Open Access
REVIEW
Advances in the studies on symbiotic arbuscular mycorrhizal fungi of traditional Chinese medicinal plants
1 College of Life Sciences, Guangxi Normal University, Guilin, 541006, China
2 Guangxi Institute of Botany, Guangxi Zhuangzu Autonomous Region and Chinese Academy of Sciences, Guilin, 541006, China
* Corresponding Author: ZHONGFENG ZHANG. Email:
(This article belongs to the Special Issue: Mycorrhizal Fungal Roles in Stress Tolerance of Plants)
BIOCELL 2022, 46(12), 2559-2573. https://doi.org/10.32604/biocell.2022.022825
Received 28 March 2022; Accepted 24 May 2022; Issue published 10 August 2022
Abstract
Arbuscular mycorrhizal (AM) fungi reside in the rhizosphere and form mutualistic associations with plant roots. They promote photosynthesis, improve stress resistance, and induce secondary metabolite biosynthesis in host medicinal plants. The AM fungi that are symbiotic with medicinal plants comprise a wide array of species and have abundant germplasm resources. Though research on the AM fungi in medicinal plants began relatively recently, it has nonetheless become an investigative hot spot. Several scholars have explored the diversity and the growth-promoting effects of mycorrhizal fungi in hundreds of medicinal plants. Current research on symbiotic AM fungi in medicinal plants has focused mainly on the effects of inoculating host plants with symbiotic mycorrhizal fungi. However, research on the symbiotic AM fungi in medicinal plants continues to expand, and further study is required to determine the mechanisms by which AM fungi interact with host plants. This paper introduces the diversity of symbiotic AM fungi of medicinal plants and the effects of AM fungi on rhizosphere soil of medicinal plants, including soil structure, microbiota, enzyme activities, etc. This review focuses on the effects of AM fungi on medicinal plants, including antioxidant enzyme systems, drought resistance, nutrient absorption profiles of macro- and micronutrients, accumulation of secondary metabolites such as terpenes, phenolic compounds, and nitrogenous compounds, and prevention of diseases. This review is expected to provide a reference for the application of AM fungi in medicinal plant cultivation, biological control, resource conservation, and the sustainable development of the traditional Chinese medicine industry.
Keywords
Market demand for medicinal plants has increased over recent years. However, wild medicinal plant resources have become scarce or even endangered. Primitive, incompatible artificial cultivation technology has degraded germplasms, lowered the quality of medicinal herbs, destabilized their medicinal properties, and limited the development of the traditional Chinese medicine (TCM) industry. Studies have shown that arbuscular mycorrhizal (AM) fungi use hyphae and other structures to expand the nutrient absorption range of plants, help medicinal plants acquire water from the soil and improve plant drought resistance and alleviate stress (Li and Chen, 2012; Xing et al., 2019a). AM fungi can optimize soil structure and microbiota, such as soil fungi, bacteria, and actinomycetes (Ou et al., 2016; Wei et al., 2021). AM fungi play an important role in improving soil fertility (Li and Zhao, 2005; Liu et al., 2012; Ye et al., 2013), increasing soil protease, urease, phosphatase, and invertase activities (Ou et al., 2016; Jia et al., 2020; Wei et al., 2021), and promoting the absorption and transportation of macronutrient and micronutrient profiles such as C, N, P, Fe, Cu, and Zn in plants (Qi et al., 2006; Shi et al., 2016; Wang and Liu, 2017). AM fungi also regulate the plant antioxidant enzyme system, including superoxide dismutase, catalase, and peroxidase steady-states (Lanfranco et al., 2005; Zhang et al., 2016; Zhang et al., 2019a). AM fungi can improve the yield and quality of active ingredients of medicinal plants, including terpenes, phenolic compounds, and nitrogenous compounds (Mandal et al., 2015a; Xie et al., 2020). In terms of diseases control, AM fungi can improve plant defense enzyme activities, regulate host defense gene expression, combat infectious and non-infectious diseases (Xiao et al., 2011; Zhang et al., 2013a), and improve plant disease resistance and enhance plant adaptability to adverse environments (Zhao and Cheng, 2001; Zhu et al., 2011). AM fungi have shown great potential in promoting medicinal plant growth. Elucidating the relationship between AM fungi and medicinal plants is fundamental for improving the quality of the latter, maintaining biodiversity, and promoting the development of the TCM industry (Zeng et al., 2007). This review introduces the diversity of symbiotic AM fungi of medicinal plants and the effects of AM fungi on rhizosphere soil of medicinal plants, including soil structure, soil microbiota, enzyme activities, fertility, etc. This review also focuses on the effects of AM fungi on medicinal plants, including antioxidant enzyme system, drought resistance, nutrient absorption, transportation of macronutrient and micronutrient profiles, accumulation of secondary metabolites such as terpenes, phenolic compounds, and nitrogenous compounds, and the prevention and control of infectious diseases and non-infectious diseases of medicinal plants. The review provides comprehensive information about the application of AM fungi in medicinal plant cultivation, biological control, resource conservation, and sustainable development of the TCM industry.
Diversity of symbiotic AM fungi in medicinal plants
The AM fungi of the Glomeromycota comprise one class, four orders, 12 families, and 34 genera (Mi et al., 2020). Over 300 AM fungal species have been isolated worldwide (Wang and Liu, 2017). Global research on the diversity of symbiotic AM fungi associated with medicinal plants began relatively recently but has nonetheless become a priority. There are abundant AM fungi resources in the rhizospheres of medicinal plants (Table 1). Parmita et al. (2008) examined the rates of AM fungus infection in 40 medicinal plants, including Andrographis paniculata (Burm.f) Nees, Rauwolfia serpentina (L.) Benth.ex Kurz., and Mentha arvensis Linn. from Rajshahi University Campus in Bangladesh. Thirty-five medicinal plants were colonized by AM fungi at rates ranging from 13.3% to 100%. Zubek and Błaszkowski (2009) isolated 30 AM fungi from the rhizospheres of 31 medicinal plants, including Artemisia dracunculus L., Calendula officinalis L., and Hypericum perforatum L. collected from the garden of Medicinal Plants of the Faculty of Pharmacy, Jagiellonian University, Collegium Medicum, Kraków, Poland. Piszczek et al. (2019) found that 35 of the 40 medicinal plant species collected from the Institute of Pharmacology of the Polish Academy of Sciences in Kraków were associated with 22 species of AM fungi. Later, Radhika and Rodrigues (2010) studied AM fungal diversity among 25 families and 36 species of cultivated or wild medicinal plants, including Aloe vera L., Azadirachta indica A Juss., and Eclipta alba (L.) Hassk., collected from various sites in North and South Goa, Western Ghats, Goa Region, India. They detected 30 species of medicinal plants that could form associations with AM fungi and isolated 42 species of AM fungi from five medicinal plant genera.
Chinese scholars have investigated rhizosphere AM fungal germplasm resources and diversity and their growth-promoting effects on hundreds of medicinal plants, including Pinellia ternata (Thunb.) Breit (Shi et al., 2017), Polygonum multiflorum Thunb. (Luo et al., 2013), Broussonetia papyrifera (L.) L’Hert (He et al., 2007), Angelica dahurica (Fisch. ex Hoffm.) Benth. et Hook. f. ex Franch. e (Zhao and He, 2011), Atractylodes lancea (Thunb.) DC. (Zhang et al., 2011), Camptotheca acuminata Decne. (Zhao et al., 2006), Artemisia annua L. (Huang et al., 2011), Pogostemon cablin (Blanco) Benth. (Liu, 2020), Rheum palmatum L. (Yang et al., 2018), Fritillaria taipaiensis P. Y. Li (Zhang et al., 2020), Salvia miltiorrhiza Bunge. (Yang, 2012), and Paris polyphylla var. yunnanensis (Franch.) Hand.-Mzt. (Huang et al., 2020). In Xiamen, China, Jiang (2012) isolated 63 AM fungal species from four genera in the rhizospheres of seven medicinal plant species, including Camptotheca acuminata Decne., Houttuynia cordata Thunb., and Mirabilis jalapa Linn. In Quanzhou, China, 56 species of AM fungi belonging to five genera were collected from the rhizospheres of 28 medicinal plants, including Cyclobalanopsis chungii (F. P. Metcalf) Y. C. Hsu et H. W. Jen ex Q. F. Zh, Phyllanthus emblica L., and Lamium barbatum Sieb. et Zucc. (Jiang, 2012). In Zhangzhou, China, 66 species of AM fungi belonging to five genera were isolated from the rhizospheres of 20 medicinal plants, including Lygodium japonicum (Thunb.) Sw., Alpinia officinarum Hance. and Ardisia crenata Sims. (Jiang, 2012). Shi et al. (2017) identified 20 AM fungi belonging to three genera in the rhizospheres of Pinellia ternata (Thunb.) Breit., collected from six locations in Zhejiang and Guizhou, China. Glomus was the dominant AM fungal taxon. In Hunyuan County, Shanxi Province, China, Liu et al. (2019b) isolated and identified 12 species of AM fungi belonging to three genera from the rhizospheres of wild Astragalus membranaceus (Fisch.) Bge. var. Mongholicus (Bge.) Hsiao. Six species of AM fungi belonging to three genera were gathered from the rhizospheres of growing Astragalus membranaceus (Fisch.) Bge., cultivated in Shanxi University of Chinese Medicine, Shanxi Province, China (Liu et al., 2019b). In the Karst region of China, Zhang et al. (2013a) detected 42 species and two genera of AM fungi in the rhizospheres of Rosa laevigata Michx and Glomus was the dominant AM fungal taxon. In Guiyang, China, Luo et al. (2013) found 55 species of AM fungi belonging to four genera in the rhizospheres of Polygonum multiflorum (Thunb.) Harald. Cao et al. (2020) used high-throughput sequencing analysis and identified three classes, four orders, eight families, and nine genera of AM fungi in the rhizospheres of Atractylodes lancea (Thunb.) DC in Chongqing (China). Ran et al. (2021) used staining microscopy and high-throughput sequencing technology to determine the rhizosphere AM fungi of Panax quinquefolius L. cultivated in Shandong Province, China, which included three genera, three families, three orders, one class, and one phylum. Yang et al. (2022) used Illumina MiSeq technology to detect 10 species of three genera of AM fungi in the rhizosphere soil of Ophiopogon japonicus (L. f.) Ker-Gawl. in cultivated and wild habitats. Song et al. (2019) identified AM fungi belonging to 14 genera and eight families in the rhizosphere soil of Sophora flavescens in Shanxi Province, China, using Illumina MiSeq technology. The foregoing studies generally indicated that most of the AM fungi in symbiotic association with medicinal plants were of Glomus spp. AM fungal identification has progressed from simple morphological to advanced high-throughput sequencing methods, which rapidly and accurately clarify AM fungal diversity in medicinal plants.
Effects of AM fungi on medicinal plant rhizospheres
AM fungi exist as spores in the soil, which grow into hyphae under appropriate conditions (Shang et al., 2011). In the absence of a host, AM fungi can sustain for 2–4 weeks by utilizing their own energy sources such as triacylglycerides (Requena et al., 2000, 2007) and retract the hyphal cytoplasm into the spores (Bécard et al., 2004; Tang, 2016). After invading the host plant, AM fungi mainly colonize root cortex cells, intercellular spaces, and the root surface. Structures like hyphae, vesicles, clumps, and spores can be observed after staining (Zhang et al., 2019b). Mycelia produce glomalin-related soil protein (GRSP), a class of glycoproteins that are insoluble in water, extremely stable under natural conditions, and contain metal ions (Xing et al., 2019a). GRSP is distributed on the mycelial surface; it falls onto the soil to become carbon source (Kong, 2013) and acts as a soil structure engineer. The super glue function of adhering soil particles is 3–10 times stronger than that of other soil carbohydrates (Xing et al., 2019b). On the one hand, GRSP blocks the extraradical hyphae of AM fungi in rhizosphere soil, which helps plants to utilize hyphae to acquire soil moisture and prevent other microbial disturbances (Li and Zhao, 2005; Liu et al., 2012; Ye et al., 2013). On the other hand, GRSP enters the soil concomitantly with the degradation of hyphae, thus improving the soil microenvironment and structural stability (Li and Zhao, 2005; Liu et al., 2012; Ye et al., 2013).
GRSP is synthesized and secreted by symbiotic AM fungi in medicinal plants. It optimizes the structure, regulates the microbiota, improves the activity of enzymes, enhances fertility, and improves the matrices of soils. These effects are conducive to medicinal plant growth (Li and Zhao, 2005; Liu et al., 2012; Ye et al., 2013). Zhou et al. (2020) conducted a greenhouse pot experiment and showed that inoculation with various AM fungi increased the content of easily extractable GRSP (e-GRSP) and total GRSP (t-GRSP) and was an important source of organic carbon in the rhizosphere of Paris polyphylla var. yunnanensis (Franch.) Hand.-Mzt. Li et al. (2017) showed that e-GRSP and t-GRSP are significantly positively correlated with available soil phosphorus (P) and alkaline phosphatase activity. AM fungi adhere to soil particles by secreting GRSP and forming microaggregates composed of polyvalent ion bridges and polysaccharides (Xing et al., 2019b). Microaggregates combine with fungal hyphae and plant roots to form large, stable soil aggregates (Xing et al., 2019b). Hyphal networks and aggregate structures provide gas exchange channels and space for root expansion, promote dry-wet circulation, and improve soil water infiltration, tillage, and fertility (Xing et al., 2019b).
AM fungi increase soil invertase, urease, and catalase activities and help plants metabolize, transform, and utilize carbon, nitrogen (N), and P, and K phytotoxic peroxide decomposition. Jia et al. (2020) showed that single strains of Glomus tenebrosum and Glomus formosanum most effectively improved invertase and phosphatase activities and urease activity, respectively, in the rhizosphere of Salvia miltiorrhiza Bunge. Wei et al. (2021) found that inoculation with mixed AM fungi increased protease, phosphatase, invertase, and catalase activities in the rhizosphere of Saussurea costus (Falc.) Lipsch. Ou et al. (2016), Huang et al. (2020), and Zhou et al. (2020) found that inoculation with mixed AM fungi increased protease, urease, phosphatase, invertase, and catalase activities in the rhizosphere of Paris polyphylla var. yunnanensis. Therefore, AM fungi play a key role in promoting soil enzyme activities.
AM fungi can optimize soil microbial structure and support plant growth and development by regulating soil fertility and microbial abundance and diversity in the rhizosphere. The field experiment of Huang et al. (2020), the greenhouse pot experiment of Zhou et al. (2020), and the investigation of Ou et al. (2016) on 28 types of AM fungi infecting Paris polyphylla Smith var. yunnanensis (Franch.) Hand.-Mazz. showed that the symbionts increased the numbers of bacteria, actinomycetes, and other microorganisms in host plant rhizospheres, improved microbial diversity, and augmented soil fertility. Wei et al. (2021) found that the inoculation of an AM fungal mixture increased the numbers of actinomycetes and bacteria without changing the pH of the Saussurea costus (Falc.) Lipsch. rhizosphere. Actinomycete and bacterial growth rates surpassed those of the fungi, and their numbers were significantly positively correlated. Whereas AM fungi regulate the numbers of soil microorganisms, the latter use mycorrhizal hypha and spores as nutrient sources for their own growth and development. Hence, they facilitate nutrient utilization (Liu et al., 2012; Ye et al., 2013). Gu et al. (2020) showed that the available P and available potassium (K) contents in the rhizosphere of Paris polyphylla var. yunnanensis seedlings are increased by inoculation with nine AM fungi treatment groups, including treatment group S1 (Gigaspora rosea, Gigaspora albida, Gigaspora margarita, and Gigaspora gigantea), and treatment group S2 (Scutellospora calospora, Cetraspora pellucida, Scutellospora coralloidea, and Dentiscutata erythropus). Huang et al. (2020) showed that when the inoculation of exogenous AM fungi in treatment groups S1 (Scutellospora coralloidea, Scutellospora calospora, Cetraspora pellucida, and Dentiscutata erythropus) and S2 (Scutellospora calospora, Cetraspora pellucida, Gigaspora margarita, Gigaspora gigantea, Septoglomus deserticola, and Claroideoglomus claroideum) did not significantly alter soil pH, both treatment groups significantly increased available N, P, and K in the rhizosphere of Paris polyphylla var. yunnanensis (Franch.) Hand.-Mzt., promoted soil N, P, and K accumulation, and enhanced the soil nutrient-holding capacity and matrix. In summary, AM fungi are of great importance to soil microbial quantity and soil fertility regulation.
Effects of AM fungi on drought resistance in medicinal plants
Under normal circumstances, the production and elimination of reactive oxygen species (ROS) in plants are in a dynamic balance to a certain extent. Drought stress results in the production and accumulation of ROS in plants (Wang et al., 2019). Excessive accumulation of ROS and the depletion of antioxidant enzymes disrupt the steady-state of the plant antioxidant enzyme system (Wang et al., 2019). Oxidative stress-mediated by elevated ROS levels will lead to harmful reactions such as peroxidation of plant biofilms and amino acid oxidation (Lushchak, 2011; Wang et al., 2019). AM fungi play a key role in the plant antioxidant enzyme system. In Poncirus trifoliata, AM fungi regulate the expression of PtFe-SOD, PtPOD, PtCAT1, PtADC, P5CS, PtPIPs, PtNIPs, and plant antioxidant enzyme genes (Lanfranco et al., 2005; Zhang et al., 2019a), improve the activities of superoxide dismutase (SOD), peroxidase (POD), catalase (CAT), and other enzymes in the plant antioxidant enzyme system (Lanfranco et al., 2005; Zhang et al., 2019a), increase the activity of proline dehydrogenase (ProDH), regulate transmembrane water transport between plant cells, and reduce drought-induced oxidative stress and subsequent damage in plants (Zhang et al., 2016). AM fungi improve plant tolerance to alkaline soil by regulating NADPH oxidase and dehydrin genes, transmembrane transporter activity regulation pathway (GO: 0015077), and cell wall regulation pathway (GO: 0052386) (Ding, 2021). Zhang et al. (2019a) showed that AM fungi upregulate the expression of PtFe-SOD, PtMn-SOD, PtPOD, PtCAT1, PtADC1, and PtADC2 genes of the host plant Poncirus trifoliata (L.) Raf. Sun (2014a) reported that AM fungi increase leaf water content and promote the removal of ROS in plants by upregulating the expression of PtADC1 and PtADC2 genes under drought conditions. In a pot experiment, Zhao and He (2011) inoculated AM fungi and observed significantly improved SOD and CAT activities in the leaves of Angelica dahurica (Fisch. ex Hoffm.) Benth. et Hook. f. ex Franch. e. Qi et al. (2006) found that inoculating AM fungi under drought conditions improved SOD and POD activities, reduced the malondialdehyde (MDA) content, and alleviated the adverse effects of drought stress in the leaves of Diospyros lotus L. Zhang et al. (2016) found that mycorrhizal fungi increased SOD and POD activities and mitigated physiological damage in Cyclobalanopsis glauca (Thunberg.) Oersted. seedlings under moderate drought stress. Zhang et al. (2020) found that inoculating AM fungi enhanced stress resistance in Fritillaria taipaiensis P. Y. Li by increasing POD, SOD, and CAT activities.
Drought resistance is also associated with the proline and soluble sugar levels in plant cells and tissues. Under drought conditions, plants synthesize abundant proline for osmotolerance and osmoregulation. Plants inoculated with AM fungi maintain low proline levels to protect their enzyme function. Zou et al. (2013) found that AM fungi reduce the activity of Δ(1)-pyrroline-5-carboxylate synthetase (P5CS), increase the activity of proline dehydrogenase (ProDH), and decrease proline accumulation in the host plant trifoliate orange. Zhao and He (2011) showed that the foliar proline and soluble sugar levels were lower and higher, respectively, in Angelica dahurica (Fisch. ex Hoffm.) Benth. et Hook. f. ex Franch. e inoculated with AM fungi than those in the leaves of uninoculated plants. Zhang et al. (2016) reported that mycorrhizal fungi promoted soluble sugar biosynthesis, lowered the osmotic potential, and improved water utilization in Cyclobalanopsis glauca (Thunberg.) Oersted. under drought stress. Also, the soluble sugar and soluble protein contents of Fritillaria taipaiensis P. Y. Li increased after inoculation with AM fungi compared with the control group (Zhang et al., 2020).
AM fungal hyphae play an important role in water absorption by plants. AM fungal hyphae penetrate into the cortical cells of plant roots to form dendritic or cauliflower-like branched arbuscular structures, which act as convenient water transport channels for plants and also enhance the water holding capacity of the soil. Extra-root hyphae increase the length of plant roots and form a huge hyphal network in the soil, thereby enhancing the hydraulic conductivity of roots and promoting water absorption. AM fungi flexibly regulate water transport and redistribution and leaf transpiration rate in the host plant according to the water content and demand, thereby promoting a dry/wet cycle (Xing et al., 2019a).
In addition to absorbing water via AM fungal hyphae, plants also rely on aquaporins (AQPs) to regulate transmembrane water transport between plant cells. Under drought stress, AM fungi regulate the gene expression of plasma membrane intrinsic proteins (PIPs) and vacuolar intrinsic proteins (TIPs) of host plant AQPs, mediate water absorption and efflux, regulate swelling pressure, osmotic pressure, and root water conductivity (Fotiadis et al., 2001), promote water absorption and retention, improve water metabolism, and maintain water balance in plant cells (Li and Chen, 2012; Xing et al., 2019a; Yu et al., 2021). Porcel et al. (2006) found that inoculation with AM fungi reduces the influence of drought-induced stress on the transcription products of the aquaporin gene GmPIP2. Under drought stress, AM fungi downregulate the expression of seven PtPIPs and three PtNIPs in the roots of Poncirus trifoliata (L.) Raf. (Zou et al., 2019). Under drought conditions, AM fungi upregulate RpPIP2;1 and RpTIP2;1 and downregulate RpTIP1;1 and RpPIP1;3 gene expression in Robinia pseudoacacia L. (He et al., 2016). AM fungi upregulated the expression of LbPIP2-1, LbTIP3-1, and Rir-AQP2 genes in host plants (Hu, 2017). In 200 mM NaCl, the expression of RpPIP1;1, RpPIP2;1, and RpTIP1;1 aquaporin genes in plants harboring AM fungi was higher than in non-mycorrhizal plants (Chen et al., 2017).
AM fungi use their hyphae (Zhang et al., 2019b; Xing et al., 2019a), channel proteins (Fotiadis et al., 2001), and other structures to regulate the free and bound water levels (Yang and Liao, 2005; Li and Chen, 2012; Chen et al., 2014) and proline and soluble sugar content, improve osmotic pressure, root hydraulic conductivity, enzymatic clearance, and antioxidant systems, and water metabolism, attenuate drought stress, enhance water retention and photosynthesis, and strengthen drought resistance in host plants.
Effects of AM fungi on nutrient absorption in medicinal plants
Mycorrhizal fungi provide 90% of the nutrients to the host plants, which in return provide 30% of the photosynthetic carbon assimilation products to the mycorrhizal fungi, thus helping the fungi complete their life cycle while facilitating the redistribution of nutrients (Drigo et al., 2010; Heijden et al., 2015). During P deficiency, AM fungi help plants to obtain 50–75% of the P requirement (Mbodj et al., 2018; Wang et al., 2020). External hyphae absorb N and contribute 30% of the total nitrogen absorbed by the plants (Li and Zhang, 2008; Wang et al., 2020). AM fungi promote the uptake of N, P, K, and other elements by upregulating the expression of LbAMT1.1, LbNRT3.1, LbPT3, and LbKT1 genes (Hu, 2017; Wang, 2020; Zhao, 2021).
AM fungi upregulate ammonium transport genes such as LbAMT1.1 and nitrate transport genes such as LbNRT2.4 and LbNRT3.1 to promote N uptake in host plants (Zhao, 2021). Extracellular AM fungal hyphae and penetration of the high-affinity NH4+ transporter GintAMT1 in the intracellular hyphae of host plant root cortical cells were induced under low NH4+ and repressed under high NH4+. By these mechanisms, N absorption was induced in host plants (Pérez-Tienda et al., 2011; Vieira et al., 2019; Shao et al., 2021). The symbiosis between AM fungi and host plants stimulates the uptake of ammonium, nitrate, complex organic N, and other substances and their conversion into arginine in the extraradical hyphae. The arginine is then transported to the intraradical hyphae and converted to NH4+, which regulates host plant N metabolism (Yang et al., 2011b; Shirzad and Ahmad, 2020).
In the case of P deficiency, the expression of GvPT, GiPT, and GmosPT P-transporter genes in AM fungi was enhanced by extracellular hyphae, and P uptake by mycorrhizal fungi was promoted (Xu et al., 2017; Xue et al., 2019). Phosphate uptake mainly depends on phosphate transporter (Pht) (Wang et al., 2020). AM fungi upregulate Pht1 gene expression in the pre-branch site and promote P uptake when mycorrhizal fungi transport P to the arbuscular (Xue et al., 2019; Wang et al., 2020). Under short-term and long-term salt stress conditions, mycorrhizal symbiosis selectively regulates the expression of ZjPHT1.1, ZjPHT1.4, ZjPHT1.11, and ZjPHT1.5 genes and affects plant P transport (Ma, 2020). AM fungi promote the gene expression of P transporters such as LbPT3, LbPT4, and LbPT5, thereby promoting P uptake by the host plants (Hu, 2017).
In the uptake of K, upregulation of Solanum lycopersicum High-affinity Potassium Transporter10 (SlHAK10) gene expression by AM fungi promotes K+ uptake by plants (Liu et al., 2019a). AM fungi promote plant K uptake and transport by increasing the expression levels of LbKT1 and LbSKOR (Zhang et al., 2017a) and promote uptake of K by inducing the expression of LbKAT3, LbHAK, LbKT1, and LbSKOR genes related to mediating K uptake, release and transport (Wang, 2020). Estrada et al. (2013) showed that ZmAKT2, ZmSOS1 and ZmSKOR were related to Na+ net absorption and long-distance transportation, xylem and phloem K+ transportation and release. Also, AM fungi maintained Na+ and K+ homeostasis in host plants by up-regulating the above gene expression (Estrada et al., 2013).
AM fungi expand the nutrient type and content absorption via their hyphae and other structures. They play active roles in improving chlorophyll content, net photosynthetic rate, and plant nutrient absorption. The pot experiment of Zhao and He (2011) disclosed that AM fungi promoted photosynthesis by increasing the chlorophyll a, total chlorophyll, and carotenoid content in Angelica dahurica (Fisch. ex Hoffm.) Benth. et Hook. f. ex Franch. e leaves. Huang et al. (2011) indicated that inoculation with AM fungi increased the chlorophyll content and transpiration and net photosynthetic rates and promoted N, P, and K uptake in Artemisia annua L. Yang (2012) suggested that AM fungi promoted foliar Fe, Mn, Mg, and Ca absorption and root Cu, Mn, and Fe uptake in Salvia miltiorrhiza Bunge. Huang et al. (2020) found that inoculation with exogenous AM fungi cause the accumulation of N, P and K in Paris polyphylla var. yunnanensis (Franch.) Hand.-Mzt. Sun et al. (2013) proposed that inoculation with AM fungi promote N, P, and K absorption and utilization, inhibit Na uptake, and fortify saline-alkaline resistance in Glycyrrhiza uralensis Fisch.
Effects of AM fungi on secondary metabolite accumulation in medicinal plants
The active principle content is a quality criterion of medicinal plants. Active substances in medicinal plants include secondary metabolites such as terpenes (sesquiterpene, etc.), phenolic compounds (flavonoids, etc.), and nitrogenous compounds (alkaloids, etc.) (Zhang et al., 2015). AM fungi are of great significance in regulating gene expression in medicinal plants, which improves the yield of secondary metabolites and the overall medicinal quality (Mandal et al., 2015b; Xie et al., 2020).
Terpenes. In plant terpenoid biosynthesis, the substrate isopentenyl pyrophosphate and its isomer dimethylallyl diphosphate are obtained via the 2-C-methyl-D-erythritol-4-phosphate pathway (MEP pathway) in plastids and mevalonate pathway (MVA pathway) in the cytoplasm (Wang et al., 2013; Xie et al., 2020). In the MEP pathway, 1-deoxy-D-xylulose 5-phosphate synthase (DXS) and 1-deoxy-D-xylulose 5-phosphate reductoisomerase (DXR) are the two key enzymes (Xie et al., 2020), while 3-Hydroxy-3-methylglutaryl coenzyme A reductase (HMGR) is the key enzyme in MVA pathway (Chappell et al., 1995). Huang et al. (2011) and Chaudhary et al. (2008) showed that inoculation of AM fungi increased the artemisinin (sesquiterpene) and essential oil content in Artemisia annua L. to varying degrees depending on the plant organ or tissue. It was also reported that AM fungi increased artemisinin accumulation in the host plant by upregulating key genes involved in artemisinin biosynthesis, including ADS, DBR2, and ALDH1 (Mandal et al., 2015b; Xie et al., 2020). Maes et al. (2011) and Mandal et al. (2015b) demonstrated that jasmonic acid (JA) promotes glandular synthesis and upregulates the expression of artemisinin synthesis genes, causing artemisinin accumulation. AM fungi upregulate the expression of DXS and DXR genes in the leaves of Stevia rebaundiana Bertoni and promote stevioside accumulation (Mandal et al., 2015a). Relative MtDXS2 expression and carotenoid content increased in Ocimum basilicum L. var. inoculated with AM fungi (Copetta et al., 2006). AM fungi upregulate HMGR gene expression and promote alpha- and beta-amyrin accumulation in the roots of Zea mays L. (Gerlach et al., 2015). Inoculation of AM fungi increased the auxin (indole-3-acetic acid), content and promoted the accumulation of organic matter and patchouli alcohol in Pogostemon cablin (Blanco.) Benth. (Liu, 2020). In a greenhouse pot experiment, the content and structure ratios of dehydrocostus lactone, costunolide, and other lactones were increased to varying degrees in Saussurea costus (Falc.) Lipsch treated with exogenous AM fungi (Zhang et al., 2017b). The saponin content in Panax notoginseng (Bruk) F. H. Chen markedly increased in response to 20–30% AM fungi infection intensity (Zeng et al., 2015). Li et al. (2021) found that AM fungi inoculation increased the content of polyphyllin, the active substance of Paris polyphylla var. yunnanensis, and this was related to the expression of PpSE and PpHMGR genes. PkSS1, PkSS2, PkFPS1, PkCAS1, and other genes coding for key enzymes of steroid saponin synthesis respond differently to AM fungi and P levels in different parts of Polygonatum kingianum Coll. et Hemsl. (Zhang, 2021). Under the interaction of AM fungi and P levels, the differentially expressed genes are mainly enriched in ribosomes, biosynthesis of secondary metabolites, and metabolic pathways (Zhang, 2021). AM fungal infection improved the content of fat-soluble tanshinone I and miltionone in the roots of Salvia miltiorrhiza Bunge (Yang, 2012). Zubek et al. (2010) reported that AM fungi significantly increased the thymol content in the roots of Inula ensifolia L.
Phenolic compounds. The synthesis of phenolic compounds is related to cascade signaling pathways of signal molecules H2O2, salicylic acid (SA), and nitric oxide (NO) (Zhang et al., 2013b; Zhang et al., 2015). Signal molecule SA regulates the gene expression of systemic acquired resistance (SAR) in plants and induces the synthesis of phenolic compounds (Kogel and Langen, 2005; Zhang et al., 2010). NO is involved in the regulation of chalcone synthase (CHS) expression, and an NO donor enhances H2O2 accumulation (Soheila et al., 2001; Wang et al., 2006). Under N deficiency conditions, H2O2 and NO jointly regulate the activity of phenylalanine ammonia-lyase (PAL) and the accumulation of phenolic substances in Matricaria chamomilla L. (Kováčik et al., 2009). Zhang et al. (2010) speculated that the mechanism and pathway of phenolic synthesis induced by AM fungi as follows: after infecting plant roots with AM fungi, SA and H2O2 were induced in-situ, and the enzyme activity related to phenolic biosynthesis centered on PAL was stimulated, and the phenolic content in infected plant roots was increased. At the same time, SA and H2O2 can be used as signal molecules to transport to the sites not infected by AM fungi at a long distance in the plant transport tissues, induce the expression of CHS, PAL, and other genes, and promote the synthesis of phenolic compounds in roots not infected by AM fungi in plants. (Zhang et al., 2010). Studies have shown that AM fungi increase the H2O2, SA, and NO contents in roots, and H2O2, SA, and NO signaling molecules activate PAL and participate in the synthesis of phenolic compounds in plant roots (Zhang et al., 2013b). AM fungi promote total flavonoid accumulation in Clematis intricata Bunge by up-regulating CiCHS expression (Liu, 2017). Through greenhouse experiments and field trials, Ceccarelli et al. (2010) found that inoculation with AM fungi increased the total phenolic content and anti-ROS capacity of artichoke (Cynara cardunculus L. var. scolymus). Jurkiewicz et al. (2010) showed that inoculation with AM fungi increased the phenolic acid content in the roots and buds of Arnica montana L. Zubek et al. (2012) demonstrated that inoculation with AM fungi, such as Rhizophagus intraradices, increased the levels of the anthraquinone derivatives hypericin and pseudohypericin in Hypericum perforatum L. Toussaint et al. (2007) disclosed that inoculation with AM fungi promoted the accumulation of the antioxidants rosmarinic and caffeic acid in Ocimum basilicum L. stems. Yang (2012) reported that inoculation with AM fungi increased the content of water-soluble salvianolic acid B and rosmarinic acid in Salvia miltiorrhiza Bunge. Inoculation with AM fungi promoted P uptake and enhanced the glycyrrhizin content in licorice (Glycyrrhiza uralensis) Fisch. leaves and roots, respectively (Liu et al., 2007).
Nitrogenous compounds. Studies have shown that the alkaloid biosynthetic pathway is similar to the terpene biosynthetic pathway, which can be synthesized by MVA or MEP pathways, such as Dendrobium alkaloids (Bai, 2019). MVA pathway is the main pathway to produce steroidal alkaloid backbones (Suzuki and Muranaka, 2007; Chen, 2019). HMGR is a key MVA pathway regulator (Chen, 2019). First, cycloartenol synthase (CAS) cyclizes 2,3-oxidosqualene to form an important precursor for the synthesis of Fritillaria alkaloids, namely cycloartenol (CA) and then forms various Fritillaria alkaloids through a series of complex reactions (Sun, 2014b; Chen, 2019). CAS is considered to be the key enzyme for the synthesis of Fritillaria alkaloids (Sun, 2014b; Chen, 2019). Chen (2019) cloned two genes related to alkaloid synthesis in Fritillaria cirrhosa D. Don, namely HMGR gene (FcHMGR) and CAS gene (FcCAS), and found that the increase of alkaloid content in Fritillaria cirrhosa D. Don with age may be positively correlated with the high expression of genes. Inoculation with Claroideoglomus etunicatum changed the accumulation of monoterpene indole alkaloids (MIAs) such as ajmalicine and serpentine in different parts of Catharanthus roseus (L.) G. Don, and the expression patterns of TDC, STR, SGD, DAT, PRX1, and other analytical genes of alkaloids (Andrade et al., 2013). The q-markers peimisine, onych-peimisine, and aethyl-peimisine were enhanced in Fritillaria taipaiensis P. Y. Li inoculated with AM fungi (Zhang et al., 2020). Phellodendron amurense Rupr. from three different provenances (including space breeding and Jilin, China, and Heihe, China) were infected with three different AM fungi: Rhizophagus intraradices, Claroideoglomus etunicatum, and Funneliformis mosseae. The levels of three alkaloids, including palmatine, berberine, and jatrorrhizine, increased in various plant fractions (Zhou et al., 2015). Zhou et al. (2015) also showed that Funneliformis mosseae had the most significant effect on berberine and jatrorrhizine, and Rhizophagus intraradices had the most significant effect on palmatine. Fan et al. (2006) found that inoculation of Funneliformis mosseae, Claroideoglomus etunicatum, Glomus versiforme, and Oehlia diaphana increased the content of berberine, jatrorrhizine, and palmatine in Phellodendron amurense Rupr. Zhou and Fan (2007) found that AM fungi promoted the accumulation of berberine in Phellodendron chinense Schneid. through the greenhouse pot experiment of inoculating Phellodendron chinense Schneid. with seven AM fungi, including Acaulospora laevis, Acaulospora mellea, Oehlia diaphana, Claroideoglomus etunicatum, Rhizophagus intraradices, Funneliformis mosseae, and Glomus versiforme. Zhao et al. (2006) conducted greenhouse pot experiments on Camptotheca acuminata Decne. inoculated with Oehlia diaphana and Acaulospora laevis. Yu et al. (2012) infected Camptotheca acuminata Decne. with Acaulospora mellea and Rhizophagus intraradices. Both studies revealed that inoculation with AM fungi increased the camptothecin content in the host plant. Rojas-Andrade et al. (2003) indicated that in Prosopis laevigata (Willd.) M.C. Johnst. Inoculated with Gigaspora rosea, the shoot pyridine alkaloid content remained constant while the root trigonelline content increased 1.8-fold compared with the uninoculated plants.
Effects of AM fungi on disease resistance in medicinal plants
Medicinal plant diseases may be infectious or non-infectious depending on the nature of the pathogenic factors (Xiao et al., 2011). Non-infectious diseases are caused by non-biological factors, such as the toxic effects of environmental pollutants, drought, and flooding caused by too much or too little soil moisture, burning or freezing caused by high or low temperatures, nutritional imbalances caused by excess or insufficient fertilizer, improper cultivation measures, and planting density and sowing time (Xiao et al., 2011). AM fungi alleviate non-infectious diseases by expanding the absorption range of plant roots with their hypha, promoting nutrient absorption, and improving plant stress resistance and survival rates (Xiao et al., 2011; Yang, 2012).
Diseases caused by fungi, bacteria, viruses, nematodes, and other contagious pathogens are called infectious diseases (Xiao et al., 2011). AM fungi resist infectious diseases by increasing defense enzyme activity and upregulating defense genes in the host and by improving the sensitivity of the plant defense system to pathogens (Xiao et al., 2011; Zhang et al., 2013a). Plants induce the expression of pathogenesis-related (PR) genes PR1 and PR5, PDF1, and Thi2.1 (Berrocal and Molina, 2007; Sukhada et al., 2011; Wang et al., 2012) through SA, JA, and NO signaling pathways (Spoel and Dong, 2008; Schmidt et al., 2008; Wang et al., 2012) to establish their own defense mechanisms against pathogen invasion. SA pathway induces the expression of PR genes (Ganesan and Thomas, 2001). Infection by AM fungi can enhance the cDNA expression of the main JA synthase allene oxide cyclase and increase the endogenous JA content in roots (Isayenkov et al., 2005). AM fungi improve JA levels and enhance plant defense by inducing the synthesis of key enzymes in the JA pathway, such as lipoxygenase, chitinase (Xie et al., 2012), and allene oxide synthase (Mandal et al., 2015b). Jasmonic acid carboxyl methyltransferase (JMT) catalyzes JA to form methyl jasmonate (MeJA) with strong volatility (Li et al., 2019). The effect of MeJA varies on the expression levels of GubAS, GubAO, and GuDOCS genes in Glycyrrhiza uralensis Fisch. under different concentrations and different durations of treatment, thereby affecting plant chemical defense (Liang et al., 2017; Li et al., 2019). NO accumulation is partly dependent on the activities and functions of DMI1, DMI2, and DMI3 (Wang et al., 2012). Inoculation of AM fungi elicits high mRNA expression of extracellular PR-1, intracellular GluBAS, and Chi9 in host plant mutants (Gao et al., 2004, 2006; Wang et al., 2012). Huang et al. (2007) found that AM fungi stimulate plant disease resistance mechanisms by upregulating the genes encoding the biosynthesis of secondary metabolites such as 2,4-dihydroxy-7-methoxy-2H-1,4-benzoxazin-3(4H)-one. Yang (2012) found that inoculation with AM fungi and pathogens increased chitinase and β-1,3-glucanase activity, upregulated PR10 and PDF2.1, and improved disease resistance in Salvia miltiorrhiza Bunge. In an earlier report, Li (2002) suggested that inoculation with AM fungi increased POD, PAL, and β-1,3-glucanase activity and regulated PAL5, Chib1, and VCH3 expression at the transcriptional level in host plants. In this way, nematode infestations were inhibited. When AM fungus-inoculated host plants were reinfested with the soybean cyst nematode (SCN; Heterodera glycines) (infectious disease), their defense genes were upregulated. According to the statistics of Veresoglou and Rillig (2012), differences in the efficiency of nematode pathogens depend on the number of AM isolates. Carlsen et al. (2008) demonstrated that AM fungi reduced the biomass of Pythium ultimum (infectious disease) by modulating the flavonoid content in white clover (Trifolium repens L.). Compared with the control, replanted Ginkgo biloba L. inoculated with three AM fungi presented with lower leaf blight disease indices, enhanced growth, and successful continuous cropping (Qi et al., 2002). The use of microorganisms to control plant pests and diseases is an important means of biological control (Huang et al., 2011). AM fungi play vital roles in plant disease resistance. Nevertheless, their practical application in plant disease control remains to be developed. Small-scale application of AM fungi for biological control cannot completely replace conventional agrochemicals (Xiao et al., 2011).
Traditional methods of research on AM fungal diversity in medicinal plants have certain limitations. AM fungi are still identified mainly based on conventional physiological ecology, morphology, and histochemistry. However, the classification of AM fungi may be affected by certain discrepancies in morphological description resulting from examining different stages of plant development, using different sampling times and staining techniques, and human error (Chen et al., 2011). With the development of molecular biological techniques, more precise means of identifying AM fungi currently employ second-generation high-throughput sequencing (HTS), including Illumina (Solexa), Roche/454, and Applied Biosystems solid, among others (Chen et al., 2011). Among them, Illumina (Solexa), which utilizes single-molecule arrays to bridge polymerase chain reactions on a minichip (flow cell) (Mardis, 2008; Du et al., 2014), has gradually become a popular sequencing platform, being high throughput, accurate, sensitive, and low-cost technique (Du et al., 2014; Tang, 2016). Nevertheless, the methods used to assess fungal symbiosis in agriculture and ecology are not fully transferable to symbiotic AM fungi in medicinal plants as the latter include a wide range of different taxa that occupy diverse and complex habitats. Hence, soil texture, microbial abundance and diversity, root morphology, and sampling time must be considered in the processes of isolating and identifying symbiotic AM fungi in medicinal plants (Chen et al., 2011).
Most studies on the growth-promoting effects of AM fungi on medicinal plants were performed as pot experiments; little field research was conducted. Until now, studies have been reported on the use of AM fungi, including Acaulospora laevis (Zhou and Fan, 2007), Oehlia diaphana (Zhou and Fan, 2007), Claroideoglomus etunicatum (Andrade et al., 2013), Rhizophagus intraradices (Zhou et al., 2015), Gigaspora rosea (Gu et al., 2020), Gigaspora albida (Gu et al., 2020), Scutellospora calospora (Huang et al., 2020), Scutellospora coralloidea (Huang et al., 2020), and others to investigate the inoculation effect of medicinal plants. Most of these inoculation experiments were performed on potted plants raised in a greenhouse and/or under artificial interventions such as high-temperature, drought, or salt stress. Under the foregoing conditions, AM fungal inoculation is usually beneficial to the host plant. Nevertheless, soil volume and water and nutrient content differ between field and greenhouse pot experiments. Furthermore, in these reports, AM fungi were comparatively less efficacious in the field-than in greenhouse-grown plants. High temperature accelerates evaporation and causes drought, which in turn results in salt accumulation on the soil surface and in plant roots. Hence, plants are often simultaneously subjected to multiple stressors. AM fungi improve plant resistance to a single controllable stress factor. It is not known whether they can also mitigate the negative impact of multiple adverse effects on commercial crops and medicinal plants. It also remains to be determined whether they can benefit host plants under multiple stressors, different soil types and field experiment designs, or long-term cultivation. Future research should also investigate whether medicinal plants can maintain good growth status even after they are no longer being inoculated with AM fungi.
Most prior studies focused on the effects of AM fungi on medicinal plant seedlings. AM fungi increased the terpene, phenolic, and nitrogenous secondary metabolite content of medicinal plants and improved their quality. Subsequent research must aim to elucidate the physiological effects of AM fungi on perennial plants, different plant growth stages, and various secondary metabolites. The impact of AM fungi on the expression of genes encoding secondary metabolites in different plant tissues and organs should also be explored. Moreover, the influences of various AM fungi infection intensities on the host plant and environmental factors on the AM fungi merit further evaluation.
Research on the disease resistance effect of AM fungi on medicinal plants is still in the early stages. AM fungi help host plants resist disease by regulating the expression of their defense genes and improving the sensitivity of their defense systems to pathogens. These services could greatly advance sustainable agriculture. Nevertheless, the application of AM fungus in plant disease control is still under development. Furthermore, small-scale application of AM fungi in biological control cannot completely replace conventional pesticides (Xiao et al., 2011). Future investigations should examine plant defense-related enzyme activity, gene expression, and pathogen sensitivity. The signal transduction pathways among AM and pathogenic fungi, bacteria, and viruses in medicinal plants should also be explored. Other research focal points could include the relationships among root exudates, host genotype, soil microenvironment, biocontrol agents, and active and passive AM fungus defense mechanisms. The relationships among inoculant dosages, disease types, and AM fungal control agents also merit further research. The learnings from the foregoing studies might lay the foundation for improving the soil environment, yield, and quality of medicinal plants.
AM fungi-based fertilizer is promising for medicinal plant cultivation. Research on the diversity and growth promotion of symbiotic AM fungi in medicinal plants has increased in recent years. However, AM fungi are seldom practically applied to medicinal plant production. The isolation and identification of AM fungi by molecular methods and the development of novel, efficacious AM fungi-based fertilizer preparations may enhance the breeding and propagation of endangered wild medicinal plants. Newly discovered AM fungal strains could restore and promote medicinal plant growth, stimulate secondary metabolite biosynthesis, reduce chemical fertilizer and pesticide consumption, augment the therapeutic efficacy of medicinal plants, and increase the ecological and economic value of cultivating these crops. Techniques involving biochemistry, molecular ecology, metabolomics, proteomics, bioinformatics, genotyping, and phenotyping can be used to determine the heritability, germplasm resources, infection rates, diversity, and distribution of AM fungi at the transcriptional and translational levels. The effects of AM fungi colonization on energy distribution, photosynthesis, phytohormones, and secondary metabolites in medicinal plants should also be explored. Moreover, determination of the key roles of AM fungi in medicinal plant cultivation may eventually facilitate novel approaches towards biological control, environmental protection, and the sustainable development of TCM and other constituents of the economy.
Author Contribution: The authors confirm contribution to the paper as follows: study conception and design: Zhongfeng Zhang, Limin Yu; data collection: Limin Yu; analysis and interpretation of results: Limin Yu; draft manuscript preparation: Zhongfeng Zhang, Limin Yu, Longwu Zhou. All authors reviewed the results and approved the final version of the manuscript.
Funding Statement: This work was supported by the National Natural Science Foundation of China (31960272), Basic research fund of Guangxi Academy of Sciences (CQZ-E-1909), and the Guangxi Scientific and Technological Project (Guike AB18126065).
Conflicts of Interest: The authors declare no conflicts of interest associated with this study.
References
Andrade SAL, Malik S, Sawaya ACHF, Bottcher A, Mazzafera P (2013). Association with arbuscular mycorrhizal fungi influences alkaloid synthesis and accumulation in Catharanthus roseus and Nicotiana tabacum plants. Acta Physiologiae Plantarum 35: 867–880. DOI 10.1007/s11738-012-1130-8. [Google Scholar] [CrossRef]
Bai WY (2019). Analysis of Polysaccharides and Alkaloids Biosynthesis Pathway in Dendrobiums (MA Thesis). South China Agricultural University. DOI 10.27152/d.cnki.ghanu.2019.000320. [Google Scholar] [CrossRef]
Bécard G, Kosuta S, Tamasloukht M, Séjalon-Delmas N, Roux C (2004). Partner communication in the arbuscular mycorrhizal interaction. Canadian Journal of Botany 82: 1186–1197. DOI 10.1139/b04-087. [Google Scholar] [CrossRef]
Berrocal LM, Molina A (2007). Arabidopsis defense response against Fusarium oxysporum. Trends in Plant Science 13: 145–150. DOI 10.1016/j.tplants.2007.12.004. [Google Scholar] [CrossRef]
Cai RS, Wang Y, Meng DK, Zhao NN, Gu MH et al. (2020). The diversity of arbuscular mycorrhizal fungi in rhizophere of Pteris vittata L. around the high as areas in Guangxi. Ecology and Environmental Sciences 29: 842–849. DOI 10.16258/j.cnki.1674-5906.2020.04.026. [Google Scholar] [CrossRef]
Cao DX (2008). Ecology Study of Arbuscular Mycorrhizal Fungi in the Rhizospere of Angelica Dahurica in Anguo (MA Thesis). Hebei University. https://kns.cnki.net/KCMS/detail/detail.aspx?dbname=CMFD2011&filename=2010065446.nh. [Google Scholar]
Cao M, Hu KZ, Liu YQ, Liu CL, Tang XY et al. (2020). Arbuscular mycorrhizal fungi diversity in rhizosphere soil of Atractylodes lancea (Thunb.) DC. in Chongqing region by high-throughput sequencing. Microbiology China 47: 2877–2886. DOI 10.13344/j.microbiol.china.200670. [Google Scholar] [CrossRef]
Carlsen SCK, Understrup A, Fomsgaard IS, Mortensen AG, Ravnskov S (2008). Flavonoids in roots of white clover: Interaction of arbuscular mycorrhizal fungi and a pathogenic fungus. Plant and Soil 302: 33–43. DOI 10.1007/s11104-007-9452-9. [Google Scholar] [CrossRef]
Ceccarelli N, Curadi M, Martelloni L, Sbrana C, Picciarelli P et al. (2010). Mycorrhizal colonization impacts on phenolic content and antioxidant properties of artichoke leaves and flower heads two years after field transplant. Plant and Soil 335: 311–323. DOI 10.1007/s11104-010-0417-z. [Google Scholar] [CrossRef]
Chappell J, Wolf F, Proulx J, Cuellar R, Saunders C (1995). Is the reaction catalyzed by 3-Hydroxy-3-Methylglutaryl coenzyme a reductase a rate-limiting step for isoprenoid biosynthesis in plants? Plant Physiology 109: 1337–1343. DOI 10.2307/4276936. [Google Scholar] [CrossRef]
Chaudhary V, Kapoor R, Bhatnagar AK (2008). Effectiveness of two arbuscular mycorrhizal fungi on concentrations of essential oil and artemisinin in three accessions of Artemisia annua L. Applied Soil Ecology 40: 174–181. DOI 10.1016/j.apsoil.2008.04.003. [Google Scholar] [CrossRef]
Chen J, Zhang H, Zhang X, Tang M (2017). Arbuscular mycorrhizal symbiosis alleviates salt stress in Black Locust through improved photosynthesis, water status, and K+/Na+ homeostasis. Frontiers in Plant Science 8: 1739. DOI 10.3389/fpls.2017.01739. [Google Scholar] [CrossRef]
Chen K, Shi SM, Yang XH, Huang XZ (2014). Contribution of arbuscular mycorrhizal inoculation to the growth and photosynthesis of mulberry in Karst rocky desertification area. Applied Mechanics and Materials 488–489: 769–773. DOI 10.4028/www.scientific.net/AMM.488-489.769. [Google Scholar] [CrossRef]
Chen ML, Guo LP, Yang G, Chen M, Yang L, Huang LQ (2011). Discussion on appraisal methods and key technologies of arbuscular mycorrhizal fungi and medicinal plant symbiosis system. China Journal of Chinese Materia Medica 36: 3051–3056. [Google Scholar]
Chen XY (2019). Determination of Alkaloids in Fritillaria Cirrhosa D. Don and Cloning and Expression of Key Enzyme Genes for Its Synthesis (MA Thesis). Chengdu University. https://kns.cnki.net/kcms/detail/detail.aspx?dbcode=CMFD&dbname=CMFD202001&filename=1019619263.nh&uniplatform=NZKPT&v=Va9EauKiuJlfadyTNlOVVP71XooAhiVV9Izcbz2YjelG-RMwOVNjY0xD7h0yxKu8. [Google Scholar]
Copetta A, Lingua G, Berta G (2006). Effects of three AM fungi on growth, distribution of glandular hairs, and essential oil production in Ocimum basilicum L. var. Genovese. Mycorrhiza 16: 485–494. DOI 10.1007/s00572-006-0065-6. [Google Scholar] [CrossRef]
Ding SL (2021). Mechanism of AM Fungi Improving the Tolerance of Grasses in Saline-Alkali Soil (MA Thesis). Inner Mongolia University. DOI 10.27224/d.cnki.gnmdu.2021.001258. [Google Scholar] [CrossRef]
Drigo B, Pijl AS, Duyts H, Kielak AM, Gamper HA et al. (2010). Shifting carbon flow from roots into associated microbial communities in response to elevated atmospheric CO2. Proceedings of the National Academy of Sciences 107: 10938–10942. DOI 10.1073/pnas.0912421107. [Google Scholar] [CrossRef]
Du L, Liu G, Lu J, Liu CS, Ha F (2014). Development of high-throughput sequencing technology and its application in bioscience. China Animal Husbandry & Veterinary Medicine 41: 109–116. [Google Scholar]
Estrada B, Aroca R, Maathuis FJM, Barea JM, Ruiz-Lozano JM (2013). Arbuscular mycorrhizal fungi native from a Mediterranean saline area enhance maize tolerance to salinity through improved ion homeostasis. Plant, Cell & Environment 36: 1771–1782. DOI 10.1111/pce.12082. [Google Scholar] [CrossRef]
Fan JH, Yang GT, Mu LQ, Zhou JH (2006). Effect of AMF on the content of berberine, jatrorrhizine and palmatine of phellodendron amurense seedlings. Protection Forest Science and Technology 5: 24–26. DOI 10.13601/j.issn.1005-5215.2006.05.010. [Google Scholar] [CrossRef]
Fotiadis D, Jenö P, Mini T, Wirtz S, Müller SA et al. (2001). Structural characterization of two aquaporins isolated from native spinach leaf plasma membranes. The Journal of Biological Chemistry 276: 1707–1714. DOI 10.1074/jbc.m009383200. [Google Scholar] [CrossRef]
Fu XF (2017). Diversity of Arbuscular Mycorrhizal Fungi in Rhizosphere Soil of Taxus chinensis var. Mairei and the Inoculation Effects of PSB and AMF on Host Plant (MA Thesis). Shanxi Normal University. https://kns.cnki.net/KCMS/detail/detail.aspx?dbname=CMFD201801&filename=1017279688.nh. [Google Scholar]
Ganesan V, Thomas G (2001). Salicylic acid response in rice: influence of salicylic acid on H2O2 accumulation and oxidative stress. Plant Sciences 160: 1095–1106. DOI 10.1016/S0168-9452(01)00327-2. [Google Scholar] [CrossRef]
Gao LL, Knogge W, Delp G, Smith FA, Smith SE (2004). Expression patterns of defense-related genes in different types of arbuscular mycorrhizal development in wild-type and mycorrhiza-defective mutant tomato. Molecular Plant-Microbe Interactions 17: 1103–1113. DOI 10.1094/mpmi.2004.17.10.1103. [Google Scholar] [CrossRef]
Gao LL, Smith FA, Smith SE (2006). The rmc locus does not affect plant interactions or defence-related gene expression when tomato (Solanum lycopersicum) is infected with the root fungal parasite. Rhizoctonia Functional Plant Biology: FPB 33: 289–296. DOI 10.1071/FP05153. [Google Scholar] [CrossRef]
Gerlach N, Schmitz J, Polatajko A, Schlüter U, Fahnenstich H et al. (2015). An integrated functional approach to dissect systemic responses in maize to arbuscular mycorrhizal symbiosis. Plant, Cell & Environment 38: 1591–1612. DOI 10.1111/pce.12508. [Google Scholar] [CrossRef]
Gu WC, Zhang J, Zhou N, Pan XJ, Ding B et al. (2020). Effect of different arbuscular mycorrhizal fungi combinations and inoculation periods on rhizosphere soil physicochemical properties and microbial quantity of Paris polyphylla var. yunnanensis seedlings. Chinese Journal of Experimental Traditional Medical Formulae 26: 116–130. DOI 10.13422/j.cnki.syfjx.20202072. [Google Scholar] [CrossRef]
He F, Zhang HQ, Tang M (2016). Aquaporin gene expression and physiological responses of Robinia pseudoacacia L. to the mycorrhizal fungus Rhizophagus irregularis and drought stress. Mycorrhiza 26: 311–323. DOI 10.1007/s00572-015-0670-3. [Google Scholar] [CrossRef]
Heijden MGA, Martin FM, Selosse MA, Sanders IR (2015). Mycorrhizal ecology and evolution: The past, the present, and the future. The New phytologist 205: 1406–1423. DOI 10.1111/nph.13288. [Google Scholar] [CrossRef]
He YJ, Zhong ZC, Liu JM, Liu JC, Song HX et al. (2007). Growth response of Broussonetia papyrifera seedlings to VA mycorrhizal fungi inoculation. Chinese Journal of Applied Ecology 10: 2209–2213. [Google Scholar]
Huang JH, Tan JF, Jie HK, Zeng RK (2011). Effects of inoculating arbuscular mycorrhizal fungi on Artemisia annua growth and its officinal components. Chinese Journal of Applied Ecology 22: 1443–1449. DOI 10.13287/j.1001-9332.2011.0211. [Google Scholar] [CrossRef]
Huang JH, Zeng RS, Luo SM, Gu WX, Nie CR et al. (2007). Antifungus activity of DIMBOA from Maize seedlings compared with several phenolic acids. Natural Product Research and Development 4: 572–577. DOI 10.16333/j.1001-6880.2007.04.008. [Google Scholar] [CrossRef]
Huang WL, Fan XJ, Yan ZY, Ma YT, Meng XL (2012). Diversity study of arbuscular mycorrhizal fungi of Coptis deltoidea. Journal of Chinese Medicinal Materials 35: 689–693. DOI 10.13863/j.issn1001-4454.2012.05.003. [Google Scholar] [CrossRef]
Hu WT (2017). A study on mechanisms of arbuscular mycorrhizal fungus regulating drought resistance and phosphorus metabolism of Lycium Barbarum under water stress. Northwest A&F University. https://kns.cnki.net/kcms/detail/detail.aspx?dbcode=CDFD&dbname=CDFDLAST2017filename&=1017101971.nh&uniplatform=NZKPT&v=BPYt0WguSNs0q7pkwpNAJVXymP_rBJSG8zMlhsSyFJeljNYORa946sRGZIkym4Sc. [Google Scholar]
Huang YP, Gan QX, Yang M, Qi JS, Pan XJ et al. (2020). Effect of field inoculation with arbuscular mycorrhizal fungi on rhizosphere soil structure of Paris polyphylla var. yunnanensis. Chinese Journal of Experimental Traditional Medical Formulae 26: 110–115. DOI 10.13422/j.cnki.syfjx.20202081. [Google Scholar] [CrossRef]
Isayenkov S, Mrosk C, Stenzel I, Strack D, Hause B (2005). Suppression of allene oxide cyclase in hairy roots of Medicago truncatula reduces jasmonate levels and the degree of mycorrhization with Glomus intraradices. Plant Physiology 139: 1401–1410. DOI 10.1104/pp.105.069054. [Google Scholar] [CrossRef]
Jia HM, Fang Q, Zhang SH, Yan ZY, Liu M (2020). Effects of AM fungi on growth and rhizosphere soil enzyme activities of Salvia miltiorrhiza. Acta Prataculturae Sinica 29: 83–92. [Google Scholar]
Jiang P (2012). The Resources and Species Diversity Research on AM Fungi occurring in Medicinal Plants of South Fujian (MA Thesis). Huaqiao University. https://kns.cnki.net/KCMS/detail/detail.aspx?dbname=CMFD201301&filename=1013001147.nh. [Google Scholar]
Jurkiewicz A, Ryszka P, Anielska T, Waligórski P, Białońska D et al. (2010). Optimization of culture conditions of Arnica montana L.: Effects of mycorrhizal fungi and competing plants. Mycorrhiza 20: 293–306. DOI 10.1007/s00572-009-0280-z. [Google Scholar] [CrossRef]
Kogel KH, Langen G (2005). Induced disease resistance and gene expression in cereals. Cellular Microbiology 7: 1555–1564. DOI 10.1111/j.1462-5822.2005.00592.x. [Google Scholar] [CrossRef]
Kong XS (2013). Screening of Symbiotic Genes between AM Fungi and Amorpha Fruticosa by Suppression Subtractive Hybridization (MA Thesis). Heilongjiang University. https://kns.cnki.net/KCMS/detail/detail.aspx?dbname=CMFD201602&filename=1016189992.nh. [Google Scholar]
Kováčik J, Klejdus B, Bačkor M (2009). Nitric oxide signals ROS scavenger-mediated enhancement of PAL activity in nitrogen-deficient Matricaria chamomilla roots: Side effects of scavengers. Free Radical Biology and Medicine 46: 1686–1693. DOI 10.1016/j.freeradbiomed.2009.03.020. [Google Scholar] [CrossRef]
Lanfranco L, Novero M, Bonfante P (2005). The mycorrhizal fungus Gigaspora margarita possesses a Cu/Zn superoxide dismutase that is upregulated during symbiosis with legume hosts. Plant Physiology 137: 1319–1330. DOI 10.1104/pp.104.050435. [Google Scholar] [CrossRef]
Liang XW, Yang Q, Li D, Cheng XX, Tang XM et al. (2017). Regulation of methyl jasmonate on secondary metabolism of Glycyrrhiza uralensis root. Guangdong Agricultural Sciences 44: 57–62. DOI 10.16768/j.issn.1004-874X.2017.06.008. [Google Scholar] [CrossRef]
Liu DJ, Tu B, Shi SM, Yang XH, Huang XZ et al. (2012). Research progress of the ecological crisis in rocky desertification area and the bioremediation potential of mycorrhizal mulberry. Carsologica Sinica 31: 185–190. [Google Scholar]
Liu JJ, Liu JL, Liu JH, Cui MM, Huang YJ et al. (2019a). The potassium transporter SlHAK10 is involved in mycorrhizal potassium uptake. Plant Physiology 180: 465–479. DOI 10.1104/pp.18.01533. [Google Scholar] [CrossRef]
Liu JN, Wu LJ, Wei SL, Xiao X, Su CX et al. (2007). Effects of arbuscular mycorrhizal fungi on the growth, nutrient uptake and glycyrrhizin production of licorice (Glycyrrhiza uralensis Fisch). Plant Growth Regulation 52: 29–39. DOI 10.1007/s10725-007-9174-2. [Google Scholar] [CrossRef]
Liu JQ, He RL, Ping LL, Wu JH, Li QS (2019b). Preliminary separation and identification of arbuscular mycorrhizal fungi from rhizosphere soil of Astragalus membranaceus in Shanxi. Journal of Shanxi College of Traditional Chinese Medicine 20: 337–339 343. DOI 10.19763/j.cnki.1671-0258.2019.05.008. [Google Scholar] [CrossRef]
Liu L (2020). Study on the Growth and Effective Medicinal Ingredient Accumulation of Pogostemon Cablin Induced by Arbuscular Mycorrhizal Fungi (MA Thesis). Guangxi University. DOI 10.27034/d.cnki.ggxiu.2020.002397. [Google Scholar] [CrossRef]
Liu M, Huang WL, Wang X, Wan RH, Wang H, Yan ZY (2017). Study on diversity of arbuscular mycorrhizal fungi in main producing areas of Salvia miltiorrhiza. China Journal of Chinese Materia Medica 42: 70–75. DOI 10.19540/j.cnki.cjcmm.20161222.039. [Google Scholar] [CrossRef]
Liu T (2017). Study on the Mechanism of Growth and the Content of Active Components of Clematis Intricata Bunge Regulated by AM Fungi (MA Thesis). Inner Mongolia Medical University. https://kns.cnki.net/KCMS/detail/detail.aspx?dbname=CMFD201801&filename=1017858702.nh. [Google Scholar]
Li HY (2002). The Mechanisms Involved in Plant Resistance/tolerance Response Induced by AM Fungi Towards Nematode (Ph.D. Thesis). Shandong Agricultural University. https://kns.cnki.net/KCMS/detail/detail.aspx?dbname=CDFD9908&filename=2002091068.nh. [Google Scholar]
Li HL, Xu LF, Li ZW, Zhao SX, Guo DQ et al. (2021). Mycorrhizas affect polyphyllin accumulation of Paris polyphylla var. yunnanensis through promoting PpSE expression. Phyton-International Journal of Experimental Botany 90: 1535–1547. DOI 10.32604/phyton.2021.015697. [Google Scholar] [CrossRef]
Li SJ, Wang JQ, Shi ZG, Zhao X, Li YR (2017). Effect of different base fertilizer treatments on maize soil enzyme activity and glomalin-related protein. Chinese Journal of Applied and Environmental Biology 23: 357–363. [Google Scholar]
Li T, Chen BD (2012). Arbuscular mycorrhizal fungi improving drought tolerance of maize plants by up-regulation of aquaporin gene expressions in roots and the fungi themselves. Chinese Journal of Plant Ecology 36: 973–981. [Google Scholar]
Li T, Zhao ZW (2005). Advances in researches on glomalin produced by arbuscular mycorrhizal fungi. Chinese Journal of Ecology 9: 1080–1084. [Google Scholar]
Li X, Zhang JL (2008). Contribution of arbuscular mycorrhizal fungi to N uptake by plants. Journal of Shanxi Datong University (Natural Science Edition) 24: 75–78. [Google Scholar]
Li Y, Yan JX, Chen XL (2019). Advances in research on the effects of AM fungi on plant pest control. Journal of Agricultural Biotechnology 27: 1692–1702. [Google Scholar]
Luo C, Wu T, Tan JY (2013). Diversity of arbuscular mycorrhizal fungi of Polygonum multiflorum. Guizhou Agricultural Sciences 41: 107–111. [Google Scholar]
Lushchak VI (2011). Adaptive response to oxidative stress: Bacteria, fungi, plants and animals. Comparative Biochemistry and Physiology Part C: Toxicology & Pharmacology 153: 175–190. DOI 10.1016/j.cbpc.2010.10.004. [Google Scholar] [CrossRef]
Maes L, Van Nieuwerburgh FC, Zhang YS, Reed DW, Pollier J et al. (2011). Dissection of the phytohormonal regulation of trichome formation and biosynthesis of the antimalarial compound artemisinin in Artemisia annua plants. The New Phytologist 189: 176–189. DOI 10.1111/j.1469-8137.2010.03466.x. [Google Scholar] [CrossRef]
Mandal S, Upadhyay S, Singh VP, Kapoor R (2015a). Enhanced production of steviol glycosides in mycorrhizal plants: A concerted effect of arbuscular mycorrhizal symbiosis on transcription of biosynthetic genes. Plant Physiology and Biochemistry 89: 100–106. DOI 10.1016/j.plaphy.2015.02.010. [Google Scholar] [CrossRef]
Mandal S, Upadhyay S, Wajid S, Ram M, Jain DC et al. (2015b). Arbuscular mycorrhiza increase artemisinin accumulation in Artemisia annua by higher expression of key biosynthesis genes via enhanced jasmonic acid levels. Mycorrhiza 25: 345–357. DOI 10.1007/s00572-014-0614-3. [Google Scholar] [CrossRef]
Mardis ER (2008). Next-generation DNA sequencing methods. Annual Review of Genomics and Human Genetics 9: 387–402. DOI 10.1146/annurev.genom.9.081307.164359. [Google Scholar] [CrossRef]
Ma ZB (2020). The Mechanism of Arbuscular Mycorrhizal Symbiosis Improving Salt Tolerance of Sour Jujube Seedlings (MA Thesis). Northwest A & F University. DOI 10.27409/d.cnki.gxbnu.2020.001555. [Google Scholar] [CrossRef]
Mbodj D, Effa-Effa B, Kane A, Manneh B, Gantet P et al. (2018). Arbuscular mycorrhizal symbiosis in rice: Establishment, environmental control and impact on plant growth and resistance to abiotic stresses. Rhizosphere 8: 3–26. DOI 10.1016/j.rhisph.2018.08.003. [Google Scholar] [CrossRef]
Mi YD, Li XR, Liu XM, Peng T, Wang W et al. (2020). Research advance of arbuscular mycorrhizal fungi diversity in Karst areas. Earth and Environment 48: 631–641. DOI 10.14050/j.cnki.1672-9250.2020.48.051. [Google Scholar] [CrossRef]
Ou H, Guo DQ, Lin JJ, Yan ZY, Teng Z et al. (2016). Effects of different AM fungi on quantity and enzyme activity of rhizosphere soil microorganism of Paris polyphylla var. yunnanensis. Journal of Chinese Medicinal Materials 39: 948–955. DOI 10.13863/j.issn1001-4454.2016.05.002. [Google Scholar] [CrossRef]
Parmita Z, Apurbo KR, Nurus SK, Nurul A, Tanzima Y (2008). Arbuscular mycorrhizal status of medicinal plants in rajshahi university campus. Mycosystema 27: 543–553. [Google Scholar]
Pérez-Tienda J, Testillano PS, Balestrini R, Fiorilli V, Azcón-Aguilar C et al. (2011). GintAMT2, a new member of the ammonium transporter family in the arbuscular mycorrhizal fungus glomus intraradices. Fungal Genetics and Biology 48: 1044–1055. DOI 10.1016/j.fgb.2011.08.003. [Google Scholar] [CrossRef]
Piszczek P, Kuszewska K, Błaszkowski J, Sochacka OA, Stojakowska A et al. (2019). Associations between root-inhabiting fungi and 40 species of medicinal plants with potential applications in the pharmaceutical and biotechnological industries. Applied Soil Ecology 137: 69–77. DOI 10.1016/j.apsoil.2019.01.018. [Google Scholar] [CrossRef]
Porcel R, Aroca R, Azcon R, Ruiz-Lozano JM (2006). PIP aquaporin gene expression in arbuscul armycorrhizal Glycine max and Lactuca sativa plants in relation to drought stress tolerance. Plant Molecular Biology 60: 389–404. DOI 10.1007/s11103-005-4210-y. [Google Scholar] [CrossRef]
Qi GH, Li BG, Guo SP, Yang WL, Lv GY (2006). Effects of arbuscular mycorrhizal fungi on water condition,activities of protective enzymes and peroxidation of membrane lipid of Diospyros lotus L. Journal of Agricultural University of Hebei 2: 22–25 41. [Google Scholar]
Qi GH, Zhang LP, Yang WL, Lu XR, Li CL (2002). Effects of arbuscular mycorrhizal fungi on growth and disease resistance of replanted Ginkgo (Ginkgo biloba L.) seedlings. Hebei Journal of Forestry and Orchard Research 1: 58–61. [Google Scholar]
Radhika KP, Rodrigues BF (2010). Arbuscular mycorrhizal fungal diversity in some commonly occurring medicinal plants of western ghats, goa region. Journal of Forestry Research 21: 45–52+120. DOI 10.1007/s11676-010-0007-1. [Google Scholar] [CrossRef]
Ran ZF, Yang XT, Li R, Zhou J, Zhang YQ et al. (2021). Diversity of arbuscular mycorrhizal fungi of Panax quinquefolius cultivated in Shandong province. China Journal of Chinese Materia Medica 46: 4103–4110. DOI 10.19540/j.cnki.cjcmm.20210523.107. [Google Scholar] [CrossRef]
Requena N, Mann P, Franken P (2000). A homologue of the cell cycle check point TOR2 from Saccharomyces cerevisiae exists in the arbuscular mycorrrhizal fungus Glomus mosseae. Protoplasma 212: 89–98. DOI 10.1007/BF01279350. [Google Scholar] [CrossRef]
Requena N, Serrano E, Ocón A, Breuninger M (2007). Plant signals and fungal perception during arbuscular mycorrhiza establishment. Phytochemistry 68: 33–40. DOI 10.1016/j.phytochem.2006.09.036. [Google Scholar] [CrossRef]
Rojas-Andrade R, Cerda-García-Rojas CM, Frías-Hernández JT, Dendooven L, Olalde-Portugal V et al. (2003). Changes in the concentration of trigonelline in a semi-arid leguminous plant (Prosopis laevigata) induced by an arbuscular mycorrhizal fungus during the presymbiotic phase. Mycorrhiza 13: 49–52. DOI 10.1007/s00572-002-0201-x. [Google Scholar] [CrossRef]
Schmidt K, Pflugmacher M, Klages S, Maser A, Mock A et al. (2008). Accumulation of the hormone abscisic acid (ABA) at the infection site of the fungus Cercospora beticola supports the role of ABA as a repressor of plant defence in sugar beet. Molecular Plant Pathology 9: 661–673. DOI 10.1111/j.1364-3703.2008.00491.x. [Google Scholar] [CrossRef]
Shang S, Wand P, Chen CY (2011). Signal recognition and transduction in the arbuscular mycorrhizal symbiosis. Plant Physiology Journal 47: 331–338. DOI 10.13592/j.cnki.ppj.2011.04.011. [Google Scholar] [CrossRef]
Shao YD, Hu XC, Wu QS, Yang TY, Srivastava AK et al. (2021). Mycorrhizas promote P acquisition of tea plants through changes in root morphology and P transporter gene expression. South African Journal of Botany 137: 455–462. DOI 10.1016/j.sajb.2020.11.028. [Google Scholar] [CrossRef]
Shi GX, Jiang SJ, Qin XX, Guo XQ, Ma SR et al. (2016). Effects of simulated grazing on arbuscular mycorrhizal fungi in alpine meadow ecosystem. Acta Agrestia Sinica 24: 610–617. [Google Scholar]
Shirzad K, Ahmad M (2020). Nitroxin and arbuscular mycorrhizal fungi alleviate negative effects of drought stress on Sorghum bicolor yield through improving physiological and biochemical characteristics. Plant Signaling & Behavior 15: 1813998. DOI 10.1080/15592324.2020.1813998. [Google Scholar] [CrossRef]
Shi XF, Huang JJ, Shi Y, Ding ZS, Cheng DQ (2017). On AMF diversity of Pinellia ternate. Journal of Shaanxi University of Chinese Medicine 40: 75–81. DOI 10.13424/j.cnki.jsctcm.2017.03.026. [Google Scholar] [CrossRef]
Soheila AHM, John CF, Jordan B, Thomas B (2001). Early signaling components in ultraviolet-B responses: distinct roles for different reactive oxygen species and nitric oxide. FEBS Letters 489: 237–242. DOI 10.1016/S0014-5793(01)02103-2. [Google Scholar] [CrossRef]
Song J, Han YY, Bai BX, Jin S, He QF et al. (2019). Diversity of arbuscular mycorrhizal fungi in rhizosphere soils of the Chinese medicinal herb Sophora flavescens Ait. Soil and Tillage Research 195: 104423. DOI 10.1016/j.still.2019.104423. [Google Scholar] [CrossRef]
Spoel SH, Dong X (2008). Making sense of hormone crosstalk during plant immune responses. Cell Host & Microbe 3: 348–351. DOI 10.1016/j.chom.2008.05.009. [Google Scholar] [CrossRef]
Sukhada M, Manjula R, Rawal RD (2011). Evaluation of arbuscular mycorrhiza and other biocontrol agents against Phytophthora parasitica var. nicotianae infecting papaya (Carica papaya cv. Surya) and enumeration of pathogen population using immunotechniques. Biological Control 58: 22–29. DOI 10.1016/j.biocontrol.2011.03.013. [Google Scholar] [CrossRef]
Sun PP (2014a). Functional Charaterization of Arginine Decarboxylase Gene Ptadc from Poncirus Trifolita and Indentification of the Regulators (Ph.D. Dissertation). Huazhong Agricultural University. https://kns.cnki.net/kcms/detail/detail.aspx?dbcode=CDFD&dbname=CDFDLAST2015&filename=1014213594.nh&uniplatform=NZKPT&v=LPdsJUJrY-gIHqSHEqj2CX3xlYBZTBty88ZzY9QyeHSw0FCTp2btLiwVueptrEFh. [Google Scholar]
Sun X (2014b). Studies on the Construction of Fritillaria Ussuriensis Maxim CAS Gene RNAi Vectors and Establish Genetic Transformation System (MA Thesis). Jilin Agricultural University. https://kns.cnki.net/KCMS/detail/detail.aspx?dbname=CMFD201501&filename=1014052993.nh. [Google Scholar]
Sun YC, Zheng XL, Liu XY, Chen X, Wang JW et al. (2013). Effects of inoculation with arbuscular mycorrhizal fungi on the growth of Glycyrrhiza uralensis Fisch in saline-alkaline soil. Journal of Anhui Agricultural Sciences 41: 10284–10285. DOI 10.13989/j.cnki.0517-6611.2013.25.062. [Google Scholar] [CrossRef]
Suzuki M, Muranaka T (2007). Molecular genetics of plant sterol backbone synthesis. Lipids 42: 47–54. DOI 10.1007/s11745-006-1000-5. [Google Scholar] [CrossRef]
Tang NW (2016). RNA-seq Based Gene Repertoire Survey and Symbiosis-Related Gene Analysis of the Arbuscular Mycorrhizal Fungus Gigaspora Rosea (Ph.D. Dissertation). Huazhong Agricultural University. https://kns.cnki.net/kcms/detail/detail.aspx?dbcode=CDFD&dbname=CDFDLAST2017&filename=1016156360.nh&uniplatform=NZKPT&v=fdzRJjuHnfsub34V2yN-_U_0LD_tA2VDqNPepTGF5o3-gRe7pi33FIWwupi28IZv. [Google Scholar]
Toussaint JP, Smith FA, Smith SE (2007). Arbuscular mycorrhizal fungi can induce the production of phytochemicals in sweet basil irrespective of phosphorus nutrition. Mycorrhiza 17: 291–297. DOI 10.1007/s00572-006-0104-3. [Google Scholar] [CrossRef]
Veresoglou SD, Rillig MC (2012). Suppression of fungal and nematode plant pathogens through arbuscular mycorrhizal fungi. Biology Letters 8: 214–217. DOI 10.1098/rsbl.2011.0874. [Google Scholar] [CrossRef]
Vieira LC, Silva DKA, Melo MAC, Escobar IEC, Oehl F et al. (2019). Edaphic factors influence the distribution of arbuscular mycorrhizal fungi along an altitudinal gradient of a tropical mountain. Microbial Ecology 78: 904–913. DOI 10.1007/s00248-019-01354-2. [Google Scholar] [CrossRef]
Wang FX, Xiao KZ, Jiang SF, Qu MY, Lian L et al. (2019). Mechanisms of reactive oxygen species in plants under drought stress. Chinese Science Bulletin 64: 1765–1779. DOI 10.1360/N972018-01116. [Google Scholar] [CrossRef]
Wang JW, Zheng LP, Wu JY, Tan RX (2006). Involvement of nitric oxide in oxidative burst, phenylalanine ammonia-lyase activation and Taxol production induced by low-energy ultrasound in Taxus yunnanensis cell suspension cultures. Nitric Oxide 15: 351–358. DOI 10.1016/j.niox.2006.04.261. [Google Scholar] [CrossRef]
Wang L (2013). The Resources Diversity of AM Fungi and the Relationship with Soil Carbon and Nitrogen in the Rhizosphere of Dendranthema morifolium (MA Thesis). Hebei University. https://kns.cnki.net/KCMS/detail/detail.aspx?dbname=CMFD201402&filename=1013033194.nh. [Google Scholar]
Wang LJ, Fang X, Yang CQ, Li JX, Chen XY (2013). Biosynthesis and regulation of secondary terpenoid metabolism in plants. Scientia Sinica 43: 1030–1046. DOI 10.1360/052013-307. [Google Scholar] [CrossRef]
Wang XF, Zhang X, Zhao RH, Yu J, Gu W et al. (2020). Effect and mechanism of arbuscular mycorrhizal fungi in herbs. Chinese Journal of Experimental Traditional Medical Formulae 26: 217–226. DOI 10.13422/j.cnki.syfjx.20200811. [Google Scholar] [CrossRef]
Wang XK, Guo SX, Li M, Zhao HH (2012). Molecular mechanisms of improving plant disease resistance by arbuscular mycorrhizal fungi. Journal of Qingdao Agricultural University (Natural Science) 29: 170–175. [Google Scholar]
Wang YS, Liu RJ (2017). A checklist of arbuscular mycorrhizal fungi in the recent taxonomic system of Glomeromycota. Mycosystema 36: 820–850. DOI 10.13346/j.mycosystema.170078. [Google Scholar] [CrossRef]
Wang YY (2020). The mechanism of arbuscular mycorrhizal fungi and potassium regulating salt tolerance and Potassium absorption of Lycium barbarum. Northwest A&F University. DOI 10.27409/d.cnki.gxbnu.2020.000588. [Google Scholar] [CrossRef]
Wei XY (2010). Preliminary Research on Arbuscular Mycorrhizas in Rhizosphere Soil of Paeonia suffruticosa (MA Thesis). Anhui Normal University. https://kns.cnki.net/kcms/detail/detail.aspx?dbcode=CMFD&dbname=CMFD2012&filename=1011041307.nh&uniplatform=. [Google Scholar]
Wei ZC, Zhao SX, Li ZW, Zhao JJ, Zhou N et al. (2021). Effects of mixed inoculation of different arbuscular mycorrhizal fungi on Saussurea costus rhizosphere microorganisms and soil enzyme activities. Chinese Wild Plant Resources 40: 6–11, 26. [Google Scholar]
Xiao WJ, Yang G, Chen ML, Guo LP, Wang M (2011). AM and its application in plant disease prevention of Chinese medicinal herbs cultivation. China Journal of Chinese Materia Medica 36: 252–257. [Google Scholar]
Xie LJ, Song YY, Zeng RS, Wang RL, Wei XC et al. (2012). Disease resistance signal transfer between roots of different tomato plants through common arbuscular mycorrhiza networks. Chinese Journal of Applied Ecology 23: 1145–1152. DOI 10.13287/j.1001-9332.2012.0189. [Google Scholar] [CrossRef]
Xie W, Hao ZP, Guo LP, Zhang X, Zhang SB et al. (2020). Research advances in terpenoids synthesis and accumulation in plants as influenced by arbuscular mycorrhizal symbiosis. Biotechnology Bulletin 36: 49–63. DOI 10.13560/j.cnki.biotech.bull.1985.2020-0117. [Google Scholar] [CrossRef]
Xing D, Wang ZH, Shen G, Han SY, Luo CB et al. (2019a). Promoting mechanism of arbuscular mycorrhizal fungi on plants water absorption and utilization in Karst area. World Forestry Research 32: 24–29. DOI 10.13348/j.cnki.sjlyyj.2018.0095.y. [Google Scholar] [CrossRef]
Xing D, Han SY, Luo CB, Yang ST, Zhang F et al. (2019b). Effects of arbuscular mycorrhizal fungi on Mulberry growth and water use efficiency under drought condition. Science of Sericulture 45: 475–483. DOI 10.13441/j.cnki.cykx.2019.04.002. [Google Scholar] [CrossRef]
Xue YL, Li CY, Wang CR, Wang Y, Liu J et al. (2019). Mechanisms of phosphorus uptake from soils by arbuscular mycorrhizal fungi. Journal of Soil and Water Conservation 33: 10–20. DOI 10.13870/j.cnki.stbcxb.2019.06.002. [Google Scholar] [CrossRef]
Xu K (2012). Ecological Distribution of Arbuscular Mycorrhizal Fungi and Glomalin in the Rhizosphere of Scutellaria baicalensis (MA Thesis). Hebei University. https://kns.cnki.net/KCMS/detail/detail.aspx?dbname=CMFD201502&filename=1012028470.nh. [Google Scholar]
Xu LJ, Jiang XL, Hao ZP, Li T, Wu ZX et al. (2017). Arbuscular mycorrhiza improves plant adaptation to phosphorus deficiency through regulating the expression of genes relevant to carbon and phosphorus metabolism. Chinese Journal of Plant Ecology 41: 815–825. [Google Scholar]
Yang AN, Lu L, Wu CX, Xia MM (2011a). Arbuscular mycorrhizal fungi associated with Huangshan Magnolia (Magnolia cylindrica). Journal of Medicinal Plants Research 5: 4542–4548. DOI 10.5897/JMPR.9000262. [Google Scholar] [CrossRef]
Yang J, He XL, Zhao LL (2011b). Species diversity of arbuscular mycorrhizal fungi in the rhizosphere of Salix psammophila in inner Mongolia desert. Biodiversity Science 19: 377–385. [Google Scholar]
Yang L (2012). Effects of Arbuscular Mycorrhizal Fungi on Resistance to Root Disease by Salvia Miltiorrhiza and Related Mechanisms (MA Thesis). Southwest Jiaotong University. https://kns.cnki.net/KCMS/detail/detail.aspx?dbname=CMFD2012&filename=1012392499.nh. [Google Scholar]
Yang M, Zhang J, Zhang DQ, He J, Qi JS et al. (2018). Effect of arbuscular mycorrhizal on yield and secondary metabolites of Rheum palmatum. Chinese Journal of Experimental Traditional Medical Formulae 24: 33–37. DOI 10.13422/j.cnki.syfjx.20181007. [Google Scholar] [CrossRef]
Yang RY, Deng QZ, Tian LP, Tian JW, Qiu CS et al. (2022). Diversity of arbuscular mycorrhizal fungi in the rhizosphere of Ophiopogon japonicus in different habitats. Acta Botanica Boreali-Occidentalia Sinica 42: 145–153. DOI 10.7606/j.issn.1000-4025.2022.01.0145. [Google Scholar] [CrossRef]
Yang ZY, Liao SX (2005). Advances in the research on the effects of arbuscular mycorrhizal fungi on plant stress resistance. World Forestry Research 2: 26–29. DOI 10.13348/j.cnki.sjlyyj.2005.02.006. [Google Scholar] [CrossRef]
Ye JS, Li T, Hu YJ, Hao ZP, Gao YZ et al. (2013). Influences of AM fungi on plant growth and water-stable soil aggregates under drought stresses. Acta Ecologica Sinica 33: 1080–1090. DOI 10.5846/stxb. [Google Scholar] [CrossRef]
Yu Z, Liang KN, Huang GH, Yang G, Zhou ZZ et al. (2021). Research progress on the mechanisms of arbuscular mycorrhizal fungi on drought resistance in plants. Pratacultural Science 4: 640–653. [Google Scholar]
Yu Y, Yu T, Wang Y, Yan XF (2012). Effect of CO-cultivation time on camptothecin content in Camptotheca acuminata seedlings after inoculation with arbuscular mycorrhizal fungi. Acta Ecologica Sinica 32: 1370–1377. [Google Scholar]
Zeng Y, Guo LP, Sun YZ, Zhou J (2007). AM and its application in TCM cultivation. World Science and Technology-Modernization of Traditional Chinese Medicine and Materia Medica 6: 83–87. [Google Scholar]
Zeng Y, Guo LP, Wang JY, Cui XM, Yang G et al. (2015). Relationship between intensity of the mycorrhizal colonisation in root system and biomass or saponins content of Panax notoginseng. Modern Chinese Medicine 17: 1253–1257. DOI 10.13313/j.issn.1673-4890.2015.12.006. [Google Scholar] [CrossRef]
Zhang CB, Tan JY, Luo C (2013a). Arbuscular mycorrhizal fungal diversity of Rosa laevigata Michx. in Karst areas. Hubei Agricultural Sciences 52: 2044–2047. DOI 10.14088/j.cnki.issn0439-8114.2013.09.046. [Google Scholar] [CrossRef]
Zhang F, Zou YN, Wu QS (2019a). Effects of Funneliformis mosseae on the expression of antioxidant enzyme genes in trifoliate orange exposed to drought stress. Mycosystema 38: 2043–2050. DOI 10.13346/j.mycosystema.190199. [Google Scholar] [CrossRef]
Zhang HQ, Wei SZ, Hu WT, Xiao LM, Tang M (2017a). Arbuscular mycorrhizal fungus rhizophagus irregularis increased potassium content and expression of genes encoding potassium channels in Lycium barbarum. Frontiers in Plant Science 8: 440. DOI 10.3389/fpls.2017.00440. [Google Scholar] [CrossRef]
Zhang H, Sun JQ, Bao YY (2015). Advances in studies on plant secondary metabolites influenced by arbuscular mycorrhizal fungi. Journal of Agricultural Biotechnology 23: 1093–1103. DOI 10.3969/j.issn.1674-7968.2015.08.013. [Google Scholar] [CrossRef]
Zhang JH, Feng BB, Wu CS (2020). Effects of arbuscular mycorrhizal fungi on growth and q-markers of Fritillaria Taipaiensis. Chinese Journal of Information on TCM 27: 88–93. [Google Scholar]
Zhang J, Liu DH, Guo LP, Jin H, Yang G et al. (2011). Effects of arbuscular mycorrhizae fungi on biomass and essential oil in rhizome of Atractylodes lancea in different temperatures. Chinese Traditional and Herbal Drugs 42: 372–375. [Google Scholar]
Zhang J, Pan XJ, Luo J, Zhou N, Xie E et al. (2017b). Effects of arbuscular mycorrhizal fungi on the yield and contents of costunolide and dehydrocostuslactone in root of Saussurea costus. China Pharmacy 28: 2179–2182. [Google Scholar]
Zhang RQ, Zhao HQ, Zhu HH, Yao Q (2010). Research progress in the biosynthesis of phenols in plants induced by arbuscular mycorrhizal fungi. Microbiology China 37: 1216–1221. DOI 10.13344/j.microbiol.china.2010.08.007. [Google Scholar] [CrossRef]
Zhang RQ, Zhu HH, Zhao HQ, Yao Q (2013b). Arbuscular mycorrhizal fungal inoculation increases phenolic synthesis in clover roots via hydrogen peroxide, salicylic acid and nitric oxide signaling pathways. Journal of Plant Physiology 170: 74–79. DOI 10.1016/j.jplph.2012.08.022. [Google Scholar] [CrossRef]
Zhang X (2021). Study on the Effects of AMF Colonization on the Accumulation of Polygonatum Kingianum Functional Components under Different Phosphate Levels and its Regulation Mechanism (MA Thesis). Yunnan University of Chinese Medicine DOI 10.27460/d.cnki.gyzyc.2021.000212. [Google Scholar] [CrossRef]
Zhang X, Guo YN, Li YD, Wang WB, Zheng YY et al. (2019b). Spatial and temporal distribution of arbuscular mycorrhiza fungi and dark septate endophytes in Hedysarum scoparium from northwest desert belt. Mycosystema 38: 2030–2042. DOI 10.13346/j.mycosystema.190220. [Google Scholar] [CrossRef]
Zhang ZF, Zhang JC, Huang YQ, Xu GP, Zhang DN, Yu YC (2016). Effects of mycorrhizal fungi on the drought tolerance of Cyclobalanopsis glauca seellings. Acta Ecologica Sinica 36: 3402–3410. [Google Scholar]
Zhao F (2021). The Mechanism of Arbuscular Mycorrhizal Fungi Regulating Nitrogen Absorbption of Lycium Barbarum (MA Thesis). Northwest A & F University DOI 10.27409/d.cnki.gxbnu.2021.001528. [Google Scholar] [CrossRef]
Zhao JL, He XL (2011). Effects of AM fungi on drought resistance and content of chemical components in Angelica dahurica. Acta Agriculturae Boreali-Occidentalis Sinica 20: 184–189. [Google Scholar]
Zhao WZ, Cheng GD (2001). Mycorrhizae and its application in desertification land restoration. Chinese Journal of Applied Ecology 6: 947–950. [Google Scholar]
Zhao X, Wang BW, Yan XF (2006). Effect of arbuscular mycorrhiza on camptothecin content in Camptotheca acuminata seedlings. Acta Ecologica Sinica 4: 1057–1062. [Google Scholar]
Zheng YR (2021). Diversity of Arbuscular Mycorrhizal Fungi (AMF) in Mulberry Rhizosphere and its Effect on Salt Tolerance of Hosts (MA Thesis). Northwest A&F University DOI 10.27409/d.cnki.gxbnu.2021.001672. [Google Scholar] [CrossRef]
Zhou JH, Fan JH (2007). Effects of AM fungi on the berberine content in Phellodendron chinense seedlings. Northern Horticulture 12: 25–27. [Google Scholar]
Zhou Y, Yang M, Guo DQ, Pan XJ, Ding B et al. (2020). Effect of different exogenous arbuscular mycorrhizal fungi combinations on rhizosphere soil environment of Paris polyphylla var. yunnanensis. Chinese Journal of Experimental Traditional Medical Formulae 26: 96–109. DOI 10.13422/j.cnki.syfjx.20202080. [Google Scholar] [CrossRef]
Zhou ZQ, Hu YN, Peng YL, Sun ML, Zhang YH et al. (2015). Effects of three arbuscular mycorrhizas on different provenances of Amur Cork seedings. Bulletin of Botanical Research 35: 92–100. DOI CNKI:SUN:MBZW.0.2015-01-017. [Google Scholar]
Zhu XC, Song FB, Liu SQ, Liu TD (2011). Effects of arbuscular mycorrhizal fungus on photosynthesis and water status of maize under high temperature stress. Plant and Soil 346: 189–199. DOI 10.1007/s11104-011-0809-8. [Google Scholar] [CrossRef]
Zou YN, Wu HH, Giri B, Wu QS, Kuča K (2019). Mycorrhizal symbiosis down-regulates or does not change root aquaporin expression in trifoliate orange under drought stress. Plant Physiology and Biochemistry 144: 292–299. DOI 10.1016/j.plaphy.2019.10.001. [Google Scholar] [CrossRef]
Zou YN, Wu QS, Huang YM, Ni QD, He XH (2013). Mycorrhizal-mediated lower proline accumulation in Poncirus trifoliata under water deficit derives from the integration of inhibition of proline synthesis with increase of proline degradation. PLoS One 8: 1–8. DOI 10.1371/journal.pone.0080568. [Google Scholar] [CrossRef]
Zubek S, Błaszkowski J (2009). Medicinal plants as hosts of arbuscular mycorrhizal fungi and dark septate endophytes. Phytochemistry Reviews 8: 571–580. DOI 10.1007/s11101-009-9135-7. [Google Scholar] [CrossRef]
Zubek S, Mielcarek S, Turnau K (2012). Hypericin and pseudohypericin concentrations of a valuable medicinal plant Hypericum perforatum L. are enhanced by arbuscular mycorrhizal fungi. Mycorrhiza 22: 149–156. DOI 10.1007/s00572-011-0391-1. [Google Scholar] [CrossRef]
Zubek S, Stojakowska A, Anielska T, Turnau K (2010). Arbuscular mycorrhizal fungi alter thymol derivative contents of Inula ensifolia L. Mycorrhiza 20: 497–504. DOI 10.1007/s00572-010-0306-6. [Google Scholar] [CrossRef]
Cite This Article
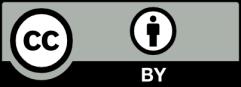
This work is licensed under a Creative Commons Attribution 4.0 International License , which permits unrestricted use, distribution, and reproduction in any medium, provided the original work is properly cited.