Open Access
ARTICLE
Magnesium Demethylcantharidate induces apoptosis in hepatocellular carcinoma cells via ER stress
1 Guizhou Provincial College-Based Key Lab for Tumor Prevention and Treatment with Distinctive Medicines, Zunyi Medical University, Zunyi, 563000, China
2 College of Basic Medicine, Zunyi Medical University, Zunyi, 563000, China
3 Scientific Research Center, The First People’s Hospital of Zunyi (The Third Affiliated Hospital of Zunyi Medical University), Zunyi, 563000, China
4 School of Forensic Medicine, Zunyi Medical University, Zunyi, 563000, China
* Corresponding Authors: RONG YAN. Email: ; Yun Liu. Email:
# These authors contributed equally
(This article belongs to the Special Issue: Herbal Active Ingredients: Potential for the Prevention and Treatment of Cancer)
BIOCELL 2022, 46(12), 2595-2600. https://doi.org/10.32604/biocell.2022.025468
Received 14 May 2022; Accepted 22 July 2022; Issue published 10 August 2022
Abstract
Cantharidin (CTD) is a bioactive ingredient isolated from Cantharis vesicatoria (blister beetles), which has potential therapeutic value as an anticancer agent. Magnesium Demethylcantharidate (MDC) is a recently developed derivative of Cantharidin (CTD), and previous studies have illustrated its excellent anticancer activity on HCC cells. However, the effect and mechanism of MDC remains unclear and need to be further studied. In particular, whether MDC can cause ER stress in HCC is still unknown. In this study, we demonstrated that endoplasmic reticulum stress (ERS)-related proteins were changed in SMMC-7721 and Bel-7402 cells after being exposed to MDC. Moreover, we found that MDC could significantly inhibit the growth of xenograft tumor in nude mice. In summary, we confirmed that MDC could induce ERS in HCC cells and thus induce apoptosis.Keywords
Being ranked as the seventh most common cancer and the third lethal cause in cancer all over the world according to GLOBOCAN 2020, there were approximately 905,677 new liver cancer cases and 830,180 deaths annually (Sung et al., 2021). The main type of primary liver cancer was HCC, which comprises more than 80% of the cases (Yang et al., 2019). Chronic Hepatitis B Virus (HBV), Hepatitis C Virus (HCV), alcohol abuse, diabetes and exposure to aflatoxin B1 are risk factors for HCC (Kulik and El-Serag, 2019). Besides, Surgical resection, liver transplantation, radio-embolization, radiofrequency ablation, chemotherapy, targeted therapy and immunotherapy are commonly used for HCC patients (Lee et al., 2020). However, the 5-year survival rate of HCC is 18% globally and even as low as 12% in China (Craig et al., 2020).
Endoplasmic reticulum (ER) is responsible for the synthesis, folding, processing, transportation of protein, the storage of Ca2+ (Oakes, 2020), and participate in lipid metabolism. The disorder of Ca2+ and accumulation of unfolded proteins in ER lumen, which is called ER stress (ERS), can be caused by reactive oxygen species (ROS), hypoxia and nutrient deprivation, as they could result in the disorder of cellular redox regulation (Kim and Kim, 2018). Unfolded protein response (UPR), mediated by inosito-requiring enzyme 1 (IRE1), protein kinase RNA-like ER kinase (PERK) and activating transcription factor 6 (ATF6), can alleviate ERS and maintain intracellular homeostasis (Marciniak, 2019). In addition, excessive and persistent ERS will lead to the binding of glucose-regulated protein 78 (GRP78) or binding protein (BIP) to the unfolded protein and promote the activation of IRE1, PERK and ATF6 pathways, thereby activating the pro-apoptotic pathway to induce cancer cell death (Chen and Cubillos-Ruiz, 2021; Eugene et al., 2020; Zachariah et al., 2021). Recently, a large number of studies have confirmed the role of ERS in nonalcoholic steatohepatitis, chronic liver disease and HCC (Hernández-Alvarez et al., 2019; Lebeaupin et al., 2018; Liu et al., 2019; Wu et al., 2021; Xia et al., 2020). Therefore, it is necessary to find effective ERS inducers for the treatment of HCC.
In recent years, an increasing number of researchers have realized that some natural compounds can kill cancer cells, and exploring the molecular mechanisms would also be interesting by which we can find out how these compounds work against tumors. Cantharis vesicatoria (blister beetles) has been used as a traditional Chinese folk medicine for more than 2000 years (Puerto Galvis et al., 2013). Cantharidin (CTD) is an anticancer active ingredient isolated from Cantharis vesicatoria. Current studies have reported that the anticancer mechanisms of cantharidin and its derivatives include inhibition of invasion and metastasis of tumor cells (Hsia et al., 2016), induction of apoptosis (Wang et al., 2018), regulation of cell cycle (Ye et al., 2017), induction of autophagy (Sun et al., 2017). Magnesium Demethylcantharidate (MDC) is a derivative of cantharidin with anti-HCC activity (Liu et al., 2021). Because there are two methyl groups less than cantharidin, the toxicity of MDC is greatly reduced, and its LD50 is much higher than cantharidin (Li and Casida, 1992; Liu et al., 2021). But the mechanism of MDC remains to be further studied. In particular, whether MDC can cause ER stress of HCC were still unknown. In this study, we elucidated the anticancer mechanism of MDC in two HCC cell lines through ERS signaling pathway. Our study suggests that MDC is a potential drug for the treatment of HCC.
Magnesium Demethylcantharidate (MDC) was synthesized according to the Chinese patent (ZL201410163711.0), and the purity was over 95%. The HCC cell lines SMMC-7721 and Bel-7402 were obtained from Genechem Co., Ltd. (Shanghai, China). The cell lines were cultured in RPMI-1640 medium (Hyclone, Logan, UT, USA) supplemented with 10% fetal bovine serum (Biological Industries, Beit Haemek Ltd., Israel) and 1% penicillin/streptomycin (Solarbio, Beijing, China) and were maintained at 37°C in a humidified incubator containing 5% CO2.
SRB colorimetry was performed to test cell viability. The cells (8 × 103) at logarithmic growth stage were seeded into 96-well plates. After culturing for 24 h, different concentrations of MDC (0, 6.25, 12.5, 25, 50 and 100 μM) were added for further culture for 24 or 48 h. Staining with SRB (Sigma-Aldrich, Saint Louis, MO, USA), then the SpectraMax i3X tablet reader (Molecular Devices, Silicon Valley, CA, USA) was used to measure the absorbance at 530 nm. The cell viability measurements were performed in three independent replicates.
Cells (500 cells/well) were seeded into 6-well plates and cultured for 24 h. Then, SMMC-7721 and Bel-7402 cells were treated with MDC (0, 9, 18 or 36 µM), respectively, and cultured for another 14 days without drugs. The clones were counted after staining with Giemsa solution (Solarbio, Beijing, China).
The cells in 96-well were treated with MDC (0, 9, 18 or 36 µM) for 24 h, fixed with pre-cooled 4% paraformaldehyde for 15 min, then added 80 μL Hoechst33342 (Beyotime, Shanghai, China) staining reagent to each well for 25 min, and observed under fluorescence microscope (Olympus, Tokyo, Japan).
Cells were harvested after treatment with various concentrations of MDC (0, 9, 18 or 36 µM) for 24 h. The BCA (Solarbio, Beijing, China) method detected the protein concentration of cells and the dissected tumors, lysed by RIPA lysis buffer (Solarbio, Beijing, China). 50 μg of proteins per well was sequentially electrophoretic, electrotransfer, blocked, incubated primary antibody, and incubated secondary antibody. Protein bands were finally incubated using Immobilon Western Chemiluminescent HRP Substrate (Millipore, MA, USA) and then detected by the BIO-RAD ChemiDoc Imaging System (Hercules, CA, USA).
4-week-old male BALB/c nude mice (Beijing HFK Bioscience Co., Ltd., Beijing, China) were maintained in specific pathogen free (SPF) condition. After one week of adaptation, SMMC-7721 cells (2 × 106) were inoculated subcutaneously on the right posterior side of the nude mouse’s back. Once the mean tumor volume reached 70 mm3, the drug treatment group (n = 6) was intraperitoneally injected with MDC (4.3 mg/kg) every other day, and the vehicle group (n = 6) was intraperitoneally injected with normal saline. The nude mice were weighed and tumor volume was measured.
All experiments were independently repeated for thrice. All data were expressed as means ± SD. GraphPad Prism 7.0 (Graphpad Software, San Diego, CA, USA) was used for statistical analysis of data. One-way ANOVA and Student’s t-test were used for data difference analysis. A p value < 0.05 was indicated statistically significant.
MDC effectively inhibits the proliferation of HCC cells
SRB assay was used to detect the effect of MDC on cell viability of SMMC-7721 and Bel-7402 cells after 24 and 48 h treatment. In this study, MDC significantly reduced SMMC-7721 and Bel-7402 cells viability in a dose- and time-dependent manner (Figs. 1B and 1C). The colony formation showed that the colony number of SMMC-7721 and Bel-7402 cells decreased significantly in a dose-dependent manner (Figs. 1D–1F). These results proved that MDC could inhibit the proliferation of the two HCC cell lines.
Figure 1: MDC effectively suppressed the proliferation of HCC cells. (A) The chemical structure of MDC. After treatment with various doses of MDC for 24 or 48 h, the SRB colorimetry was used to detect cell viability of SMMC-7721 and Bel-7402 cells (B and C). The colony formation assay was performed to detect proliferation, after treating SMMC-7721 and Bel-7402 cells with 0, 9, 18 or 36 µM of MDC for 24 h (D–F). The data of three independent replicates were expressed as the means ± SD ( *p < 0.05 vs. control).
MDC induced apoptosis in HCC cells
The results of Hoechst staining showed that the chromatin of condensation, polynucleosomal fragmentation and nuclear contraction increased with the increase of MDC (Fig. 2A). Furthermore, Western blot was used to detect the expression of apoptosis-related proteins after MDC treatment. Western blot showed that cleaved caspase-3, cleaved caspase-9, and Bax/Bcl-2 expressions were increased in SMMC-7721 and Bel-7402 cells in a dose-dependent manner after MDC treatment (Fig. 2B). According to these results, it indicated that MDC treatment could induce apoptosis of HCC Cells.
Figure 2: MDC induced apoptosis in HCC cells. (A) Hoechst staining showed that the chromatin of condensation, polynucleosomal fragmentation and nuclear contraction, after treating SMMC-7721 and Bel-7402 cells with 0, 9, 18 or 36 µM of MDC for 24 h. Hoechst staining ×200, bar: 50 μm. (B) Western blotting was used to analyze apoptotic proteins Bax, Bcl-2, cleaved caspase-3, and cleaved caspase-9.
As shown in the Fig. 3, western blot analysis revealed that ERS occurred when SMMC-7721 and Bel-7402 cells were exposed to MDC, resulting in high expression of ERS-related proteins p-IRE1, GRP78/BiP, XBP1s, Caspase 12 and CHOP. It suggested that ERS induced by MDC must be an important feature of HCC apoptosis.
Figure 3: MDC induced ERS in HCC cells. Western blotting was used to analyze ERS related proteins, including p-IRE1, GRP78/BiP, XBP1s, Caspase 12 and CHOP.
MDC significantly inhibited the growth of xenograft tumors in nude mice
SMMC-7721 cells were inoculated subcutaneously into the dorsal side of the right hind limb of nude mice to construct allograft tumor model. After the average tumor volume reached 70 mm3, the nude mice were randomly assigned to control group and drug treatment group. The drug treatment group was intraperitoneally injected with MDC (4.3 mg/kg) every other day for 20 days. Compared with the control group, the weight of the nude mice treated with MDC did not change significantly, but the mass and volume of the allograft tumor were significantly reduced (Figs. 4A–4D). These data suggested that MDC could inhibit the growth of HCC in vivo.
Figure 4: MDC significantly inhibited the growth of xenograft tumors in nude mice. (A) After treatment with MDC for 20 days, nude mice were sacrificed and tumor masses were exfoliated. (B) The exfoliated tumor mass of the two groups, six nude mice in each group. (C and D) Tumor volumes and body weight of nude mice were measured every other day. Data are represented as means ± SD. *p < 0.05 vs. control.
HCC is a serious threat to human health due to its high incidence and mortality (He et al., 2021; Piñero et al., 2020). In recent decades, many researchers found that certain natural products can kill HCC cells, and it would also be interesting to explore the anti-tumor molecular mechanisms. Cantharidin is one of natural products that can significantly inhibit the proliferation of HCC cells both in vitro and in vivo (Wang et al., 2000; Zhu et al., 2020). MDC is a novel agent derived from cantharidin, which can inhibit the invasion and metastasis of HCC cells by activating transcription factor FOXO1 (Liu et al., 2021). However, the mechanism of MDC needs further investigation. In particular, whether MDC can cause ER stress of HCC remains unknown.
It is known that apoptosis includes intrinsic and extrinsic pathways. The intrinsic pathway can be triggered by ERS, excess reactive oxygen species (Kesavardhana et al., 2020). ERS is the adaptive response of cells to external adverse stimuli. In the early stage, ERS can inhibit cell apoptosis, which is beneficial to cell growth. However, when ERS is too intense and persistent, it can induce apoptosis and therefore promote cell death (Fernández et al., 2015). In this study, we observed the elevation of Bax/Bcl-2 levels, and the caspase-9 and caspase-3 were cleaved after HCC cells were treated with MDC (Fig. 2B), which indicated that intrinsic apoptosis occurred in HCC cells. The increased expression of BIP was observed through western blot, leading to the phosphorylation of IRE1 and further proved that MDC induced apoptosis is generated through ERS signaling pathway (Fig. 3). Phosphorylated IRE1 can promote the synthesis of spliced X-box binding protein1 (XBP1s), and activate ASK1/JNK signal to activate C/EBP homologous protein (CHOP), Caspase12 and Bax (Liu et al., 2020). When CHOP and Caspase12 elevated, the expression of Bcl-2 is inhibited, then, cleaved Caspase-9 was released, and cleaved Caspase-3 was further released, inducing apoptosis (Meng et al., 2019).
Overall, we demonstrated that MDC could induce apoptosis of HCC cells through the ERS pathway. This finding suggest that MDC may be a potential anti-liver cancer drug.
Availability of Data and Materials: The datasets generated during and/or analysed during the current study are available from the corresponding author on reasonable request.
Author Contributions:Xinting Zhu, Yun Liu, Rong Yan and Xiaofei Li conceptualized and designed the study; Xinting Zhu, Meng Ye, Kelan Fang, Fang Liu, Jing Hui and Meichen Liu performed the experiments and the data analysis. Xinting Zhu and Meng Ye wrote the manuscript. All authors have read and approved the final manuscripts.
Ethics Approval: This study was approved by the Institutional Animal Ethics and Use Committee of Zunyi Medical University at 2016-3-15, and the ethical approval code was LS(2016)-2-065.
Funding Statement: This work was supported by Natural Science Foundation of China (81660611); Guizhou Provincial Science & Technology Program (ZK[2022]615); the Science and Technology Project of Guizhou Provincial Health Commission (gzwkj2021-59); the Science & Technology Plan of Zunyi (ZSKHZC-HZ[2020]98); the Xin miao Foundation of Zunyi Medical University ([2017]5733-055); Innovation Talent Team of Guizhou Science and Technology Department (QKHPTRC[2020] 5007); Science and Technology Department of Zunyi City of Guizhou province of China ([2020]7); and Innovation Talent Team of Zunyi (ZSKRC[2019]1).
Conflicts of Interest: The authors declare that they have no conflicts of interest to report regarding the present study.
References
Chen X, Cubillos-Ruiz JR (2021). Endoplasmic reticulum stress signals in the tumour and its microenvironment. Nature Reviews Cancer 21: 71–88. DOI 10.1038/s41568-020-00312-2. [Google Scholar] [CrossRef]
Craig AJ, von Felden J, Garcia-Lezana T, Sarcognato S, Villanueva A (2020). Tumour evolution in hepatocellular carcinoma. Nature Reviews Gastroenterology & Hepatology 17: 139–152. DOI 10.1038/s41575-019-0229-4. [Google Scholar] [CrossRef]
Eugene SP, Reddy VS, Trinath J (2020). Endoplasmic reticulum stress and intestinal inflammation: A perilous union. Frontiers in Immunology 11: 543022. DOI 10.3389/fimmu.2020.543022. [Google Scholar] [CrossRef]
Fernández A, Ordóñez R, Reiter RJ, González-Gallego J, Mauriz JL (2015). Melatonin and endoplasmic reticulum stress: Relation to autophagy and apoptosis. Journal of Pineal Research 59: 292–307. DOI 10.1111/jpi.12264. [Google Scholar] [CrossRef]
He K, Liu X, Cheng S, Zhou P (2021). Zingiberensis newsaponin inhibits the malignant progression of hepatocellular carcinoma via suppressing autophagy moderated by the AKR1C1-mediated JAK2/STAT3 pathway. Evidence-Based Complementary and Alternative Medicine 2021: 4055209–4055211. DOI 10.1155/2021/4055209. [Google Scholar] [CrossRef]
Hernández-Alvarez MI, Sebastián D, Vives S, Ivanova S, Bartoccioni P et al. (2019). Deficient endoplasmic reticulum-mitochondrial phosphatidylserine transfer causes liver disease. Cell 177: 881–895.e817. DOI 10.1016/j.cell.2019.04.010. [Google Scholar] [CrossRef]
Hsia TC, Yu CC, Hsiao YT, Wu SH, Bau DT, Lu HF, Huang YP, Lin JG, Chang SJ, Chung JG (2016). Cantharidin impairs cell migration and invasion of human lung cancer NCI-H460 cells via UPA and MAPK signaling pathways. Anticancer Research 36: 5989–5997. DOI 10.21873/anticanres.11187. [Google Scholar] [CrossRef]
Kesavardhana S, Malireddi RKS, Kanneganti TD (2020). Caspases in cell death, inflammation, and pyroptosis. Annual Review of Immunology 38: 567–595. DOI 10.1146/annurev-immunol-073119-095439. [Google Scholar] [CrossRef]
Kim C, Kim B (2018). Anti-cancer natural products and their bioactive compounds inducing ER stress-mediated apoptosis: A review. Nutrients 10: 1021. DOI 10.3390/nu10081021. [Google Scholar] [CrossRef]
Kulik L, El-Serag HB (2019). Epidemiology and management of hepatocellular carcinoma. Gastroenterology 156: 477–491.e471. DOI 10.1053/j.gastro.2018.08.065. [Google Scholar] [CrossRef]
Lebeaupin C, Vallée D, Hazari Y, Hetz C, Chevet E, Bailly-Maitre B (2018). Endoplasmic reticulum stress signalling and the pathogenesis of non-alcoholic fatty liver disease. Journal of Hepatology 69: 927–947. DOI 10.1016/j.jhep.2018.06.008. [Google Scholar] [CrossRef]
Lee YH, Tai D, Yip C, Choo SP, Chew V (2020). Combinational immunotherapy for hepatocellular carcinoma: Radiotherapy, immune checkpoint blockade and beyond. Frontiers in Immunology 11: 568759. DOI 10.3389/fimmu.2020.568759. [Google Scholar] [CrossRef]
Li YM, Casida JE (1992). Cantharidin-binding protein: Identification as protein phosphatase 2A. Proceedings of The National Academy of Sciences 89: 11867–11870. DOI 10.1073/pnas.89.24.11867. [Google Scholar] [CrossRef]
Liu F, Zhu XT, Li Y, Wang CJ, Fu JL et al. (2021). Magnesium demethylcantharidate inhibits hepatocellular carcinoma cell invasion and metastasis via activation transcription factor FOXO1. European Journal of Pharmacology 911: 174558. DOI 10.1016/j.ejphar.2021.174558. [Google Scholar] [CrossRef]
Liu J, Fan L, Yu H, Zhang J, He Y et al. (2019). Endoplasmic reticulum stress causes liver cancer cells to release exosomal miR-23a-3p and up-regulate programmed death ligand 1 expression in macrophages. Hepatology 70: 241–258. DOI 10.1002/hep.30607. [Google Scholar] [CrossRef]
Liu Q, Körner H, Wu H, Wei W (2020). Endoplasmic reticulum stress in autoimmune diseases. Immunobiology 225: 151881. DOI 10.1016/j.imbio.2019.11.016. [Google Scholar] [CrossRef]
Marciniak SJ (2019). Endoplasmic reticulum stress: A key player in human disease. The FEBS Journal 286: 228–231. DOI 10.1111/febs.14740. [Google Scholar] [CrossRef]
Meng X, Dong H, Pan Y, Ma L, Liu C, Man S, Gao W (2019). Diosgenyl saponin inducing endoplasmic reticulum stress and mitochondria-mediated apoptotic pathways in liver cancer cells. Journal of Agricultural and Food Chemistry 67: 11428–11435. DOI 10.1021/acs.jafc.9b05131. [Google Scholar] [CrossRef]
Oakes SA (2020). Endoplasmic reticulum stress signaling in cancer cells. The American Journal of Pathology 190: 934–946. DOI 10.1016/j.ajpath.2020.01.010. [Google Scholar] [CrossRef]
Piñero F, Dirchwolf M, Pessôa MG (2020). Biomarkers in hepatocellular carcinoma: Diagnosis, prognosis and treatment response assessment. Cells 9: 1370. DOI 10.3390/cells9061370. [Google Scholar] [CrossRef]
Puerto Galvis CE, Vargas Méndez LY, Kouznetsov VV (2013). Cantharidin-based small molecules as potential therapeutic agents. Chemical Biology & Drug Design 82: 477–499. DOI 10.1111/cbdd.12180. [Google Scholar] [CrossRef]
Sun CY, Zhu Y, Li XF, Tang LP, Su ZQ, Wang XQ, Li CY, Yang HM, Zheng GJ, Feng B (2017). Norcantharidin alone or in combination with crizotinib induces autophagic cell death in hepatocellular carcinoma by repressing c-Met-mTOR signaling. Oncotarget 8: 114945–114955. DOI 10.18632/oncotarget.22935. [Google Scholar] [CrossRef]
Sung H, Ferlay J, Siegel RL, Laversanne M, Soerjomataram I, Jemal A, Bray F (2021). Global cancer statistics 2020: GLOBOCAN estimates of incidence and mortality worldwide for 36 cancers in 185 countries. CA: A Cancer Journal for Clinicians 71: 209–249. DOI 10.3322/caac.21660. [Google Scholar] [CrossRef]
Wang CC, Wu CH, Hsieh KJ, Yen KY, Yang LL (2000). Cytotoxic effects of cantharidin on the growth of normal and carcinoma cells. Toxicology 147: 77–87. DOI 10.1016/S0300-483X(00)00185-2. [Google Scholar] [CrossRef]
Wang SC, Chow JM, Chien MH, Lin CW, Chen HY, Hsiao PC, Yang SF (2018). Cantharidic acid induces apoptosis of human leukemic HL-60 cells via c-Jun N-terminal kinase-regulated caspase-8/-9/-3 activation pathway. Environmental Toxicology 33: 514–522. DOI 10.1002/tox.22537. [Google Scholar] [CrossRef]
Wu J, Qiao S, Xiang Y, Cui M, Yao X, Lin R, Zhang X (2021). Endoplasmic reticulum stress: Multiple regulatory roles in hepatocellular carcinoma. Biomedicine & Pharmacotherapy = Biomedecine & Pharmacotherapie 142: 112005. DOI 10.1016/j.biopha.2021.112005. [Google Scholar] [CrossRef]
Xia SW, Wang ZM, Sun SM, Su Y, Li ZH et al. (2020). Endoplasmic reticulum stress and protein degradation in chronic liver disease. Pharmacological Research 161: 105218. DOI 10.1016/j.phrs.2020.105218. [Google Scholar] [CrossRef]
Yang JD, Hainaut P, Gores GJ, Amadou A, Plymoth A, Roberts LR (2019). A global view of hepatocellular carcinoma: Trends, risk, prevention and management. Nature Reviews Gastroenterology & Hepatology 16: 589–604. DOI 10.1038/s41575-019-0186-y. [Google Scholar] [CrossRef]
Ye K, Wei Q, Gong Z, Huang Y, Liu H, Li Y, Peng X (2017). Effect of norcantharidin on the proliferation, apoptosis, and cell cycle of human mesangial cells. Renal Failure 39: 458–464. DOI 10.1080/0886022X.2017.1308257. [Google Scholar] [CrossRef]
Zachariah M, Maamoun H, Milano L, Rayman MP, Meira LB, Agouni A (2021). Endoplasmic reticulum stress and oxidative stress drive endothelial dysfunction induced by high selenium. Journal of Cellular Physiology 236: 4348–4359. DOI 10.1002/jcp.30175. [Google Scholar] [CrossRef]
Zhu M, Shi X, Gong Z, Su Q, Yu R, Wang B, Yang T, Dai B, Zhan Y, Zhang D (2020). Cantharidin treatment inhibits hepatocellular carcinoma development by regulating the JAK2/STAT3 and PI3K/Akt pathways in an EphB4-dependent manner. Pharmacological Research 158: 104868. DOI 10.1016/j.phrs.2020.104868. [Google Scholar] [CrossRef]
Cite This Article
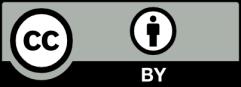
This work is licensed under a Creative Commons Attribution 4.0 International License , which permits unrestricted use, distribution, and reproduction in any medium, provided the original work is properly cited.