Open Access
REVIEW
Presenilin and Alzheimer’s disease interactions with aging, exercise and high-fat diet: A systematic review
Ludong University, Yantai, 264025, China
* Corresponding Author: Dengtai Wen,
BIOCELL 2023, 47(1), 41-49. https://doi.org/10.32604/biocell.2022.022689
Received 21 March 2022; Accepted 27 June 2022; Issue published 26 September 2022
Abstract
Presenilin (Psn) protein is associated with organismal aging. Mutations in the Psn gene may lead to Alzheimer’s disease (AD), dilated cardiomyopathy (DCM), and many age-dependent degenerative diseases. These diseases seriously affect the quality of life and longevity of the population and place a huge burden on health care and economic systems around the world. Humans have two types of Psn, presenilin-1 (PSEN1) and presenilin-2 (PSEN2). Mutations in the genes encoding PSEN1, PSEN2, and amyloid precursor protein (APP) have been identified as the major genetic causes of AD. Psn is a complex gene strongly influenced by genetic and environmental factors. The effects of exercise, training, and a high-fat diet on the Psn gene expressed in the heart and its related pathways are not fully understood. Fortunately, relevant aspects of the mutational effects on Psn can be studied experimentally in easily handled animal models, including Drosophila, mice, and other animals, all of which share orthologous genes of Psn with humans. Many previous studies have linked aging, exercise training, and a high-fat diet to the Psn gene. This review discusses the interrelationship between aging, exercise training, and a high-fat diet on the Psn gene and its associated disease, AD. The aim is to understand the adverse effects of Psn gene mutations on the body and the diseases caused by AD, find ways to alleviate the adverse effects and provide new directions for the improvement of treatment strategies for diseases caused by Psn gene mutations.Keywords
Population aging phenomenon exists in most developed countries, even some developing countries. The world’s aging population is increasing continuously at a high rate. The aging of the body is accompanied by a variety of age-dependent degenerative diseases such as Alzheimer’s disease (AD) and dilated cardiomyopathy (DCM). Research has shown that mutations in the Presenilin (Psn) gene have been found in both AD and DCM (Li et al., 2011a). Research has also shown that mutations in the Psn gene and a high-fat diet accelerate the development of aging-related diseases. On the contrary, exercise training and a balanced diet can delay the aging process and reduce the risk of aging-related diseases (Marcon et al., 2009). However, there are few reports on the effects of exercise training and a high-fat diet on the Psn gene and related pathways.
Psn is a multichannel transmembrane protein, an intramembrane protease complex that catalyzes the intramembrane cleavage of intact membrane proteins such as Notch receptors (Guo et al., 1999). Psn is located in the apical plasma membrane, late endosomes, and recycling endosomes. It is an integral component of the plasma membrane (Ankarcrona and Hultenby, 2002). It is expressed in multiple structures, including the anterior and posterior subdivision of the organism and the central nervous system, and is essential for the study of AD and DCM (Lehmann et al., 1997). Psn is a γ-catalytic component of the secretase intramembrane protease complex; other non-catalytic Psn roles are also found in cellular signaling processes, including calcium homeostasis, lysosomal acidification, autophagy, and protein transport (Song et al., 2013). Vertebrates have two Psn genes, presenilin-1 (PSEN1) and presenilin-2 (PSEN2). PSEN1 is a macromolecular protein on the endoplasmic reticulum and Golgi apparatus. PSEN1 is, additionally, a transmembrane protein that forms a complex with amyloid precursor protein (APP) in the cell and is involved in APP transport and post-synthesis processing (Raemaekers et al., 2005). With age, PSEN1 increases in the human brain, thereby affecting memory in the elderly (Culvenor et al., 2004). Mutations in the PSEN1 gene, which encodes this protein, are thought to be closely associated with the development of AD (Blanchard et al., 1997). Patients with hereditary AD carry PSEN1 and PSEN2 or APP. These disease-related mutations lead to an increase in amyloid-beta (Aβ) peptides (Villegas et al., 2007). Both PSEN1 and PSEN2 are proteins with anti-apoptotic effects.
Age-dependent neurodegenerative diseases affect millions of people worldwide (Arora and Ligoxygakis, 2020). Psn mutations are not only an important cause of age-dependent neurodegenerative diseases but also have many adverse effects on the body (Han et al., 2021). In this review, we will systematically explore the interaction of Psn and AD with aging, exercise training and a high-fat diet, hoping to find ways to alleviate the adverse effects through dietary changes and exercise training. Through this study, we aim to provide new directions for the improvement of complementary treatment strategies for Psn mutation-related diseases, thereby improving the quality of life and longevity and reducing the burden on the economy and health care systems around the world.
PSEN1 and PSEN2 genes are expressed in various human organs such as the brain and heart (Bruni, 1998). In the brain, Psn is found in neuronal cells in the hippocampus and cerebral cortex associated with learning and memory (Lee et al., 1996). Psn has a general and essential role in the survival of excitatory and inhibitory neurons during aging (Kang and Shen, 2020). Genetic studies in mice suggest that Psn regulates neurodevelopment in the developing brain through the Notch signaling pathway (Kim and Shen, 2008). Specific loss of PSEN1 in the forebrain of mice affects specific aspects of memory (Feng et al., 2001). PSEN1 plays an important role in brain development and neuronal function, which is related to the brain-specific pathological role of PSEN1 mutations (Hartmann et al., 1997). PSEN1 and PSEN2 are expressed in the heart and its role in the heart has been discussed earlier (Levy-Lahad et al., 1996). Mutations in the Psn gene are thought to be involved in pathological changes in the heart (Yang et al., 2020). PSEN1 can act as an important regulator of cardiac Ca2+ pump function with complex stimulatory/inhibitory properties (Bovo et al., 2021). PSEN1 is associated with apoptosis and cardiac development, and PSEN1 mutations trigger enlarged ventricular chambers and systolic dysfunction (Li et al., 2011b). Abnormalities in the dynamic regulation and function of PSEN1 lead to abnormal cardiomyocyte ultrastructure and cardiovascular disease (CVD) (Song et al., 2018). In contrast, PSEN2 is ubiquitous in various organ tissues, including the heart, and plays an important role in cardiac excitation-systole coupling by interacting with the cardiac ryanodine receptor (RyR2) (Takeda et al., 2005).
PSEN1 and PSEN2 genes are necessary for the survival of adult neurons, and mutation in this gene affects the aging of the organism. Aging is characterized by a deterioration of cellular function and physical health over time, accompanied by an increased susceptibility to disease (Goldsmith, 2014). Psn has a role in promoting neuronal activity in the brain, and the knockdown of Psn gene leads to an increase in the likelihood of neuronal death (Kang et al., 2017). AD is the most common form of dementia, accounting for more than half of dementia cases (Gaugler et al., 2022). AD is an age-related neurological disease that is one of the leading causes of death and disability worldwide (Small et al., 1997). It is an irreversible neurodegenerative disease characterized by insidious onset and slow progression (Hashimoto et al., 2005). In addition, AD is the most common type of chronic neurodegenerative disease among the elderly and is clinically characterized by progressive memory decline (Zhuang et al., 2020). In general, the clinical manifestations of AD are mainly characterized by anterograde episodic memory disorder. This condition is often accompanied by multiple cognitive impairments, such as visuospatial, language, and executive function (Chan et al., 2013). The combination of the above features can lead to global cognitive decline, eventually leading to a state of total dependence and, ultimately, death (Bowman and Quinn, 2008). The above studies show that AD can cause serious damage to the body, so it is crucial to study the treatment strategies for AD by exploring Psn-related literature.
AD caused by Psn mutations can have many adverse effects on the organism. Both AD and familial AD (FAD) are affected by changes in Psn levels. Studies on cultured nerve cells have shown that mutations in the PSEN1 gene lead to disturbances in cellular calcium homeostasis, and many early-onset familial AD (EOFAD) is caused by mutations in this gene (Mattson et al., 2000). Studies have shown that mutations in a single copy of the Psn and APP may contribute to the development of FAD. Only normal Psn levels can maintain normal cognition throughout the lifespan. Therefore, the decrease in PSEN1 and PSEN2 functional activity may be related to the pathogenesis of FAD. A decrease in Psn function was found to lead to age-related cognitive deficits (Nagakura et al., 2013). Some aspects of FAD and AD may be caused by the decreased activity level of Psn (McBride et al., 2010). It is, hence, apparent that Psn is closely related to the generation of AD, and its low levels largely lead to memory deficit and cognitive impairment, resulting in the occurrence of FAD and AD.
A close relationship between Psn and aging can be found in studies using mice as a model. Psn plays an important role in the growth and development of embryos, and the inactivation of PSEN1 may lead to developmental defects and eventual perinatal death in mice (Donoviel et al., 1999). Studies have shown that the interaction between the AD-associated protein PSEN1 and the synaptic vesicular protein SYT-1 is increased during normal aging of the mouse brain and neuronal aging in vitro (Keller et al., 2020). Therefore, studies on mice have found that changes in Psn level can affect normal learning and memory, growth and development, and even the conditions necessary for the survival of mice.
A study using Drosophila as a model found that Psn is extremely closely related to growth, development, and aging. In Drosophila melanogaster, Psn is expressed at different developmental stages, and the expression level in the adult is higher than that in the larvae, mainly in the central nervous system. Mutations in the Psn gene are the most common cause of EOFAD. PSEN1 mutations account for 18%–50% of EOFAD cases, while PSEN2 mutations are rare. PSEN1 is uniformly expressed, and PSEN2 is confined to the heart, skeletal muscle, and pancreas (Zheng et al., 2015). It is thus clear that Psn plays an important role in growth and development and mainly acts on mature individuals. The loss of Psn function in D. melanogaster increases the level of apoptosis in developing tissues (Ye and Fortini, 1999). It is evident that both Psn knockdown and overexpression can lead to apoptosis. RNA interference (RNAi) to stall the expression of the Drosophila ubiquitin homolog (dUbqln) enhances retinal degeneration caused by Psn overexpression (Li et al., 2007). Overexpression of Psn in the retina leads to a smaller eye phenotype (Reynolds-Peterson et al., 2020). Taking into account the aforementioned studies, it is, therefore, demonstrated that Psn gene mutations can alter the phenotype of Drosophila eyes and also lead to serious consequences such as apoptosis.
PSEN1 and PSEN2 play an important role in the regulation of cardiovascular function. PSEN2 promotes heart excitation-contraction by directly coupling with RyR2 (Takeda et al., 2005). The morphology of the heart in mice with PSEN1 gene mutation exhibits ventricular septal defects and a double outlet of the right ventricular (Nakajima et al., 2004). The incidence of heart dysfunction and arrhythmias in the hearts of senescent Drosophila increases significantly with age (Ocorr et al., 2007). Cardiovascular stimulation in Drosophila revealed a negative correlation between age and maximum heart rate, that being smaller in older Drosophila (Paternostro et al., 2001). It is thus clear that Psn plays an important physiological role in the heart.
Psn gene is closely related to the development and function of the heart. The mutations in the Psn gene lead to EOFAD and DCM, both of which accelerate cardiac aging (Cannon and Bodmer, 2016). The etiology of AD is mainly idiopathic, and in particular, autosomal dominant genetic disorders caused by mutations in the PSEN1 and PSEN2 genes are thought to be the main cause of FAD (Yang et al., 2020). Psn knockdown can lead to a significant decrease in heart rate, while the opposite occurs with Psn overexpression (Li et al., 2011a). Research has found a strong correlation between AD and heart insufficiency (Tublin et al., 2019). Mutations in PSEN1 and PSEN2 may lead to an increased risk of cardiac systolic and diastolic dysfunction in patients with AD (Yang et al., 2020). Research has shown that PSEN1 and PSEN2 mutations are associated with DCM and heart failure (Li et al., 2006). Therefore, these studies suggest that mutations in the Psn gene lead to abnormal heart development, dysfunction, and accelerated cardiac aging.
Alzheimer’s disease and muscle aging
Psn mutation-induced AD is often accompanied by muscle atrophy and aging. With age and muscle atrophy, the onset and progression of AD may be accelerated and accompanied by weight loss. One of the main features of aging is the progressive loss of skeletal muscle function and a gradual decrease in skeletal muscle mass called skeletal sarcopenia (Demontis et al., 2013a). Studies have shown that muscle changes play an important role in common diseases (Wolfe, 2006). Muscle aging is associated with a gradual decline in muscle quantity and mass (Kim et al., 2021). Clinical observation shows that skeletal muscle can affect central nervous system aging, and neurodegeneration of the central nervous system is a decisive characteristic of body aging affected by peripheral tissue (Rai et al., 2021). With the increase in age, the function of multiple organ systems gradually declines, and skeletal muscle atrophy is one of the main physiological problems of the elderly. Studies have shown that elderly APP/PS1 double transgenic mice (APP/PS1 double transgenic mice is an AD transgenic animal model established by transferring amyloid precursor protein (APP) and PSEN1 mutant genes into mice.) demonstrating phenotypes of lower body weight, less muscle tissue, increased myostatin expression, lower muscle strength, and reduced myostatin expression show AD-related memory impairment (Lin et al., 2019). This progressive muscle wasting can lead to the progression of chronic diseases such as metabolic complexes, cancer, and AD (Ruiz et al., 2008). Thus, as the organism gradually ages, the possibility of neurodegenerative pathologies of the central system, like AD, increases. The onset of these diseases is often accompanied by a progressive decrease in skeletal muscle function and quality.
Muscle levels, in turn, affect the progression of AD. People with higher muscle strength levels are at less risk of developing AD than those with lower muscle strength levels (Boyle et al., 2009). Sudden weight loss is one of the signs of dementia in the elderly, often occurring in AD patients (Grundman, 2005), and weight loss can predict AD (Luchsinger and Gustafson, 2009). People with AD lose muscle at a faster rate than normal people (Burns et al., 2010). Nutrition and stress perception in skeletal muscle affect the lifespan and overall aging of the body, and actin in the muscle affects the progression of age-dependent diseases, such as AD (Demontis et al., 2013b). Therefore, weight changes are one of the signs to determine whether or not dementia is present. If an older person experiences a sudden loss of weight, it can be considered a sign of dementia onset.
Alzheimer’s disease and exercise training
Exercise training can mitigate the adverse effects of AD on the organism. AD is a progressive neurodegenerative disease for which there are few effective treatments (Um et al., 2008). Physical exercise is used to treat AD by eliciting positive neurophysiological effects (Garcia-Mesa et al., 2011). Aerobic exercise can slow the progressive decline of older adults as they age (Young et al., 2015). Physical exercise improves cognitive performance (da Silva et al., 2018). Therefore, active physical exercise can reduce the likelihood of cognitive impairment in older adults (de la Rosa et al., 2020). Exercise also slows the progression of AD by improving mitochondrial function and REDOX homeostasis (Teglas et al., 2020). Regular running is beneficial for people who have traditional cardiovascular risk factors (Tapia-Rojas et al., 2016). Studies have shown that exercise may also affect Aβ levels by modulating the immune response of AD patients (Nichol et al., 2008). Exercise training improves cognitive function in AD patients (Zhang et al., 2017). Hence, regular exercise training has a series of positive effects on AD.
Evidence shows that exercise training can enhance heart function and is beneficial in delaying cardiac aging and reducing the occurrence of heart failure (Lai et al., 2014). Physical exercise can also improve cardiac diastolic dysfunction, reduce lipid overaccumulation, reduce oxidative damage, and to some extent, improve the mobility and life span of Drosophila (Wen et al., 2019). Endurance exercise promotes health and longevity, while chronic endurance training also prevents disease, improves heart, skeletal muscles, and brain functions, and reduces obesity, heart disease, and cognitive decline (Sujkowski et al., 2020). Running is an effective way to delay cardiac aging (Wen et al., 2021). Thus, exercise training may alleviate cardiac aging and its adverse effects.
In animal models of AD, studies conducted on mice have shown the protective effect of exercise training on the organism (Kim et al., 2019). Exercise improved short-term recognition memory and spatial learning and memory ability, and restored neuronal excitability of APP/PS1 double transgenic mice (Tan et al., 2020). Exercise also improves the exploration ability (Li et al., 2019). And running reduced brain inflammation (Falkenhain et al., 2020) in APP/PS1 double transgenic mice. In addition, voluntary exercise prevented mitochondrial dysfunction in AD, enhanced mitochondrial autophagic activity in the hippocampus, and effectively improved the pathological phenotype of APP/PS1 transgenic mice (Zhao et al., 2020). Regular treadmill exercise plays a neuroprotective role in age-related memory loss (Zeng et al., 2020). In conclusion, exercise training alleviates age-dependent degenerative diseases such as AD and improves cognitive and neural activities.
A combined intervention of treadmill exercise and dietary polyphenols improves cognitive loss in APP/PS1 double transgenic mice and has a therapeutic effect on AD (Zhang et al., 2016). Running has been found to inhibit the deposition of Aβ plaques in the hippocampus, one of the main pathological markers of AD in APP/PS1 double transgenic mice (Xia et al., 2019). Treadmill exercise reduces hippocampal neuron loss in APP/PS1 double transgenic mice (Zhang et al., 2019). Running also improves the structure and function of the hippocampus and amygdala-related neurons in APP/PS1 double transgenic mice (Lin et al., 2015). In addition, exercise combined with probiotic supplementation can further delay the progression of AD in APP/PS1 double transgenic mice (Abraham et al., 2019).
Sports training is categorized into voluntary and passive exercises that bring about different degrees of training effect; besides, their impact on AD is also not the same. In a transgenic mouse model of AD, voluntary exercise significantly reduced Aβ load (Adlard et al., 2005). Long-term treadmill exercise can better delay the progression of AD neuropathology in the hippocampus of APP/PS1 double transgenic mice (Liu et al., 2013). The effect of voluntary exercise in improving memory impairment due to AD is better than passive exercise training (Yuede et al., 2009). Long-term treadmill exercise also had positive effects on cognitive function and synaptic plasticity in APP/PS1 double transgenic mice (Zhao et al., 2015). The above-mentioned studies prove that exercise training, especially voluntary exercise training, has a certain therapeutic effect on delaying the progression of AD. However, the relationship between exercise training and Psn is unclear.
Alzheimer’s disease and a high-fat diet
A high-fat diet is also one of the main causes of AD. High-fat diets have been shown to induce elevated insulin and blood glucose in the body and lead to the development of fatty liver (Oliveira et al., 2014). Research data suggest that high-fat diets have a detrimental effect on the brain, increasing the prevalence of AD and damaging the structure and function of the hippocampus, which impact consequently learning and memory (Stranahan et al., 2008). Obesity induced by a high-fat diet is one of the main risk factors for cognitive impairment in AD (Sah et al., 2017). The high-fat diet causes significant increases in plasma cholesterol (TC), triacylglycerol (TG), and low-density lipoprotein (LDL-C) (Park et al., 2018). Long-term high-fat diets can cause the body to produce more cholesterol and lead to other adverse effects on the body, increasing the risk of developing AD.
Studies reveal that a high-fat diet produces a series of negative effects on APP/PS1 double transgenic mice, who exhibited a phenotype of significantly impaired glucose tolerance (Hiltunen et al., 2012). Genetic and diet-induced insulin resistance affects the pre-pathological manifestations of AD in APP/PS1 double transgenic mice (Bruning et al., 2000). Both episodic and spatial memory were found to be impaired in mice fed on a high-fat diet for just one day (McLean et al., 2018). Further, APP/PS1 double transgenic mice fed on a high-fat diet exhibited increased body weight, decreased number of age spots in the hippocampus, and significant lipid droplets deposition in the liver (Guo et al., 2021). High-fat diets also promoted Aβ production (Perdoncin et al., 2021). Moreover, a high-fat diet resulted in memory and motor dysfunction and impaired socialization in APP/PS1 double transgenic mice (de Souza et al., 2019). Bad dietary habits can also cause many adverse effects. Short-term western diets are sufficient to induce an increase in oxidative stress in young APP/PS1 double transgenic mice (Studzinski et al., 2009). Feeding a high-fat diet to triad transgenic mice (including PSEN1 transgenic mice) impaired memory capacity, exacerbated memory deficits in AD mice, and impaired mitochondrial morphology (Martins et al., 2017). A high-fat diet leads to altered APP and PSEN1 protein levels and thus affects Aβ metabolism (Spagnuolo et al., 2020). Other studies have found that ethyl acetate (EAE) can prevent memory loss and reduce the progressive development of neurological disorders in AD patients (Jara-Moreno et al., 2018). A study found that high-fat diet-fed obese mice exhibit reversible impairment of hippocampal function (Hao et al., 2016). Even in the absence of obesity, an unbalanced diet with prolonged high-fat diets and continuous intake of excessive fat can cause cognitive impairment in mice (Cifre et al., 2018). High-fat diets can also have a range of negative effects on the heart; it is one of the major factors contributing to CVD (Stobdan et al., 2019). Obesity caused by a chronic high-fat diet also accelerates the aging of the body’s heart function and exercise capacity (Wen et al., 2018). Studies have shown that a high-fat diet can lead to the accumulation of heart lipids, decrease heart contractility, block the conduction, and cause serious structural lesions (Birse et al., 2010). A high-fat diet can also induce hereditary heart dysfunction, such as lipotoxic cardiomyopathy (Guida et al., 2019). It is thus clear that a high-fat diet can adversely affect the heart function of Drosophila and damage the integrity of heart structure and function. However, the relationship between a high-fat diet and heart Psn awaits further investigation.
In this review, we have systematically explored many articles on the interaction of the Psn gene and AD with aging, exercise training, and high-fat diets. Based on these, we show that the Psn gene is not only closely associated with aging but is also necessary for adult neuronal survival and normal heart function. Psn mutations can have many adverse effects on the body, and regular exercise training combined with good dietary habits can alleviate the adverse effects of Psn mutation-induced AD, such as heart dysfunction and muscle atrophy. However, the relationship between exercise training, a high-fat diet, and the Psn gene warrant further investigation. For example, further studies should focus on whether exercise training can improve cardiac aging caused by Psn overexpression or knockdown, a subject poorly examined. Therefore, the study of the relationship between exercise training, a high-fat diet, and Psn can provide a more comprehensive understanding of the prevention and treatment mechanism of aging.
Author Contributions:The authors confirm contribution to the paper as follows: research idea and study design: Y.H.G.; supervision: D.T.W., S.J.W., J.F.W. All authors reviewed the results and approved the final version of the manuscript.
Ethics Approval:Not applicable.
Funding Statement:This work was supported by the National Natural Science Foundation of China [Grant No. 32000832]; and the Shandong Province Natural Science Foundation [Grant No. ZR2020QC096].
Conflicts of Interest:The authors declare that they have no conflicts of interest to report regarding the present study.
References
Abraham D, Feher J, Scuderi GL, Szabo D, Dobolyi A, Cservenak M, Radak Z (2019). Exercise and probiotics attenuate the development of Alzheimer’s disease in transgenic mice: Role of microbiome. Experimental Gerontology 115: 122–131. DOI 10.1016/j.exger.2018.12.005. [Google Scholar] [CrossRef]
Adlard PA, Perreau VM, Pop V, Cotman CW (2005). Voluntary exercise decreases amyloid load in a transgenic model of Alzheimer’s disease. The Journal of Neuroscience 25: 4217–4221. DOI 10.1523/JNEUROSCI.0496-05.2005. [Google Scholar] [CrossRef]
Ankarcrona M, Hultenby K (2002). Presenilin-1 is located in rat mitochondria. Biochemical and Biophysical Research Communications 295: 766–770. DOI 10.1016/S0006-291X(02)00735-0. [Google Scholar] [CrossRef]
Arora S, Ligoxygakis P (2020). Beyond host defense: Deregulation of drosophilaimmunity and age-dependent neurodegeneration. Frontiers in Immunology 11: 1574. DOI 10.3389/fimmu.2020.01574. [Google Scholar] [CrossRef]
Birse RT, Choi J, Reardon K, Rodriguez J, Graham S, Diop S, Oldham S (2010). High-fat-diet-induced obesity and heart dysfunction are regulated by the TOR pathway in drosophila. Cell Metabolism 12: 533–544. DOI 10.1016/j.cmet.2010.09.014. [Google Scholar] [CrossRef]
Blanchard V, Czech C, Bonici B, Clavel N, Gohin M, Dalet K, Moussaoui S (1997). Immunohistochemical analysis of presenilin 2 expression in the mouse brain: Distribution pattern and co-localization with presenilin 1 protein. Brain Research 758: 209–217. DOI 10.1016/S0006-8993(97)00231-X. [Google Scholar] [CrossRef]
Bovo E, Nikolaienko R, Kahn D, Cho E, Robia LS, Zima VA (2021). Presenilin 1 is a direct regulator of the cardiac sarco/endoplasmic reticulum calcium pump. Cell Calcium 99: 102468. DOI 10.1016/j.ceca.2021.102468. [Google Scholar] [CrossRef]
Bowman GL, Quinn JF (2008). Alzheimer’s disease and the blood-brain barrier: Past, present and future. Aging Health 4: 47–55. DOI 10.2217/1745509X.4.1.47. [Google Scholar] [CrossRef]
Boyle PA, Buchman AS, Wilson RS, Leurgans SE, Bennett DA (2009). Association of muscle strength with the risk of Alzheimer disease and the rate of cognitive decline in community-dwelling older persons. Archives of Neurology 66: 1339–1344. DOI 10.1001/archneurol.2009.240. [Google Scholar] [CrossRef]
Bruni AC (1998). Cloning of a gene bearing missense mutations in early onset familial Alzheimer’s disease: A Calabrian study. Functional Neurology 13: 257–261. [Google Scholar]
Bruning JC, Gautam D, Burks DJ, Gillette J, Schubert M, Orban PC, Kahn CR (2000). Role of brain insulin receptor in control of body weight and reproduction. Science 289: 2122–2125. DOI 10.1126/science.289.5487.2122. [Google Scholar] [CrossRef]
Burns JM, Johnson DK, Watts A, Swerdlow RH, Brooks WM (2010). Reduced lean mass in early alzheimer disease and its association with brain atrophy. Archives of Neurology 67: 428–433. DOI 10.1001/archneurol.2010.38. [Google Scholar] [CrossRef]
Cannon L, Bodmer R (2016). Genetic manipulation of cardiac ageing. Journal of Physiology 594: 2075–2083. DOI 10.1113/JP270563. [Google Scholar] [CrossRef]
Chan KY, Wang W, Wu JJ, Liu L, Theodoratou E, Car J, Middleton L, Russ TC, Deary IJ, Campbell H, Wang W, Rudan I, Global Health Epidemiology Reference Group (GHERG) (2013). Epidemiology of Alzheimer's disease and other forms of dementia in China, 1990–2010: A systematic review and analysis. Lancet 381: 2016–2023. DOI 10.1016/S0140-6736(13)60221-4. [Google Scholar] [CrossRef]
Cifre M, Palou A, Oliver P (2018). Cognitive impairment in metabolically-obese, normal-weight rats: Identification of early biomarkers in peripheral blood mononuclear cells. Molecular Neurodegeneration 13: 14. DOI 10.1186/s13024-018-0246-8. [Google Scholar] [CrossRef]
Culvenor JG, Ilaya NT, Ryan MT, Canterford L, Hoke DE, Williamson NA, Evin G (2004). Characterization of presenilin complexes from mouse and human brain using Blue Native gel electrophoresis reveals high expression in embryonic brain and minimal change in complex mobility with pathogenic presenilin mutations. European Journal of Biochemistry 271: 375–385. DOI 10.1046/j.1432-1033.2003.03936.x. [Google Scholar] [CrossRef]
da Silva FC, Iop RDR, de Oliveira LC, Boll AM, Souza de Alvarenga JG et al. (2018). Effects of physical exercise programs on cognitive function in Parkinson’s disease patients: A systematic review of randomized controlled trials of the last 10 years. PLoS One 13: e0193113. DOI 10.1371/journal.pone.0193113. [Google Scholar] [CrossRef]
De la Rosa A, Olaso-Gonzalez G, Arc-Chagnaud C, Millan F, Salvador-Pascual A et al. (2020). Physical exercise in the prevention and treatment of Alzheimer’s disease. Journal of Sport and Health Science 9: 394–404. DOI 10.1016/j.jshs.2020.01.004. [Google Scholar] [CrossRef]
de Souza RM, de Souza L, Machado AE, de Bern Alves AC, Rodrigues FS, AguiarJr AS, Gasnhar Moreira EL (2019). Behavioural, metabolic and neurochemical effects of environmental enrichment in high-fat cholesterol-enriched diet-fed mice. Behavioural Brain Research 359: 648–656. DOI 10.1016/j.bbr.2018.09.022. [Google Scholar] [CrossRef]
Demontis F, Piccirillo R, Goldberg AL, Perrimon N (2013a). Mechanisms of skeletal muscle aging: insights from Drosophila and mammalian models. Disease Models & Mechanisms 6: 1339–1352. DOI 10.1242/dmm.012559. [Google Scholar] [CrossRef]
Demontis F, Piccirillo R, Goldberg AL, Perrimon N (2013b). The influence of skeletal muscle on systemic aging and lifespan. Aging Cell 12: 943–949. DOI 10.1111/acel.12126. [Google Scholar] [CrossRef]
Donoviel DB, Hadjantonakis AK, Ikeda M, Zheng H, Hyslop PS, Bernstein A (1999). Mice lacking both presenilin genes exhibit early embryonic patterning defects. Genes & Development 13: 2801–2810. DOI 10.1101/gad.13.21.2801. [Google Scholar] [CrossRef]
Falkenhain K, Ruiz-Uribe NE, Haft-Javaherian M, Ali M, Catchers S, Michelucci PE, Bracko O (2020). A pilot study investigating the effects of voluntary exercise on capillary stalling and cerebral blood flow in the APP/PS1 mouse model of Alzheimer’s disease. PLoS One 15: e0235691. DOI 10.1371/journal.pone.0235691. [Google Scholar] [CrossRef]
Feng R, Rampon C, Tang YP, Shrom D, Jin J, Kyin M, Tsien JZ (2001). Deficient neurogenesis in forebrain-specific presenilin-1 knockout mice is associated with reduced clearance of hippocampal memory traces. Neuron 32: 911–926. DOI 10.1016/S0896-6273(01)00523-2. [Google Scholar] [CrossRef]
Garcia-Mesa Y, Carlos Lopez-Ramos J, Gimenez-Llort L, Revilla S, Guerra R, Gruart A, Sanfeliu C (2011). Physical exercise protects against alzheimer’s disease in 3xTg-AD mice. Journal of Alzheimers Disease 24: 421–454. DOI 10.3233/JAD-2011-101635. [Google Scholar] [CrossRef]
Gaugler J, James B, Johnson T, Reimer J, Solis M, Weuve J, Hohman TJ (2022). 2022 Alzheimer’s disease facts and figures. Alzheimers & Dementia 18: 700–789. DOI 10.1002/alz.12638. [Google Scholar] [CrossRef]
Goldsmith T. C.(2014). Modern evolutionary mechanics theories and resolving the programmed/non-programmed aging controversy. Biochemistry. Biokhimiia 79: 1049–1055. DOI 10.1134/S000629791410006X. [Google Scholar] [CrossRef]
Grundman M (2005). Weight loss in the elderly may be a sign of impending dementia. Archives of Neurology 62: 20–22. DOI 10.1001/archneur.62.1.20. [Google Scholar] [CrossRef]
Guida MC, Birse RT, Dall’Agnese A, Toto PC, Diop SB, Mai A, Bodmer R (2019). Intergenerational inheritance of high fat diet-induced cardiac lipotoxicity in Drosophila. Nature Communications 10: 193. DOI 10.1038/s41467-018-08128-3. [Google Scholar] [CrossRef]
Guo Y, Ma X, Li P, Dong S, Huang X, Ren X, Yuan L (2021). High-fat diet induced discrepant peripheral and central nervous systems insulin resistance in APPswe/PS1dE9 and wild-type C57BL/6J mice. Aging-Us 13: 1236–1250. DOI 10.18632/aging.202262. [Google Scholar] [CrossRef]
Guo YQ, Livne-Bar I, Zhou LL, Boulianne GL (1999). Drosophila presenilin is required for neuronal differentiation and affects notch subcellular localization and signaling. Journal of Neuroscience 19: 8435–8442. DOI 10.1523/JNEUROSCI.19-19-08435.1999. [Google Scholar] [CrossRef]
Han J, Park H, Maharana C, Gwon AR, Park J, Baek SH, Bae HG, Cho Y, Kim HK, Sul JH, Lee J, Kim E, Kim J, Cho Y, Park S, Palomera LF, Arumugam TV, Mattson MP, JoDG(2021). Alzheimer's disease-causing presenilin-1 mutations have deleterious effects on mitochondrial function. Theranostics 11: 8855–8873. DOI 10.7150/thno.59776. [Google Scholar] [CrossRef]
Hao S, Dey A, Yu X, Stranahan AM (2016). Dietary obesity reversibly induces synaptic stripping by microglia and impairs hippocampal plasticity. Brain Behavior and Immunity 51: 230–239. DOI 10.1016/j.bbi.2015.08.023. [Google Scholar] [CrossRef]
Hartmann H, Busciglio J, Baumann KH, Staufenbiel M, Yankner BA (1997). Developmental regulation of presenilin-1 processing in the brain suggests a role in neuronal differentiation. The Journal of Biological Chemistry 272: 14505–14508. DOI 10.1074/jbc.272.23.14505. [Google Scholar] [CrossRef]
Hashimoto M, Kazui H, Matsumoto K, Nakano Y, Yasuda M, Mori E (2005). Does donepezil treatment slow the progression of hippocampal atrophy in patients with Alzheimer’s disease? The American Journal of Psychiatry 162: 676–682. DOI 10.1176/appi.ajp.162.4.676. [Google Scholar] [CrossRef]
Hiltunen M, Khandelwal VKM, Yaluri N, Tiilikainen T, Tusa M, Koivisto H, Tanila H (2012). Contribution of genetic and dietary insulin resistance to Alzheimer phenotype in APP/PS1 transgenic mice. Journal of Cellular and Molecular Medicine 16: 1206–1222. DOI 10.1111/j.1582-4934.2011.01384.x. [Google Scholar] [CrossRef]
Jara-Moreno D, Castro-Torres RD, Ettcheto M, Auladell C, Kogan MJ, Folch J, Camins A (2018). The ethyl acetate extract of leaves of Ugni molinae Turcz. Improves neuropathological hallmarks of alzheimer’s disease in female APPswe/PS1dE9 mice fed with a high fat diet. Journal of Alzheimers Disease 66: 1175–1191. DOI 10.3233/JAD-180174. [Google Scholar] [CrossRef]
Kang J, Shen J (2020). Cell-autonomous role of Presenilin in age-dependent survival of cortical interneurons. Molecular Neurodegeneration 15: 72. DOI 10.1186/s13024-020-00419-y. [Google Scholar] [CrossRef]
Kang J, Shin S, Perrimon N, Shen J (2017). An evolutionarily conserved role of presenilin in neuronal protection in the aging drosophila brain. Genetics 206: 1479–1493. DOI 10.1534/genetics.116.196881. [Google Scholar] [CrossRef]
Keller LJ, Sekula NM, Svirsky S, Maesako M, Zoltowska KM, Berezovska O (2020). Presenilin 1 increases association with synaptotagmin 1 during normal aging. Neurobiology of Aging 86: 156–161. DOI 10.1016/j.neurobiolaging.2019.10.006. [Google Scholar] [CrossRef]
Kim Ak, Kwon DW, Yeom E, Lee KP, Kwon KS, Yu K, Lee KS (2021). Lipophorin receptor 1 (LpR1) in Drosophila muscle influences life span by regulating mitochondrial aging. Biochemical and Biophysical Research Communications 568: 95–102. DOI 10.1016/j.bbrc.2021.06.080. [Google Scholar] [CrossRef]
Kim D, Cho J, Kang H (2019). Protective effect of exercise training against the progression of Alzheimer’s disease in 3xTg-AD mice. Behavioural Brain Research 374: 112105. DOI 10.1016/j.bbr.2019.112105. [Google Scholar] [CrossRef]
Kim WY, Shen J (2008). Presenilins are required for maintenance of neural stem cells in the developing brain. Molecular Neurodegeneration 3: 2. DOI 10.1186/1750-1326-3-2. [Google Scholar] [CrossRef]
Lai CH, Ho TJ, Kuo WW, Day CH, Pai PY, Chung LC, Huang CY (2014). Exercise training enhanced SIRT1 longevity signaling replaces the IGF1 survival pathway to attenuate aging-induced rat heart apoptosis. Age 36: 185. DOI 10.1007/s11357-014-9706-4. [Google Scholar] [CrossRef]
Lee MK, Slunt HH, Martin LJ, Thinakaran G, Kim G, Gandy SE, Sisodia SS (1996). Expression of presenilin 1 and 2 (PS1 and PS2) in human and murine tissues. Journal of Neuroscience 16: 7513–7525. DOI 10.1523/JNEUROSCI.16-23-07513.1996. [Google Scholar] [CrossRef]
Lehmann S, Chiesa R, Harris DA (1997). Evidence for a six-transmembrane domain structure of presenilin 1. Journal of Biological Chemistry 272: 12047–12051. DOI 10.1074/jbc.272.18.12047. [Google Scholar] [CrossRef]
Levy-Lahad E, Poorkaj P, Wang K, Fu YH, Oshima J, Mulligan J, Schellenberg GD (1996). Genomic structure and expression of STM2, the chromosome 1 familial Alzheimer disease gene. Genomics 34: 198–204. DOI 10.1006/geno.1996.0266. [Google Scholar] [CrossRef]
Li A, Xie Z, Dong Y, McKay KM, McKee ML, Tanzi RE (2007). Isolation and characterization of the Drosophila ubiquilin ortholog dUbqln: In vivo interaction with early-onset Alzheimer disease genes. Human Molecular Genetics 16: 2626–2639. DOI 10.1093/hmg/ddm219. [Google Scholar] [CrossRef]
Li A, Zhou C, Moore J, Zhang P, Tsai TH et al. (2011a). Changes in the expression of the alzheimer’s disease-associated presenilin gene in drosophila heart leads to cardiac dysfunction. Current Alzheimer Research 8: 313–322. DOI 10.2174/156720511795563746. [Google Scholar] [CrossRef]
Li B, Liang F, Ding X, Yan Q, Zhao Y, Zhang X, Bai Y, Huang T, Xu B (2019). Interval and continuous exercise overcome memory deficits related to beta-Amyloid accumulation through modulating mitochondrial dynamics. Behavioural Brain Research 376: 112171. DOI 10.1016/j.bbr.2019.112171. [Google Scholar] [CrossRef]
Li D, Parks SB, Kushner JD, Nauman D, Burgess D, Ludwigsen S, Hershberger RE et al. (2006). Mutations of presenilin genes in dilated cardiomyopathy and heart failure. American Journal of Human Genetics 79: 1030–1039. DOI 10.1086/509900. [Google Scholar] [CrossRef]
Li H, Chen Y, Zhou B, Peng Y, Sheng Y, Rao L (2011b). Polymorphisms of presenilin-1 gene associate with dilated cardiomyopathy susceptibility. Molecular and Cellular Biochemistry 358: 31–36. DOI 10.1007/s11010-011-0916-0. [Google Scholar] [CrossRef]
Lin TW, Shih YH, Chen SJ, Lien CH, Chang CY, Huang TY, Kuo YM et al. (2015). Running exercise delays neurodegeneration in amygdala and hippocampus of Alzheimer’s disease (APP/PS1) transgenic mice. Neurobiology of Learning and Memory 118: 189–197. DOI 10.1016/j.nlm.2014.12.005. [Google Scholar] [CrossRef]
Lin YS, Lin FY, Hsiao YH (2019). Myostatin is associated with cognitive decline in an animal model of alzheimer’s disease. Molecular Neurobiology 56: 1984–1991. DOI 10.1007/s12035-018-1201-y. [Google Scholar] [CrossRef]
Liu Hl, Zhao G, Zhang H, Shi LD (2013). Long-term treadmill exercise inhibits the progression of Alzheimer’s disease-like neuropathology in the hippocampus of APP/PS1 transgenic mice. Behavioural Brain Research 256: 261–272. DOI 10.1016/j.bbr.2013.08.008. [Google Scholar] [CrossRef]
Luchsinger JA, Gustafson DR (2009). Adiposity and Alzheimer’s disease. Current Opinion in Clinical Nutrition and Metabolic Care 12: 15–21. DOI 10.1097/MCO.0b013e32831c8c71. [Google Scholar] [CrossRef]
Marcon G, Di Fede G, Giaccone G, Rossi G, Giovagnoli AR, Maccagnano E, Tagliavini F (2009). A novel italian presenilin 2 gene mutation with prevalent behavioral phenotype. Journal of Alzheimers Disease 16: 509–511. DOI 10.3233/JAD-2009-0986. [Google Scholar] [CrossRef]
Martins IVA, Rivers-Auty J, Allan SM, Lawrence CB (2017). Mitochondrial abnormalities and synaptic loss underlie memory deficits seen in mouse models of obesity and alzheimer’s disease. Journal of Alzheimers Disease 55: 915–932. DOI 10.3233/JAD-160640. [Google Scholar] [CrossRef]
Mattson MP, Zhu H, Yu J, Kindy MS (2000). Presenilin-1 mutation increases neuronal vulnerability to focal ischemia in vivo and to hypoxia and glucose deprivation in cell culture: Involvement of perturbed calcium homeostasis. Journal of Neuroscience 20: 1358–1364. DOI 10.1523/JNEUROSCI.20-04-01358.2000. [Google Scholar] [CrossRef]
McBride SMJ, Choi CH, Schoenfeld BP, Bell AJ, Liebelt DA et al. (2010). Pharmacological and genetic reversal of age-dependent cognitive deficits attributable to decreased presenilin function. Journal of Neuroscience 30: 9510–9522. DOI 10.1523/JNEUROSCI.1017-10.2010. [Google Scholar] [CrossRef]
McLean FH, Grant C, Morris AC, Horgan GW, Polanski AJ et al. (2018). Rapid and reversible impairment of episodic memory by a high-fat diet in mice. Scientific Reports 8: 42. DOI 10.1038/s41598-018-30265-4. [Google Scholar] [CrossRef]
Nagakura A, Shitaka Y, Yarimizu J, Matsuoka N (2013). Characterization of cognitive deficits in a transgenic mouse model of Alzheimer’s disease and effects of donepezil and memantine. European Journal of Pharmacology 703: 53–61. DOI 10.1016/j.ejphar.2012.12.023. [Google Scholar] [CrossRef]
Nakajima M, Moriizumi E, Koseki H, Shirasawa T (2004). Presenilin 1 is essential for cardiac morphogenesis. Developmental Dynamics 230: 795–799. [Google Scholar]
Nichol KE, Poon WW, Parachikova AI, Cribbs DH, Glabe CG, Cotman CW (2008). Exercise alters the immune profile in Tg2576 Alzheimer mice toward a response coincident with improved cognitive performance and decreased amyloid. Journal of Neuroinflammation 5: 13. DOI 10.1186/1742-2094-5-13. [Google Scholar] [CrossRef]
Ocorr K, Reeves NL, Wessells RJ, Fink M, Chen HSV et al. (2007). KCNQ potassium channel mutations cause cardiac arrhythmias in Drosophila that mimic the effects of aging. PNAS 104: 3943–3948. DOI 10.1073/pnas.0609278104. [Google Scholar] [CrossRef]
Oliveira LSC, Santos DA, Barbosa-da-Silva S, Mandarim-de-Lacerda CA, Aguila MB (2014). The inflammatory profile and liver damage of a sucrose-rich diet in mice. Journal of Nutritional Biochemistry 25: 193–200. DOI 10.1016/j.jnutbio.2013.10.006. [Google Scholar] [CrossRef]
Park HS, Cho JH, Kim KW, Chung WS, Song MY (2018). Effects of Panax ginseng on obesity in animal models: A systematic review and meta-analysis. Evidence-Based Complementary and Alternative Medicine 2018: 2719794. DOI 10.1155/2018/2719794. [Google Scholar] [CrossRef]
Paternostro G, Vignola C, Bartsch DU, Omens JH, McCulloch AD, Reed JC (2001). Age-associated cardiac dysfunction in Drosophila melanogaster. Circulation Research 88: 1053–1058. DOI 10.1161/hh1001.090857. [Google Scholar] [CrossRef]
Perdoncin M, Konrad A, Wyner JR, Lohana S, Pillai SS, Pereira DG, Lakhani HV, Sodhi K (2021). A review of miRNAs as biomarkers and effect of dietary modulation in obesity associated cognitive decline and neurodegenerative disorders. Frontiers in Molecular Neuroscience 14: 14645. DOI 10.3389/fnmol.2021.756499. [Google Scholar] [CrossRef]
Raemaekers T, Esselens C, Annaert W (2005). Presenilin 1: More than just gamma-secretase. Biochemical Society Transactions 33: 559–562. DOI 10.1042/BST0330559. [Google Scholar] [CrossRef]
Rai M, Coleman Z, Curley M, Nityanandam A, Platt A et al. (2021). Proteasome stress in skeletal muscle mounts a long-range protective response that delays retinal and brain aging. Cell Metabolism 33: 1137–1154. DOI 10.1016/j.cmet.2021.03.005. [Google Scholar] [CrossRef]
Reynolds-Peterson C, Xu J, Zhao N, Cruse C, Yonel B et al. (2020). Heparan sulfate structure affects autophagy, lifespan, responses to oxidative stress, and cell degeneration in drosophila parkin mutants. G3-Genes Genomes Genetics 10: 129–141. DOI 10.1534/g3.119.400730. [Google Scholar] [CrossRef]
Ruiz JR, Sui X, Lobelo F, Morrow JRJr., Jackson AW, Sjostrom M, Blair SN (2008). Association between muscular strength and mortality in men: Prospective cohort study. BMJ 337: a439. DOI 10.1136/bmj.a439. [Google Scholar] [CrossRef]
Sah SK, Lee C, Jang JH, Park GH (2017). Effect of high-fat diet on cognitive impairment in triple-transgenic mice model of Alzheimers disease. Biochemical and Biophysical Research Communications 493: 731–736. DOI 10.1016/j.bbrc.2017.08.122. [Google Scholar] [CrossRef]
Small GW, Rabins PV, Barry PP, Buckholtz NS, DeKosky ST et al. (1997). Diagnosis and treatment of alzheimer disease and related disorders. Consensus statement of the american association for geriatric psychiatry, the alzheimer’s association, and the american geriatrics society. JAMA 278: 1363–1371. DOI 10.1001/jama.1997.03550160083043. [Google Scholar] [CrossRef]
Song H, Boo JH, Kim KH, Kim C, Kim YE et al. (2013). Critical role of presenilin-dependent gamma-secretase activity in DNA damage-induced promyelocytic leukemia protein expression and apoptosis. Cell Death and Differentiation 20: 639–648. DOI 10.1038/cdd.2012.162. [Google Scholar] [CrossRef]
Song XW, Yuan QN, Tang Y, Cao M, Shen YF et al. (2018). Conditionally targeted deletion of PSEN1 leads to diastolic heart dysfunction. Journal of Cellular Physiology 233: 1548–1557. DOI 10.1002/jcp.26057. [Google Scholar] [CrossRef]
Spagnuolo MS, Pallottini V, Mazzoli A, Iannotta L, Tonini C et al. (2020). A short-term western diet impairs cholesterol homeostasis and key players of beta amyloid metabolism in brain of middle aged rats. Molecular Nutrition & Food Research 64: 2000541. DOI 10.1002/mnfr.202000541. [Google Scholar] [CrossRef]
Stobdan T, Sahoo D, Azad P, Hartley I, Heinrichsen E, Zhou D, Haddad GG (2019). High fat diet induces sex-specific differential gene expression in Drosophila melanogaster. PLoS One 14: e0213474. DOI 10.1371/journal.pone.0213474. [Google Scholar] [CrossRef]
Stranahan AM, Norman ED, Lee K, Cutler RG, Telljohann RS, Egan JM, Mattson MP (2008). Diet-induced insulin resistance impairs hippocampal synaptic plasticity and cognition in middle-aged rats. Hippocampus 18: 1085–1088. DOI 10.1002/hipo.20470. [Google Scholar] [CrossRef]
Studzinski CM, Li F, Bruce-Keller AJ, Fernandez-Kim SO, Zhang L et al. (2009). Effects of short-term Western diet on cerebral oxidative stress and diabetes related factors in APP × PS1 knock-in mice. Journal of Neurochemistry 108: 860–866. DOI 10.1111/j.1471-4159.2008.05798.x. [Google Scholar] [CrossRef]
Sujkowski A, Gretzinger A, Soave N, Todi SV, Wessells R (2020). Alpha- and beta-adrenergic octopamine receptors in muscle and heart are required for Drosophila exercise adaptations. PLoS Genetics 16: e1008778. DOI 10.1371/journal.pgen.1008778. [Google Scholar] [CrossRef]
Takeda T, Asahi M, Yamaguchi O, Hikoso S, Nakayama H et al. (2005). Presenilin 2 regulates the systolic function of heart by modulating Ca2+ signaling. FASEB Journal 19: 2069–2071. DOI 10.1096/fj.05-3744fje. [Google Scholar] [CrossRef]
Tan YX, Liu GC, Chen HL, Lu MN, Chen B et al. (2020). Exercise-induced cognitive improvement is associated with sodium channel-mediated excitability in APP/PS1 mice. Neural Plasticity 2020: 1–18. DOI 10.1155/2020/9132720. [Google Scholar] [CrossRef]
Tapia-Rojas C, Aranguiz F, Varela-Nallar L, Inestrosa NC (2016). Voluntary running attenuates memory loss, decreases neuropathological changes and induces neurogenesis in a mouse model of alzheimer’s disease. Brain Pathology 26: 62–74. DOI 10.1111/bpa.12255. [Google Scholar] [CrossRef]
Teglas T, Abraham D, Jokai M, Kondo S, Mohammadi R et al. (2020). Exercise combined with a probiotics treatment alters the microbiome, but moderately affects signalling pathways in the liver of male APP/PS1 transgenic mice. Biogerontology 21: 807–815. DOI 10.1007/s10522-020-09895-7. [Google Scholar] [CrossRef]
Tublin JM, Adelstein JM, del Monte F, Combs CK, Wold LE (2019). Getting to the heart of alzheimer disease. Circulation Research 124: 142–149. DOI 10.1161/CIRCRESAHA.118.313563. [Google Scholar] [CrossRef]
Um HS, Kang EB, Leem YH, Cho IH, Yang CH et al. (2008). Exercise training acts as a therapeutic strategy for reduction of the pathogenic phenotypes for Alzheimer’s disease in an NSE/APPsw-transgenic model. International Journal of Molecular Medicine 22: 529–539. DOI 10.3892/ijmm_00000052. [Google Scholar]
Villegas A, Castaneda MM, Arias LF, Vieco B, Lopera F, Bedoya G (2007). Evaluation of amyloid-beta by the E280A mutation in presenilin gene. Biomedica 27: 372–384. DOI 10.7705/biomedica.v27i3.200. [Google Scholar] [CrossRef]
Wen DT, Zheng L, Li JX, Lu K, Hou WQ (2019). The activation of cardiac dSir2-related pathways mediates physical exercise resistance to heart aging in old Drosophila. Aging-Us 11: 7274–7293. DOI 10.18632/aging.102261. [Google Scholar] [CrossRef]
Wen DT, Zheng L, Lu K, Hou WQ (2021). Physical exercise prevents age-related heart dysfunction induced by high-salt intake and heart salt-specific overexpression in Drosophila. Aging-Us 13: 19542–19560. DOI 10.18632/aging.203364. [Google Scholar] [CrossRef]
Wen DT, Zheng L, Yang F, Li HZ, Hou WQ (2018). Endurance exercise prevents high-fat-diet induced heart and mobility premature aging and dsir2 expression decline in aging Drosophila. Oncotarget 9: 7298–7311. DOI 10.18632/oncotarget.23292. [Google Scholar] [CrossRef]
Wolfe RR (2006). The underappreciated role of muscle in health and disease. The American Journal of Clinical Nutrition 84: 475–482. DOI 10.1093/ajcn/84.3.475. [Google Scholar] [CrossRef]
Xia J, Li B, Yin L, Zhao N, Yan Q, Xu B (2019). Treadmill exercise decreases beta-amyloid burden in APP/PS1 transgenic mice involving regulation of the unfolded protein response. Neuroscience Letters 703: 125–131. DOI 10.1016/j.neulet.2019.03.035. [Google Scholar] [CrossRef]
Yang M, Li C, Zhang Y, Ren J (2020). Interrelationship between Alzheimer’s disease and cardiac dysfunction: the brain-heart continuum? Acta Biochimica et Biophysica Sinica 52: 1–8. DOI 10.1093/abbs/gmz115. [Google Scholar] [CrossRef]
Ye Y, Fortini ME (1999). Apoptotic activities of wild-type and Alzheimer’s disease-related mutant presenilins in Drosophila melanogaster. The Journal of Cell Biology 146: 1351–1364. DOI 10.1083/jcb.146.6.1351. [Google Scholar] [CrossRef]
Young J, Angevaren M, Rusted J, Tabet N(2015). Aerobic exercise to improve cognitive function in older people without known cognitive impairment. The Cochrane Database of Systematic Reviews 4: CD005381. DOI 10.7150/thno.59776. [Google Scholar] [CrossRef]
Yuede CM, Zimmerman SD, Dong H, Kling MJ, Bero AW et al. (2009). Effects of voluntary and forced exercise on plaque deposition, hippocampal volume, and behavior in the Tg2576 mouse model of Alzheimer’s disease. Neurobiology of Disease 35: 426–432. DOI 10.1016/j.nbd.2009.06.002. [Google Scholar] [CrossRef]
Zeng B, Zhao G, Liu HL (2020). The differential effect of treadmill exercise intensity on hippocampal soluble Aβ and lipid metabolism in APP/PS1 mice. Neuroscience 430: 73–81. DOI 10.1016/j.neuroscience.2020.01.005. [Google Scholar] [CrossRef]
Zhang L, Chao FL, Luo YM, Xiao Q, Jiang L et al. (2017). Exercise prevents cognitive function decline and demyelination in the white matter of APP/PS1 transgenic AD mice. Current Alzheimer Research 14: 645–655. DOI 10.2174/1567205014666161213121353. [Google Scholar] [CrossRef]
Zhang XL, Zhao N, Xu B, Chen XH, Li TJ (2019). Treadmill exercise inhibits amyloid-beta generation in the hippocampus of APP/PS1 transgenic mice by reducing cholesterol-mediated lipid raft formation. Neuroreport 30: 498–503. DOI 10.1097/WNR.0000000000001230. [Google Scholar] [CrossRef]
Zhang Z, Wu H, Huang H (2016). Epicatechin plus treadmill exercise are neuroprotective against moderate-stage amyloid precursor protein/presenilin 1 mice. Pharmacognosy Magazine 12: S139–S146. DOI 10.4103/0973-1296.182174. [Google Scholar] [CrossRef]
Zhao G, Liu HL, Zhang H, Tong XJ (2015). Treadmill exercise enhances synaptic plasticity, but does not alter beta-amyloid deposition in hippocampi of aged APP/PS1 transgenic mice. Neuroscience 298: 357–366. DOI 10.1016/j.neuroscience.2015.04.038. [Google Scholar] [CrossRef]
Zhao N, Yan QW, Xia J, Zhang XL, Li BX, Yin LY, Xu B (2020). Treadmill exercise attenuates Aβ-induced mitochondrial dysfunction and enhances mitophagy activity in APP/PS1 transgenic mice. Neurochemical Research 45: 1202–1214. DOI 10.1007/s11064-020-03003-4. [Google Scholar] [CrossRef]
Zheng ZZ, Chao ML, Fan ZB, Zhao YJ, Song HS (2015). Molecular cloning and characterization of presenilin gene in Bombyx mori. Molecular Medicine Reports 12: 5508–5516. DOI 10.3892/mmr.2015.4019. [Google Scholar] [CrossRef]
Zhuang L, Peng F, Huang Y, Li W, Huang J et al. (2020). CHIP modulates APP-induced autophagy-dependent pathological symptoms in Drosophila. Aging Cell 19: e13070. DOI 10.1111/acel.13070. [Google Scholar] [CrossRef]
Cite This Article
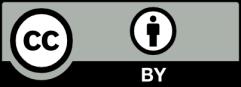
This work is licensed under a Creative Commons Attribution 4.0 International License , which permits unrestricted use, distribution, and reproduction in any medium, provided the original work is properly cited.