sRAGE 5 mg/kg through the tail vein from E1 until the day of delivery, injected with AGEs-RSA 100 mg/kg through the tail vein every other day from E2 until execution at E16 (AGEs + sRAGE group). The glucose levels were measured from abdominal aortic blood samples at E16.
At E16, 0.2% sterile solution of EB in PBS was prepared and filter-sterilized the solution to remove insoluble particulate matter. Female rat was placed in the restraint device and held by the tail. EB solution (2.5 μL/g) was slowly injected into the tail vein of the rat. The rat was placed back into the cage and observed for 45 min. Then, the rat was sacrificed through cervical dislocation, and the abdominal cavity was opened to collect the placental tissues. Placental tissues were dried at 55°C, and formamide was added to extract EB from the tissue. The absorbance value (OD) was measured at 610 nm using a microplate reader (Model 680, Bio-Rad, America), and formamide was used as a blank control. The amount of EB exuded per mg of tissue was calculated according to the standard curve equation.
Transmission electron microscopy
Placenta samples were placed in a buffer containing 0.05 M sodium dimethyl arsenate and fixed in 2.5% glutaraldehyde buffer at 4°C for 1 h, then stored at 4°C for 24 h. After dehydration with a graded ethanol system, placenta samples were immobilized in 1% osmium tetroxide at 4°C for 1 h, then placed in a graded acetone system. The samples were embedded in paraffin and cut into slices of 1 μm thickness. The slices were stained with 2% uranyl acetate for 10 min and followed by 100 µL lead citrate for 5 min at 25°C. The ultrastructure of the placenta samples was observed under the transmission electron microscope.
Placental tissues were lysed in RIPA buffer on ice. The total protein concentration was determined by using the BCA protein assay kit. Proteins were separated on sodium dodecyl sulfate-polyacrylamide gel electrophoresis gels and transferred to a polyvinylidene difluoride membrane. After that, the membranes were blocked in skimmed milk for 1 h at room temperature and incubated overnight with primary antibodies at 4°C, followed by incubation with HRP-conjugated secondary antibodies for 2 h at room temperature. The results were obtained using Chemiluminescent detectors.
Real-time quantitative polymerase chain reaction
Placental tissue RNA was extracted by Trizol® (Invitrogen, Thermo Fisher Scientific, Inc.). Total RNA concentrations were estimated to conduct a reverse transcription reaction. Primer sequences for analysis of ZO-1, Claudin5, VEGF, RAGE, NF-κB, and β-actin are listed in Table 1. The relative level of expression of genes was estimated using the 2−ΔΔCT method.
SPSS 25.0 was used for statistical processing. The indicators were expressed as mean ± SD, and analysis of variance (ANOVA) was used to analyze related statistical data; the S-N-K method and Dunnett method were used for two-by-two comparisons between the three groups, and P < 0.05 was considered a statistically significant difference.
Effect of AGEs on rat bodyweight and blood glucose
The body weight of rats was measured on the day of delivery (Fig. 1A) and showed that AGEs significantly decreased the body weight of rats. Estimation of blood glucose (Fig. 1B) demonstrated that AGEs elevated the blood glucose of rats, but no significant difference was observed in the RSA group. Further, the effects of AGEs on body weight and blood glucose of rats were blocked by administrating rats with the RAGE antagonist (FPS-ZM1) and sRAGE (Fig. 1).
Figure 1: The effect of AGEs on rat bodyweight and blood glucose (A) AGEs reduced the body weight of female rats, while this effect was abolished by using RAGE antagonist (FPS-ZM1) and soluble receptors of AGE (sRAGE). (B) AGEs have no effect on the blood glucose level of female rats, and those administrated with FPS-ZM1 and sRAGE did not have altered blood glucose levels. n = 10. The data represent the mean ± SEM, a p < 0.05, compared with those injected with AGEs-rat serum albumin (RSA group). b p < 0.05, compared with the AGEs group. ab p < 0.05 compared with the RSA group and AGEs group.
Effect of advanced glycation end-products on placental permeability and the tight junctions of rat placenta
EB, an azodye with a high affinity for plasma proteins, was used to evaluate placental permeability. In the present research, placental permeability was assessed by measuring EB levels of placentas in each group. Results in Fig. 2A indicate that AGEs increased the placental permeability of rats, and RAGE antagonist FPS-ZM1 and sRAGE abolished this effect of AGEs.
Figure 2: Effect of advanced glycation end-products on placental permeability and the tight junction (TJ) of rat placenta (A) AGEs improved the content of Evans-Blue (EB) in the placenta, FPS-ZM1 and soluble receptors of AGE (sRAGE) deprived AGEs of their capability to increase the content of EB in the placenta (n = 9). (B) AGEs destroyed the ultrastructure of the placenta, while AGEs antagonist FPS-ZM1 and sRAGE restored placental integrity. (C–F) quantitative polymerase chain reaction, western blotting, and immunohistochemical analyses showed that AGEs increased the expression of TJ-related genes. Meanwhile, FPS-ZM1 and sRAGE blocked the effect of AGEs (n = 3). The data represent the mean ± SEM, a p < 0.05, compared with the RSA group. b p < 0.05, compared with the AGEs group. c p < 0.05 compared with AGEs+FPS-ZM1 group. ab p < 0.05 compared with those injected with AGEs-rat serum albumin (RSA group) and the AGEs group. abc p < 0.05 compared with the RSA group, AGEs group, and AGEs+FPS-ZM1 group. Scale bar: 100 μm.
Further, the ultrastructure of the placenta visualized by transmission electron microscopy (Fig. 2B) demonstrated that AGEs destroyed the integrity of the placenta (the area indicated by the black arrow in Fig. 2B), with continuous and dense, tightly connected structures in the RSA group, while most of the tightly connected structures between syncytial trophoblast cells and vascular endothelial cells in placental tissues were lost, and some cells were degenerated and disintegrated in AGEs group. Meanwhile, FPS-ZM1 and sRAGE protected the placenta from this damaging effect of AGEs.
Then, the TJ-related genes ZO-1, Occludin, and Claudin5 expression levels were detected by quantitative polymerase chain reaction (qPCR) (Fig. 2C), western blotting (Figs. 2D and 2E), and immunohistochemical (IHC) staining (Fig. 2F); the results demonstrate that AGEs decreased the expression of TJ-related genes, simultaneously FPS-ZM1 and sRAGE antagonist the effect of AGEs.
Effect of advanced glycation end-products (AGEs) on the expression of receptors of AGE and nuclear factor-κB in rat placenta
To assess the mechanism of the effect of AGEs on placental integrity, expression of RAGE and NF-κB in the placenta were examined. Compared with the RSA group, AGEs treatment sharply upregulated the expression of genes and proteins of RAGE and NF-κB in the placenta (Figs. 3A–3C). This AGEs-induced increase in RAGE and NF-κB expression was ameliorated by treatment with FAM-ZM1 and sRAGE.
Figure 3: Advanced glycation end-products (AGEs) enhanced RAGE and NF-κB expression in the placenta of rats (A–C) quantitative polymerase chain reaction (qPCR) and western blotting quantification showed that AGEs increased RAGE protein level in the rat placenta. FPS-ZM1 and sRAGE abolished the AGEs-induced increase in RAGE expression (n = 3). (D–F) qPCR and western blotting quantification show that AGEs increased the NF-κB expression. FPS-ZM1 and sRAGE restored AGEs-induced increase in NF-κB level (n = 3). a p < 0.05, compared with those injected with AGEs-rat serum albumin (the RSA group). b p < 0.05, compared with the AGEs group. c p < 0.05 compared with AGEs+FPS-ZM1 group. ab p < 0.05 compared with the RSA group and AGEs group.
Effect of advanced glycation end-products on the expression of VEGF in rat placenta
To investigate the mechanism of damage induced by AGEs, to placental TJ, the expression of VEGF in the placenta was determined by using qPCR and western blotting and the results (Fig. 4) demonstrate that AGEs enhanced VEGF expression in rat placenta, and this effect of AGEs was abolished by FPS-ZM1 and sRAGE.
Figure 4: Advanced glycation end-products (AGEs) improved the expression of vascular endothelial growth factor (VEGF) in the placenta of rats (A–C) qPCR and western blotting with quantification results showed that AGEs elevated the level of VEGF in rat placenta. FPS-ZM1 and sRAGE ameliorated the AGEs-induced increase in the level of RAGE AGEs (n = 3). a p < 0.05, compared with those injected with AGEs-rat serum albumin (the RSA group). b p < 0.05, compared with the AGEs group. c p < 0.05 compared with AGEs+FPS-ZM1 group. ab p < 0.05 compared with the RSA group and AGEs group.
AGEs are a group of highly active end products formed by non-enzymatic glycosylation between the amino group of a protein, fatty acid, or nucleic acid and the aldehyde group of reducing sugar. In our previous study, we found that the placental permeability of GDM model rats was significantly higher than that of the control group, accompanied by increased serum levels of AGEs and changes in the expression of placental TJ proteins (Shi et al., 2020a). In this study, we found significantly increased permeability of the placenta of AGEs-treated rats, and observation under transmission electron microscopy revealed widened gaps and flawed TJ structure. In addition, the expression of TJ factors ZO-1, Occludin, and Claudin5 decreased in AGEs administrated rats, while RAGE, NF-κB, and VEGF levels increased significantly. However, AGEs exerted no effect on the blood glucose of rats, suggesting that in the absence of a hyperglycemic environment, AGEs can increase placental permeability and decrease the expression of TJ factors, impairing placental barrier and function. The damaging alterations in the placental barrier are very similar to those observed in the rat models with GDM, and the AGEs are an independent factor of placental damage in addition to hyperglycemia. Most recent hypotheses suggest that high level of AGEs is not only a concomitant response to diabetic hyperglycemia but that it can result from a variety of diseases such as diet and obesity (Edeas et al., 2010). In turn, high AGEs levels can induce oxidative stress in vivo, cause pancreatic β-cell dysfunction, reduce insulin secretion, and consequently insulin resistance, thus participating in the development of diabetes (Vlassara and Uribarri, 2014). In summary, we postulate that the presence of a long-term sustained hyperglycemic environment in GDM patients could increase the exposure of AGEs, thus activating intracellular oxidative stress and inflammatory response through RAGE, accelerating the formation of AGEs, further aggravating oxidative stress and inflammatory response, and decreasing insulin resistance, to finally end up promoting GDM progression.
EB is a dye with a high affinity for albumin and cannot pass through endothelial cells; therefore, the binding of EB to albumin is limited to the vasculature. Under some pathological conditions that increase vascular permeability, endothelial cells partially lose their TJs and allow the passage of small molecules such as albumin, thus allowing EB to leak into the tissue. In this experiment, EB was injected into the tail vein of rats at E16, and the placenta was removed by cesarean section to examine the change in EB content in the placenta of each group to evaluate the placental permeability. FPS-ZM1 is a high-affinity RAGE-specific inhibitor, which is non-toxic to mice, and blocks the binding of the RAGE ligands to its V region, and can easily cross the blood-brain barrier (Deane et al., 2012). The protective effects of FPS-ZM1 have been demonstrated in a variety of animal models. In Alzheimer’s disease model mice, FPS-ZM1 improved neuronal apoptosis and behavioral performance while inhibiting the expression of RAGE and NF-κB (Wang et al., 2018). FPS-ZM1, in combination with valsartan in the treatment of a diabetic rats model, also exhibited a potential for renal tubular injury protection by inhibiting renal inflammation and oxidative stress (Sanajou et al., 2019). Therefore, FPS-ZM1 is expected to be a potential drug for the prevention and treatment of AGEs-RAGE-related diseases. In this study, the permeability and TJ in the placenta of rats with GDM did not change after treatment with 1.0 mg/kg AGEs-RSA when the rats were pretreated with the same dosage of FPS-ZM1 daily, and the expression of RAGE and NF-κB reduced significantly. This protective effect of FPS-ZM1 on placental barrier damage caused by AGEs intervention was similar to that observed in our previous study on the effect of FPS-ZM1 on the placenta of GDM rats. Thus, AGEs are an important element in placental barrier dysfunction under the GDM state; they act mainly by activating RAGE. After AGEs-RSA intervention with concomitant administration of recombinant sRAGE at 5 mg/kg/day in gestational rats, the placenta did not show the elevated permeability presented after AGEs-RSA intervention alone and significantly restored the expression of TJ factor, while also downregulating the expression of RAGE and NF-κB. Gene expression is a complex process that is regulated at many levels; after gene transcription, processes such as protein translation, degradation, and post-translational modifications affect the level of protein (Buccitelli and Selbach, 2020). We found that the expression of NF-κB transcripts in the AGEs-sRAGE group was lower than in the RSA group, but the expression of NF-κB protein in the AGEs-sRAGE group was higher than in the RSA group. We thought that this may be because AGEs and sRAGE affected the processes after NF-κB gene transcription. sRAGE, a soluble RAGE, blocks the binding of RAGEs to AGEs in vivo by binding to free AGEs in blood and tissues, thereby mitigating the adverse effects of AGEs-RAGE interactions. Circulating levels of sRAGE decreased strikingly at the end of pregnancy in rats. In addition, newborns had significantly higher levels of sRAGE than their mothers, indicating that the compound, which is derived from the placenta, may exert anti-inflammatory effects by decreasing the effects of AGEs, which in turn may have a protective effect on the fetus (Quintanilla-García et al., 2019). Currently, low levels of serum sRAGE or a high ratio of AGEs/sRAGE have been proposed as biomarkers of diabetes, hyperthyroidism, hyperccholesterolemia and end-stage renal disease (Prasad, 2019). However, there are very few studies on the relationship between sRAGE and pregnancy complications. Therefore, sRAGE acts as a protective antibody against the damaging effects of AGEs-RAGE in vivo and is expected to be developed as a predictor of complications in pregnancy. Here, the protective effect of sRAGE on placental barrier injury after AGEs intervention was similar to the effect of sRAGE on the placenta of GDM rats. Thus, sRAGE reduced tissue damage by binding to free AGEs and blocked their binding to RAGE in tissues. Similar to FPS-ZM1, sRAGE protected the placental barrier, but sRAGE was not significantly superior to FPS-ZM1 in terms of improvement in EB leakage from the placenta or reversal of TJ-related genes expression, and we, therefore, hypothesized that RAGE receptor-mediated pathway is the primary mode through which AGEs induce impairment of placental barrier function. Thus, AGEs-induced impairment of placental barrier and TJs was mainly through the RAGE/NF-κB pathway.
In conclusion, AGEs can induce abnormal expression of placental TJ-related genes through the RAGE/NF-κB signaling pathway in the gestational state, damaging placental TJ structures, disrupting the integrity of the barrier, and increasing placental permeability (Fig. 5). The adverse effect of AGEs may be involved in the development of gestational diabetes and is an important factor causing poor prognosis for the mother and child. AGEs and sRAGE are promising as biomarkers for some complications of pregnancy, such as GDM and preeclampsia, and are used as clinical predictors and intervention indicators.
Figure 5: Advanced glycation end-products (AGEs) induce abnormal expression of placental tight junctions (TJ) related genes in the gestational state. RAGE, receptors of AGEs; NF-κB, nuclear factor-κB; VEGF, vascular endothelial growth factor.
Availability of Data and Materials: The datasets generated during and/or analysed during the current study are available from the corresponding author on reasonable request.
Author Contribution: The authors confirm contribution to the paper as follows: study conception and design: DDS and HY; data collection: YH and QYY; analysis and interpretation of results: DYQ; draft manuscript preparation: QL and WQ. All authors reviewed the results and approved the final version of the manuscript.
Ethics Approval: All experimental procedures were approved and supervised by the Ethics Committee of Southeast University, with file code: 20200407023 in April 07, 2020. All efforts were made to minimize animal suffering.
Funding Statement: This work was financially supported by The Jiangsu Provincial Maternal and Child Health Key Talents Project (F202042).
Conflicts of Interest: The authors declare that they have no known competing financial interests or personal relationships that could have appeared to influence the work reported in this paper.
References
Aires MB, Dos Santos AC (2015). Effects of maternal diabetes on trophoblast cells. World Journal of Diabetes 6: 338–344. DOI 10.4239/wjd.v6.i2.338. [Google Scholar] [CrossRef]
Bartakova V, Kollarova R, Kuricova K, Sebekova K, Belobradkova J, Kankova K (2016). Serum carboxymethyl-lysine, a dominant advanced glycation end product, is increased in women with gestational diabetes mellitus. Biomedical Papers of the Medical Faculty of the University Palacký, Olomouc, Czechoslovakia 160: 70–75. DOI 10.5507/bp.2015.045. [Google Scholar] [CrossRef]
Baumüller S, Lehnen H, Schmitz J, Fimmers R, Müller AM (2015). The impact of insulin treatment on the expression of vascular endothelial cadherin and β-catenin in human fetoplacental vessels. Pediatric and Developmental Pathology 18: 17–23. DOI 10.2350/13-11-1400-OA.1. [Google Scholar] [CrossRef]
Boulanger E, Grossin N, Wautier MP, Taamma R, Wautier JL (2007). Mesothelial RAGE activation by AGEs enhances VEGF release and potentiates capillary tube formation. Kidney International 71: 126–133. DOI 10.1038/sj.ki.5002016. [Google Scholar] [CrossRef]
Citi S, Guerrera D, Spadaro D, Shah J (2014). Epithelial junctions and Rho family GTPases: The zonular signalosome. Small GTPases 5: 1–15. DOI 10.4161/21541248.2014.973760. [Google Scholar] [CrossRef]
Deane R, Singh I, Sagare AP, Bell RD, Ross NT et al. (2012). A multimodal RAGE-specific inhibitor reduces amyloid β-mediated brain disorder in a mouse model of Alzheimer disease. Journal of Clinical Investigation 122: 1377–1392. DOI 10.1172/JCI58642. [Google Scholar] [CrossRef]
Edeas M, Attaf D, Mailfert AS, Nasu M, Joubet R (2010). Maillard reaction, mitochondria and oxidative stress: Potential role of antioxidants. Pathologie Biologie 58: 220–225. DOI 10.1016/j.patbio.2009.09.011. [Google Scholar] [CrossRef]
Furuse M, Sasaki H, Fujimoto K, Tsukita S (1998). A single gene product, claudin-1 or -2, reconstitutes tight junction strands and recruits occludin in fibroblasts. Journal of Cell Biology 143: 391–401. DOI 10.1083/jcb.143.2.391. [Google Scholar] [CrossRef]
Guosheng L, Hongmei S, Chuan N, Haiying L, Xiaopeng Z, Xianqiong L (2009). The relationship of serum AGE levels in diabetic mothers with adverse fetal outcome. Journal of Perinatology 29: 483–488. DOI 10.1038/jp.2009.12. [Google Scholar] [CrossRef]
Harsem NK, Braekke K, Torjussen T, Hanssen K, Staff AC (2008). Advanced glycation end products in pregnancies complicated with diabetes mellitus or preeclampsia. Hypertension in Pregnancy 27: 374–386. DOI 10.1080/10641950802000968. [Google Scholar] [CrossRef]
Jarmuzek P, Wielgos M, Bomba-Opon D (2015). Placental pathologic changes in gestational diabetes mellitus. Neuro Enocrinology Letters 36: 101–105. [Google Scholar]
Leach L, Lammiman MJ, Babawale MO, Hobson SA, Bromilou B, Lovat S, Simmonds MJ (2000). Molecular organization of tight and adherens junctions in the human placental vascular tree. Placenta 21: 547–557. DOI 10.1053/plac.2000.0541. [Google Scholar] [CrossRef]
Leach L, Taylor A, Sciota F (2009). Vascular dysfunction in the diabetic placenta: Causes and consequences. Journal of Anatomy 215: 69–76. DOI 10.1111/j.1469-7580.2009.01098.x. [Google Scholar] [CrossRef]
Ohtsuki S, Sato S, Yamaguchi H, Kamoi M, Asashima T, Terasaki T (2007). Exogenous expression of claudin-5 induces barrier properties in cultured rat brain capillary endothelial cells. Journal of Cellular Physiology 210: 81–86. DOI 10.1002/(ISSN)1097-4652. [Google Scholar] [CrossRef]
Prasad K (2019). Is there any evidence that AGE/sRAGE is a universal biomarker/risk marker for diseases? Molecular and Cellular Biochemistry 451: 139–144. DOI 10.1007/s11010-018-3400-2. [Google Scholar] [CrossRef]
Quintanilla-García CV, Uribarri J, Fajardo-Araujo ME, Barrientos-Romero JJ, Romero-Gutiérrez G, Reynaga-Ornelas MG, Garay-Sevilla ME (2019). Changes in circulating levels of carboxymethyllysine, soluble receptor for advanced glycation end products (sRAGEand inflammation markers in women during normal pregnancy. The Journal of Maternal-Fetal & Neonatal Medicine 32: 4102–4107. DOI 10.1080/14767058.2018.1481948. [Google Scholar] [CrossRef]
Radu M, Chernoff J (2013). An in vivo assay to test blood vessel permeability. Journal of Visualized Experiments, e50062. DOI 10.3791/50062. [Google Scholar] [CrossRef]
Saitou M, Furuse M, Sasaki H, Schulzke JD, Fromm M, Takano H, Noda T, Tsukita S, Nelson WJ (2000). Complex phenotype of mice lacking occludin, a component of tight junction strands. Molecular Biology of the Cell 11: 4131–4142. DOI 10.1091/mbc.11.12.4131. [Google Scholar] [CrossRef]
Sanajou D, Ghorbani Haghjo A, Argani H, Roshangar L, Rashtchizadeh N et al. (2019). Reduction of renal tubular injury with a RAGE inhibitor FPS-ZM1, valsartan and their combination in streptozotocin-induced diabetes in the rat. European Journal of Pharmacology 842: 40–48. DOI 10.1016/j.ejphar.2018.10.035. [Google Scholar] [CrossRef]
Shen L, Weber CR, Raleigh DR, Yu D, Turner JR (2011). Tight junction pore and leak pathways: A dynamic duo. Annual Review of Physiology 73: 283–309. DOI 10.1146/annurev-physiol-012110-142150. [Google Scholar] [CrossRef]
Sibley CP, Brownbill P, Glazier JD, Greenwood SL (2018). Knowledge needed about the exchange physiology of the placenta. Placenta 64 Suppl 1: S9–S15. [Google Scholar]
Shi Y, Qian J, Zhang F, Jia B, Liu X, Hu Y, Zhang Q, Yang Y, Sun D, Jiang L (2020a). Low molecular weight heparin (nadroparin) improves placental permeability in rats with gestational diabetes mellitus via reduction of tight junction factors. Molecular Medicine Reports 21: 623–630. [Google Scholar]
Shi Y, Qian J, Zhang F, Hu Y, Sun D, Jiang L (2020b). Advanced glycation end products increased placental vascular permeability of human BeWo cells via RAGE/NF-kB signaling pathway. European Journal of Obstetrics & Gynecology and Reproductive Biology 250: 93–100. DOI 10.1016/j.ejogrb.2020.04.058. [Google Scholar] [CrossRef]
Sobrevia L, Salsoso R, Fuenzalida B, Barros E, Toledo L et al. (2016). Insulin is a key modulator of fetoplacental endothelium metabolic disturbances in gestational diabetes mellitus. Frontiers in Physiology 7: 119. DOI 10.3389/fphys.2016.00119. [Google Scholar] [CrossRef]
Sobrevia L, Salsoso R, Sáez T, Sanhueza C, Pardo F, Leiva A (2015). Insulin therapy and fetoplacental vascular function in gestational diabetes mellitus. Experimental Physiology 100: 231–238. DOI 10.1113/expphysiol.2014.082743. [Google Scholar] [CrossRef]
Subiabre M, Silva L, Toledo F, Paublo M, López MA, Boric MP, Sobrevia L (2018). Insulin therapy and its consequences for the mother, foetus, and newborn in gestational diabetes mellitus. Biochimica et Biophysica Acta-Molecular Basis of Disease 1864: 2949–2956. DOI 10.1016/j.bbadis.2018.06.005. [Google Scholar] [CrossRef]
van Meer G, Simons K (1986). The function of tight junctions in maintaining differences in lipid composition between the apical and the basolateral cell surface domains of MDCK cells. The EMBO Journal 5: 1455–1464. DOI 10.1002/j.1460-2075.1986.tb04382.x. [Google Scholar] [CrossRef]
van der Aa EM, Peereboom-Stegeman JH, Noordhoek J, Gribnau FW, Russel FG (1998). Mechanisms of drug transfer across the human placenta. Pharmacy World & Science 20: 139–148. DOI 10.1023/A:1008656928861. [Google Scholar] [CrossRef]
Vlassara H, Uribarri J (2014). Advanced glycation end products (AGE) and diabetes: Cause, effect, or both? Current Diabetes Reports 14: 453. DOI 10.1007/s11892-013-0453-1. [Google Scholar] [CrossRef]
Wang H, Chen F, Du YF, Long Y, Reed MN, Hu M, Suppiramaniam V, Hong H, Tang SS (2018). Targeted inhibition of RAGE reduces amyloid-β influx across the blood-brain barrier and improves cognitive deficits in db/db mice. Neuropharmacology 131: 143–153. DOI 10.1016/j.neuropharm.2017.12.026. [Google Scholar] [CrossRef]
Xie J, Méndez JD, Méndez-Valenzuela V, Aguilar-Hernández MM (2013). Cellular signalling of the receptor for advanced glycation end products (RAGE). Cellular Signalling 25: 2185–2197. DOI 10.1016/j.cellsig.2013.06.013. [Google Scholar] [CrossRef]
Cite This Article
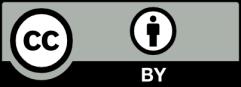
This work is licensed under a Creative Commons Attribution 4.0 International License , which permits unrestricted use, distribution, and reproduction in any medium, provided the original work is properly cited.