Open Access
VIEWPOINT
Impact of SARS-CoV-2 infection during pregnancy on postnatal brain development: The potential role of glial cells
1 Programa de Pós-Graduação em Neurociências, Instituto de Ciências Básicas da Saúde, Universidade Federal do Rio Grande do Sul, Porto Alegre, 90050170, Brazil
2 Departamento de Bioquímica, Instituto de Ciências Básicas da Saúde, Universidade Federal do Rio Grande do Sul, Porto Alegre, 90035003, Brazil
3 Faculdade de Farmácia, Universidade Federal do Rio Grande do Sul, Porto Alegre, 90610000, Brazil
4 Hospital de Clínicas de Porto Alegre, Porto Alegre, 90035903, Brazil
5 Programa de Pós-Graduação em Biologia Celular e Molecular, Centro de Biotecnologia, Universidade Federal do Rio Grande do Sul, Porto Alegre, 91501970, Brazil
6 Programa de Pós-Graduação em Ciências Biológicas: Bioquímica, Instituto de Ciências Básicas da Saúde, Universidade Federal do Rio Grande do Sul, Porto Alegre, 90035003, Brazil
* Corresponding Author: LARISSA DANIELE BOBERMIN. Email:
BIOCELL 2022, 46(12), 2517-2523. https://doi.org/10.32604/biocell.2022.021566
Received 21 January 2022; Accepted 18 April 2022; Issue published 10 August 2022
Abstract
Glial cells are crucial for maintaining central nervous system (CNS) homeostasis. They actively participate in immune responses, as well as form functional barriers, such as blood-brain barrier (BBB), which restrict the entry of pathogens and inflammatory mediators into the CNS. In general, viral infections during the gestational period can alter the embryonic and fetal environment, and the related inflammatory response may affect neurodevelopment and lead to behavioral dysfunction during later stage of life, as highlighted by our group for Zika virus infection. Severe acute respiratory syndrome coronavirus-2 (SARS-CoV-2) induces a cytokine storm and, during pregnancy, may be related to a more severe form of the coronavirus disease-19 (COVID-19) and also to higher preterm birth rates. SARS-CoV-2 can also affect the CNS by inducing neurochemical remodeling in neural cells, which can compromise neuronal plasticity and synaptic function. However, the impact of SARS-CoV-2 infection during pregnancy on postnatal CNS, including brain development during childhood and adulthood, remains undetermined. Our group has recently highlighted the impact of COVID-19 on the expression of molecular markers associated with neuropsychiatric disorders, which are strongly related to the inflammatory response. Thus, based on these relationships, we discussed the impact of SARS-CoV-2 infection either during pregnancy or in critical periods of neurodevelopment as a risk factor for neurological consequences in the offspring later in life, focusing on the potential role of glial cells. Thus, it is important to consider future and long-term public health concerns associated with SARS-CoV-2 infection during pregnancy.Keywords
New public health concerns have been raised by the severe acute respiratory syndrome coronavirus-2 (SARS-CoV-2) infection in pregnant women, which may be related to the development of a more severe form of the coronavirus disease-19 (COVID-19), gestational complications, and higher preterm birth rates (Beys-da-Silva et al., 2020; Celewicz et al., 2021; Karasek et al., 2021). However, the impact of COVID-19 during pregnancy on postnatal development, particularly development of the central nervous system (CNS), including during childhood and adulthood, remains undetermined. In general, viral infections during the gestational period can alter the embryonic and fetal environment, and the related inflammatory response may affect neurodevelopment and lead to subsequent behavioral dysfunctions (Zimmer et al., 2021). While relevant evidence has suggested vertical transmission of SARS-CoV-2 infection, pro-inflammatory mediators involved in the maternal cytokine storm may also pass through the placenta, resulting in an indirect inflammatory response in the fetus (Joma et al., 2021; Vivanti et al., 2020).
Neurological complications have also been increasingly reported in individuals with COVID-19, which may be due to either direct viral entry into the CNS or a systemic cytokine storm (Doyle, 2021; Solomon, 2021). Angiotensin-converting enzyme 2 (ACE-2) is expressed in glial cells and neurons, which supports the potential neurotropism of SARS-CoV-2 (Hernández et al., 2021). Moreover, glial cells, particularly microglia and astrocytes, perform immune and inflammatory functions that allow them to respond to both viral infection and systemic inflammation (Liddelow et al., 2020; Tremblay et al., 2020). Importantly, glial cells also play crucial roles in neurodevelopment. In this context, the dysfunction of these cells during the fetal and postnatal periods may be related to neuropsychiatric disorders such as autism spectrum disorder (ASD) and schizophrenia, later in life (Dietz et al., 2020; Scuderi and Verkhratsky, 2020). In addition, TORCH (an acronym used to refer to toxoplasmosis, rubella, cytomegalovirus, herpes simplex) congenital syndromes have been associated with several neurological complications (Megli and Coyne, 2021). In this article, we highlight the potential consequences of SARS-CoV-2 infection during pregnancy on brain development.
Immune, homeostatic, and neurodevelopmental roles of glial cells
Glial cells can be subdivided into macroglia (astrocytes and oligodendrocytes) and microglia, which are essential for brain development and homeostasis (Zuchero and Barres, 2015). Although microglial cells are resident immune cells in the CNS, astrocytes actively participate in innate immunity and inflammatory responses (Colombo and Farina, 2016). Both microglia and astrocytes synthesize and release several cytokines and chemokines that can propagate inflammation within the CNS (Liddelow et al., 2020). Astrocytes possess a specific cytoarchitecture that allows intimate contact with blood vessels, synapses, and other glial cells, which enables them to sense their surroundings and dynamically respond to changes in their microenvironment. They form functional barriers, such as the blood-brain barrier (BBB), which restricts the entry of pathogens and inflammatory mediators into the CNS (Greenhalgh et al., 2020). It is noteworthy that peripheral immune cells can affect the CNS when BBB permeability is compromised, and that astrocytes can guide inflammatory processes under healthy as well as diseased and injured conditions. Moreover, astrocytes regulate neurotransmitter systems, metabolic activity, and synthesis and release neurotrophic factors (Quincozes-Santos et al., 2021b).
Macroglia are derived from radial glial cells that have a neuroepithelial origin and are pluripotent neural cell precursors (Zuchero and Barres, 2015). Microglial cells have a distinct (mesodermal) origin from the embryonic yolk sac and infiltrate the developing neural tube (Ginhoux and Prinz, 2015). The proliferation of glial and neuronal cells peaks during fetal development, between the 9th and 16th week post-conception. This means that glial cells are present during most brain development processes, including neurogenesis, neuronal circuit building during prenatal periods, and synaptic pruning in postnatal stages (Ziats et al., 2015). While glial cells develop, proliferate, differentiate, and acquire functional properties, they establish important crosstalk with other glial cell types and neurons, which is continuously required not only during development but also in the mature brain. This communication occurs mainly via secreted molecules, including trophic and growth factors, cytokines, chemokines, neurotransmitters, and hormones (Han et al., 2021; Tay et al., 2017).
During development, astrocytes are essential for synaptogenesis, production and delivery of glutamate to neurons, extracellular potassium buffering, and metabolic support, and can respond to neuronal activity. Thus, they display a wide range of homeostatic functions that are necessary for synapse formation and maintenance (Zuchero and Barres, 2015). Microglia are necessary for neurogenesis and neuronal migration, orchestrating the assembly of prenatal neuronal circuits (Colonna and Butovsky, 2017). During postnatal development, both microglia and astrocytes are responsible for tagging and eliminating redundant neurons that do not form functional neuronal circuits, a process known as synaptic pruning (Colonna and Butovsky, 2017).
Glial cells are at the center of CNS homeostasis and respond to damage. Disturbances in glial morphology, gene expression, and functions have been reported in a growing number of neurodevelopmental and neuropsychiatric disorders, such as ASD and schizophrenia (Bristot Silvestrin et al., 2013; Dietz et al., 2020; Gottfried et al., 2015). While the precise time of onset and cellular neurobiology of these disorders remain poorly understood, the role of glial cells in these disorders has gained evidence. Different mechanisms have been proposed to link aberrant glial development/activity during gestational and postnatal periods to ASD and schizophrenia. Neuroinflammation is guided by morphological and functional changes in microglia and astrocytes. In this context, in utero exposure to pathogens and other neurotoxic environmental factors that cause neuroinflammation in the developing brain has emerged as an etiological factor for these disorders (Gottfried et al., 2015; Scuderi and Verkhratsky, 2020). Moreover, non-inflammatory mechanisms may involve defects in synaptogenesis or synaptic pruning, disruption of glutamate metabolism, potassium homeostasis, secretion of neurotrophic factors, and abnormal glial coverage and synaptic support (Ballas et al., 2009; Dietz et al., 2020; Maezawa and Jin, 2010). All of these glial cell-associated failures may result in dysregulated synaptic transmission and/or a lack of functional neurons.
Maternal infections and later neurological consequences in the offspring: potential roles of glial cells
An important connection between maternal infections during pregnancy and congenital syndromes and/or later neuropsychiatric disorders has been established using epidemiological and experimental animal models (Estes and McAllister, 2016; Knuesel et al., 2014). Gestational transmission can occur during the prenatal period through the transplacental hematogenous route, during the perinatal period through contact with blood and vaginal secretions from the mother, and during the postnatal period through breast milk (Neu et al., 2015). Importantly, although evidence of infection can be observed at birth and/or in childhood, it is possible that certain symptoms might manifest only in adulthood.
TORCH refers to congenital syndromes with clinical similarities, namely infections caused by Toxoplasma gondii, rubella, cytomegalovirus (CMV), and herpes simplex (HSV) (Megli and Coyne, 2021). The common clinical manifestations of TORCH congenital syndromes include microcephaly, cerebral calcifications, hydrocephalus, motor and brain dysfunction, sensorineural hearing loss, and intellectual disability (Megli and Coyne, 2021). CMV infection has been shown to alter the proliferation, migration, and differentiation of neural precursor cells, including astrocytes, which may explain the abnormalities in brain development observed in congenital infections (Odeberg et al., 2007). Amendments in the term TORCH, such as TORCHES or STORCH, have been proposed to include syphilis, which is caused by Treponema pallidum (Kinney and Kumar, 1988). More recently, due to the severity of congenital infection, Zika virus (ZIKV) infection has also been proposed to be included as a new member, as TORCHZ or STORCHZ (Coyne and Lazear, 2016).
Although ZIKV infection during pregnancy is mainly associated with microcephaly, it has recently been correlated with ASD diagnosis in childhood (Santi et al., 2021; Vianna et al., 2018). These neurodevelopmental abnormalities may be associated with neuroimmune changes caused by the release of inflammatory mediators by ZIKV-infected neural cells (Bobermin et al., 2020). Moreover, fetal human astrocytes can be used as a viral reservoir, contributing to chronic brain infections (Limonta et al., 2018). Other viruses, such as rubella virus and influenza, can also target glial cells and interfere with neuron-glia communication, resulting in aberrant neuronal migration, synaptogenesis, and synaptic pruning associated with ASD-like behaviors and an increased risk for schizophrenia (Fatemi et al., 2008; Scuderi and Verkhratsky, 2020). It is important to note that several neuropsychiatric disorders, other than STORCHZ, have also been reported to be associated with morphofunctional changes.
Of note, both direct infection by viruses in the fetal CNS and maternal immune responses may be critical for these neurodevelopmental and/or neuropsychiatric sequelae. Activation of Toll-like receptor (TLR) pathways (including TLR3) in host immune cells, which may include glial cells, induces the expression of inflammatory cytokines and interferons through nuclear factor κB (NFκB) signaling (Alexopoulou et al., 2001; Greenhalgh et al., 2020). In this context, pro-inflammatory cytokines such as interleukins (IL-6, IL-1β, IL-17) and tumor necrosis factor a (TNF-α) have been described in animal models as key signaling molecules that predispose the individual to neurodevelopmental and neuropsychiatric disorders (Favrais et al., 2011; Gilmore et al., 2005).
Current evidence on SARS-CoV-2 vertical transmission
Relevant evidence has raised the possibility of SARS-CoV-2 vertical transmission, either during the antepartum, intrapartum, or postpartum periods. ACE-2 expression in the placenta and detection of SARS-CoV-2 in the placenta and amniotic fluid suggest that transplacental transmission can occur (Hosier et al., 2020; Li et al., 2020; Vivanti et al., 2020). Pathological findings have also shown increased inflammatory infiltration in the placentas of SARS-CoV-2-infected pregnant women (Schoenmakers et al., 2021). Notably, the placenta may become more susceptible to SARS-CoV-2 infection when it is inflamed (Lye et al., 2020). Moreover, SARS-CoV-2 has been detected in the umbilical cord, vaginal mucosa, and milk (Fenizia et al., 2020).
In neonates born from SARS-CoV-2-infected women, severe respiratory symptoms are not common, although there are some cases of respiratory diseases, abnormal Apgar indexes, and pneumonia (Nayak et al., 2020; Wu et al., 2020). However, a case report documented viremia and neurological symptoms in a newborn, which, in addition to supporting the transplacental transmission of SARS-CoV-2, suggested the effects of SARS-CoV-2 infection in the immature brain with evidence of gliosis (Vivanti et al., 2020).
Neurological symptoms associated with SARS-CoV-2 infection: Potential role of glial cells and possible implications in neurodevelopment
Neurological manifestations are increasingly common in COVID-19 adult patients in addition to some degree of encephalitis, which could indicate substantial damage to the CNS (Doyle, 2021; Moriguchi et al., 2020). Moreover, the development of neurological symptoms in children with COVID-19 has been described (Abdel-Mannan et al., 2020). Although neurotropism and the mechanisms associated with SARS-CoV-2 entry into the CNS are still under investigation, it is important to note that other coronaviruses can compromise the nervous system by directly targeting neural cells and inducing a significant inflammatory condition that can lead to neuronal death (Li et al., 2016).
In line with this, it has been demonstrated that the main cells of the CNS, such as neurons, astrocytes, and microglia, may be direct targets of SARS-CoV-2 owing to the expression of ACE-2 (Cama et al., 2021; Crunfli et al., 2020; Song et al., 2021). In particular, astrocytes have been shown to be permissive to SARS-CoV-2, serving as a potential site for replication of the virus in the CNS. Moreover, astrocytes undergo several functional alterations after infection, including metabolic remodeling, which can compromise neuronal plasticity and synaptic functions (Crunfli et al., 2020). Therefore, astrocyte dysfunction may be a mechanism underlying the short-and long-term neurological consequences of SARS-CoV-2 infection.
Reactive astrogliosis has also been observed in post-mortem brains of COVID-19 patients, along with microglial activation and infiltration of T lymphocytes, reinforcing the presence of an inflammatory process (Matschke et al., 2020). Notably, both astrocytes and microglia are sensitive to peripheral cytokine storms, which can disrupt the BBB and facilitate viral entry into the CNS (Greenhalgh et al., 2020; Huang et al., 2021). Importantly, glial inflammatory phenotypes may contribute to various brain dysfunctions, including neurodegenerative diseases, neuropsychiatric symptoms, and neurodevelopmental disorders (Garden and Campbell, 2016; Pantelis et al., 2020).
The cytokine storm caused by SARS-CoV-2 may be an aggravating factor in pregnant women, since maternal pro-inflammatory mediators can be transferred across the placenta and subsequently reach the developing CNS (Joma et al., 2021; Reyes-Lagos et al., 2021). In this regard, high levels of important inflammatory markers, including IL-6, IL-17, and TNF-α, have been reported in the blood of pregnant women with COVID-19 and the umbilical cord, as well as in the serum of neonates born to infected mothers (Joma et al., 2021; Xiao et al., 2020; Zhu et al., 2020). Among them, the role of IL-6 in maternal systemic inflammation during fetal brain development has been particularly studied. In an animal model, maternal treatment with IL-6 alone was sufficient to predispose the offspring to neuropsychiatric disorders such as schizophrenia and autism (Smith et al., 2007). Interestingly, inhibition of IL-6 signaling through its placental receptors prevented increased IL-6 expression in the fetal brain and neurodevelopmental abnormalities (Wu et al., 2017). Moreover, studies in humans have shown architectural and functional brain alterations in children exposed to increased levels of IL-6 during pregnancy (Rasmussen et al., 2019; Rudolph et al., 2018). Therefore, since IL-6 is an important component of the cytokine storm in COVID-19, it might act as a crucial molecular mediator in the potential link between SARS-CoV-2 infection during pregnancy and neurodevelopmental and neuropsychiatric disorders in the offspring.
It is also important to consider that TNF-α and IL-17, in addition to other inflammatory mediators whose levels are commonly increased in COVID-19 patients (C-reactive protein, IL-1β, IL-2, IL-8, and interferons), may contribute to neurodevelopmental and neuropsychiatric diseases related to maternal immune activation (Jiang et al., 2018). Although the mechanisms by which cytokines affect brain development remain poorly understood, they seem to involve changes in functional brain connectivity, white matter damage, and abnormalities in neurotransmission (Jiang et al., 2018; Mirabella et al., 2021; Saunders et al., 2020). Cytokines from the periphery can also induce CNS responses in several ways, including diffusion across the disrupted BBB and induction of synthesis and release of cytokines by glial cells (Gu et al., 2021; Jiang et al., 2018). Therefore, even in the absence of vertical transmission or direct neuroinvasion by SARS-CoV-2, changes in the fetal and neonatal brain functions may occur due to immune activation.
In addition to environmental factors, susceptibility to neurodevelopmental and neuropsychiatric disorders may also involve molecular factors. Defects in glial genes related to synaptogenesis, synaptic transmission, and neuroinflammation have been reported in patients diagnosed with ASD (Scuderi and Verkhratsky, 2020). Supporting the role of altered glial-neuronal relationships during fetal CNS development in ASD, some genes that are essential for brain development and highly expressed in different glial cell types were also found to confer susceptibility to ASD (Scuderi and Verkhratsky, 2020). Notably, an analysis of differential gene expression datasets for clinical samples of COVID-19 patients identified a high number of genes that are associated with the pathophysiology of several neuropsychiatric disorders, including ASD, schizophrenia, bipolar disorder, depression, and Alzheimer’s disease (Quincozes-Santos et al., 2021a; Quincozes-Santos et al., 2021).
Glial cells are important players in neurodevelopment and maintenance of brain homeostasis, and disruption of their functions has been strongly associated with a wide range of neuropsychiatric disorders. The relationship between maternal infections and possible neurodevelopmental disorders and/or later neuropsychiatric consequences in the offspring has been established, in which the available evidence supports glial-related pathogenesis. Based on these relationships, SARS-CoV-2 infection either during pregnancy or during critical neurodevelopmental periods could be related to an increased risk of neurological consequences in offsprings later in life (Fig. 1); thus, efforts to unravel this hypothesis are currently needed. It is also possible to conceive expansion of the current TORCH/STORCHZ family, generating a new acronym to incorporate other emerging pathogens. Moreover, it is also important to consider the future and long-term public health concerns associated with SARS-CoV-2 infection during pregnancy, as well as a careful follow-up of infants born to COVID-19 positive mothers.
Figure 1: Putative role of glial cells in the possible neurodevelopmental and/or neuropsychiatric consequences related to SARS-CoV-2 infection during pregnancy. Recent evidence has raised the possibility of vertical transmission of SARS-CoV-2 as well as a direct brain infection. Additionally, maternal cytokine storm related to COVID-19 may cause fetal neuroinflammation. Both a direct infection and an inflammatory response triggered by SARS-CoV-2 might impact brain development by affecting critical functions of glial cells, as it has been demonstrated for other viral infections. These altered glial functions may increase the risk of later neurodevelopmental/neuropsychiatric disorders. The cells in the circle represent functional glial cells and neurons. Astrocytes are represented in blue; microglia are represented in yellow; SARS-CoV-2 is represented in green and cytokines/immune cells in pink.
Author Contribution: The authors confirm contribution to the paper as follows: LDB, LSM, FW, GTO, LS, WOBS, CAG, and AQS are responsible for conception, design, and draft manuscript preparation. All authors reviewed and approved the final version of the manuscript.
Ethics Approval: Not applicable.
Funding Statement: The authors are supported by Universidade Federal do Rio Grande do Sul (UFRGS), Conselho Nacional de Desenvolvimento Científico e Tecnológico (CNPq), Coordenação de Aperfeiçoamento de Pessoal de Nível Superior (CAPES), Fundação de Amparo à Pesquisa do Estado do Rio Grande do Sul (FAPERGS), and Instituto Nacional de Ciência e Tecnologia para Excitotoxicidade e Neuroproteção (INCTEN/CNPq).
Conflicts of Interest: The authors declare that they have no conflicts of interest to report regarding the present study.
References
Abdel-Mannan O, Eyre M, Löbel U, Bamford A, Eltze C, Hameed B, Hemingway C, Hacohen Y (2020). Neurologic and radiographic findings associated with COVID-19 infection in children. JAMA Neurology 77: 1440. DOI 10.1001/jamaneurol.2020.2687. [Google Scholar] [CrossRef]
Alexopoulou L, Holt AC, Medzhitov R, Flavell RA (2001). Recognition of double-stranded RNA and activation of NF-κB by Toll-like receptor 3. Nature 413: 732–738. DOI 10.1038/35099560. [Google Scholar] [CrossRef]
Ballas N, Lioy DT, Grunseich C, Mandel G (2009). Non-cell autonomous influence of MeCP2-deficient glia on neuronal dendritic morphology. Nature Neuroscience 12: 311–317. DOI 10.1038/nn.2275. [Google Scholar] [CrossRef]
Beys-da-Silva WO, da Rosa RL, Santi L, Tureta EF, Terraciano PB et al. (2020). The risk of COVID-19 for pregnant women: Evidences of molecular alterations associated with preeclampsia in SARS-CoV-2 infection. Biochimica et Biophysica Acta (BBA) Molecular Basis of Disease 1867: 165999. DOI 10.1016/j.bbadis.2020.165999. [Google Scholar] [CrossRef]
Bobermin LD, Quincozes-Santos A, Santos CL, Varela APM, Teixeira TF et al. (2020). Zika virus exposure affects neuron-glia communication in the hippocampal slices of adult rats. Scientific Reports 10: 21604. DOI 10.1038/s41598-020-78735-y. [Google Scholar] [CrossRef]
Bristot Silvestrin R, Bambini-Junior V, Galland F, Daniele Bobermim L, Quincozes-Santos A et al. (2013). Animal model of autism induced by prenatal exposure to valproate: Altered glutamate metabolism in the hippocampus. Brain Research 1495: 52–60. DOI 10.1016/j.brainres.2012.11.048. [Google Scholar] [CrossRef]
Cama VF, Marín-Prida J, Acosta-Rivero N, Acosta EF, Díaz LO et al. (2021). The microglial NLRP3 inflammasome is involved in human SARS-CoV-2 cerebral pathogenicity: A report of three post-mortem cases. Journal of Neuroimmunology 361: 577728. DOI 10.1016/j.jneuroim.2021.577728. [Google Scholar] [CrossRef]
Celewicz A, Celewicz M, Michalczyk M, Woźniakowska-Gondek P, Krejczy K, Misiek M, Rzepka R (2021). Pregnancy as a risk factor of severe COVID-19. Journal of Clinical Medicine 10: 5458. DOI 10.3390/jcm10225458. [Google Scholar] [CrossRef]
Colombo E, Farina C (2016). Astrocytes: Key regulators of neuroinflammation. Trends Immunology 37: 608–620. DOI 10.1016/j.it.2016.06.006. [Google Scholar] [CrossRef]
Colonna M, Butovsky O (2017). Microglia function in the central nervous system during health and neurodegeneration. Annual Review of Immunology 35: 441–468. DOI 10.1146/annurev-immunol-051116-052358. [Google Scholar] [CrossRef]
Coyne CB, Lazear HM (2016). Zika virus—reigniting the TORCH. Nature Reviews Microbiology 14: 707–715. DOI 10.1038/nrmicro.2016.125. [Google Scholar] [CrossRef]
Crunfli F, Carregari VC, Veras FP, Vendramini PH, Valença AGF et al. (2020). SARS-CoV-2 infects brain astrocytes of COVID-19 patients and impairs neuronal viability (preprint). Neurology. DOI 10.1101/2020.10.09.20207464. [Google Scholar] [CrossRef]
Dietz AG, Goldman SA, Nedergaard M (2020). Glial cells in schizophrenia: A unified hypothesis. The Lancet Psychiatry 7: 272–281. DOI 10.1016/S2215-0366(19)30302-5. [Google Scholar] [CrossRef]
Doyle MF (2021). Central nervous system outcomes of COVID-19. Translational Research 241: 41–51. DOI 10.1016/j.trsl.2021.09.002. [Google Scholar] [CrossRef]
Estes ML, McAllister AK (2016). Maternal immune activation: Implications for neuropsychiatric disorders. Science 353: 772–777. DOI 10.1126/science.aag3194. [Google Scholar] [CrossRef]
Fatemi SH, Folsom TD, Reutiman TJ, Sidwell RW (2008). Viral regulation of aquaporin 4, connexin 43, microcephalin and nucleolin. Schizophrenia Research 98: 163–177. DOI 10.1016/j.schres.2007.09.031. [Google Scholar] [CrossRef]
Favrais G, van de Looij Y, Fleiss B, Ramanantsoa N, Bonnin P et al. (2011). Systemic inflammation disrupts the developmental program of white matter. Annals of Neurology 70: 550–565. DOI 10.1002/ana.22489. [Google Scholar] [CrossRef]
Fenizia C, Biasin M, Cetin I, Vergani P, Mileto D et al. (2020). Analysis of SARS-CoV-2 vertical transmission during pregnancy. Nature Communications 11: 5128. DOI 10.1038/s41467-020-18933-4. [Google Scholar] [CrossRef]
Garden GA, Campbell BM (2016). Glial biomarkers in human central nervous system disease: Glial biomarkers in human CNS disease. Glia 64: 1755–1771. DOI 10.1002/glia.22998. [Google Scholar] [CrossRef]
Gilmore JH, Jarskog LF, Vadlamudi S (2005). Maternal poly I: C exposure during pregnancy regulates TNFα, BDNF, and NGF expression in neonatal brain and the maternal-fetal unit of the rat. Journal of Neuroimmunology 159: 106–112. DOI 10.1016/j.jneuroim.2004.10.008. [Google Scholar] [CrossRef]
Ginhoux F, Prinz M (2015). Origin of microglia: Current concepts and past controversies. Cold Spring Harbor Perspectives in Biology 7: a020537. DOI 10.1101/cshperspect.a020537. [Google Scholar] [CrossRef]
Gottfried C, Bambini-Junior V, Francis F, Riesgo R, Savino W (2015). The impact of neuroimmune alterations in autism spectrum disorder. Frontiers in Psychiatry 6: 121. DOI 10.3389/fpsyt.2015.00121. [Google Scholar] [CrossRef]
Greenhalgh AD, David S, Bennett FC (2020). Immune cell regulation of glia during CNS injury and disease. Nature Reviews Neuroscience 21: 139–152. DOI 10.1038/s41583-020-0263-9. [Google Scholar] [CrossRef]
Gu M, Mei XL, Zhao YN (2021). Sepsis and cerebral dysfunction: BBB damage, neuroinflammation, oxidative stress, apoptosis and autophagy as key mediators and the potential therapeutic approaches. Neurotoxicity Research 39: 489–503. DOI 10.1007/s12640-020-00270-5. [Google Scholar] [CrossRef]
Han RT, Kim RD, Molofsky AV, Liddelow SA (2021). Astrocyte-immune cell interactions in physiology and pathology. Immunity 54: 211–224. DOI 10.1016/j.immuni.2021.01.013. [Google Scholar] [CrossRef]
Hernández VS, Zetter MA, Guerra EC, Hernández-Araiza I, Karuzin N, Hernández-Pérez OR, Eiden LE, Zhang L (2021). ACE2 expression in rat brain: Implications for COVID-19 associated neurological manifestations. Experimental Neurology 345: 113837. DOI 10.1016/j.expneurol.2021.113837. [Google Scholar] [CrossRef]
Hosier H, Farhadian SF, Morotti RA, Deshmukh U, Lu-Culligan A et al. (2020). SARS-CoV-2 infection of the placenta. Journal of Clinical Investigation 130: 4947–4953. DOI 10.1172/JCI139569. [Google Scholar] [CrossRef]
Huang X, Hussain B, Chang J (2021). Peripheral inflammation and blood-brain barrier disruption: Effects and mechanisms. CNS Neuroscience & Therapeutics 27: 36–47. DOI 10.1111/cns.13569. [Google Scholar] [CrossRef]
Jiang NM, Cowan M, Moonah SN, Petri WA (2018). The impact of systemic inflammation on neurodevelopment. Trends in Molecular Medicine 24: 794–804. DOI 10.1016/j.molmed.2018.06.008. [Google Scholar] [CrossRef]
Joma M, Fovet CM, Seddiki N, Gressens P, Laforge M (2021). COVID-19 and pregnancy: Vertical transmission and inflammation impact on newborns. Vaccines 95-Molecular Approaches to the Control of Infectious Diseases 9: 391. DOI 10.3390/vaccines9040391. [Google Scholar] [CrossRef]
Karasek D, Baer RJ, McLemore MR, Bell AJ, Blebu BE et al. (2021). The association of COVID-19 infection in pregnancy with preterm birth: A retrospective cohort study in California. The Lancet Regional Health–Americas 2: 100027. DOI 10.1016/j.lana.2021.100027. [Google Scholar] [CrossRef]
Kinney JS, Kumar ML (1988). Should we expand the TORCH complex? A description of clinical and diagnostic aspects of selected old and new agents. Clinics in Perinatology 15: 727–744. DOI 10.1016/S0095-5108(18)30670-5. [Google Scholar] [CrossRef]
Knuesel I, Chicha L, Britschgi M, Schobel SA, Bodmer M, Hellings JA, Toovey S, Prinssen EP (2014). Maternal immune activation and abnormal brain development across CNS disorders. Nature Reviews Neurology 10: 643–660. DOI 10.1038/nrneurol.2014.187. [Google Scholar] [CrossRef]
Li K, Wohlford-Lenane C, Perlman S, Zhao J, Jewell AK, Reznikov LR, Gibson-Corley KN, Meyerholz DK, McCray PB (2016). Middle east respiratory syndrome coronavirus causes multiple organ damage and lethal disease in mice transgenic for human dipeptidyl peptidase 4. The Journal of Infectious Diseases 213: 712–722. DOI 10.1093/infdis/jiv499. [Google Scholar] [CrossRef]
Li M, Chen L, Zhang J, Xiong C, Li X (2020). The SARS-CoV-2 receptor ACE2 expression of maternal-fetal interface and fetal organs by single-cell transcriptome study. PLoS One 15: e0230295. DOI 10.1371/journal.pone.0230295. [Google Scholar] [CrossRef]
Liddelow SA, Marsh SE, Stevens B (2020). Microglia and astrocytes in disease: Dynamic duo or partners in crime? Trends in Immunology 41: 820–835. DOI 10.1016/j.it.2020.07.006. [Google Scholar] [CrossRef]
Limonta D, Jovel J, Kumar A, Airo A, Hou S et al. (2018). Human fetal astrocytes infected with zika virus exhibit delayed apoptosis and resistance to interferon: Implications for persistence. Viruses 10: 646. DOI 10.3390/v10110646. [Google Scholar] [CrossRef]
Lye P, Dunk CE, Zhang J, Wei Y, Nakpu J et al. (2020). SARS-CoV-2 cell entry gene ACE2 expression in immune cells that infiltrate the placenta in infection-associated preterm birth (preprint). Obstetrics and Gynecology. DOI 10.1101/2020.09.27.20201590. [Google Scholar] [CrossRef]
Maezawa I, Jin LW (2010). Rett syndrome microglia damage dendrites and synapses by the elevated release of glutamate. Journal of Neuroscience 30: 5346–5356. DOI 10.1523/JNEUROSCI.5966-09.2010. [Google Scholar] [CrossRef]
Matschke J, Lütgehetmann M, Hagel C, Sperhake JP, Schröder AS et al. (2020). Neuropathology of patients with COVID-19 in Germany: A post-mortem case series. The Lancet Neurology 19: 919–929. DOI 10.1016/S1474-4422(20)30308-2. [Google Scholar] [CrossRef]
Megli CJ, Coyne CB (2021). Infections at the maternal-fetal interface: An overview of pathogenesis and defence. Nature Reviews Microbiology 20: 67–82. DOI 10.1038/s41579-021-00610-y. [Google Scholar] [CrossRef]
Mirabella F, Desiato G, Mancinelli S, Fossati G, Rasile M et al. (2021). Prenatal interleukin 6 elevation increases glutamatergic synapse density and disrupts hippocampal connectivity in offspring. Immunity 54: 2611–2631.e8. DOI 10.1016/j.immuni.2021.10.006. [Google Scholar] [CrossRef]
Moriguchi T, Harii N, Goto J, Harada D, Sugawara H et al. (2020). A first case of meningitis/encephalitis associated with SARS-Coronavirus-2. International Journal of Infectious Diseases 94: 55–58. DOI 10.1016/j.ijid.2020.03.062. [Google Scholar] [CrossRef]
Nayak AH, Kapote DS, Fonseca M, Chavan N, Mayekar R, Sarmalkar M, Bawa A (2020). Impact of the Coronavirus infection in pregnancy: A preliminary study of 141 patients. The Journal of Obstetrics and Gynecology of India 70: 256–261. DOI 10.1007/s13224-020-01335-3. [Google Scholar] [CrossRef]
Neu N, Duchon J, Zachariah P (2015). TORCH infections. Clinics in Perinatology 42: 77–103. DOI 10.1016/j.clp.2014.11.001. [Google Scholar] [CrossRef]
Odeberg J, Wolmer N, Falci S, Westgren M, Sundtröm E, Seiger Å., Söderberg-Nauclér C (2007). Late human cytomegalovirus (HCMV) proteins inhibit differentiation of human neural precursor cells into astrocytes. Journal of Neuroscience Research 85: 583–593. DOI 10.1002/(ISSN)1097-4547. [Google Scholar] [CrossRef]
Pantelis C, Jayaram M, Hannan AJ, Wesselingh R, Nithianantharajah J et al. (2020). Neurological, neuropsychiatric and neurodevelopmental complications of COVID-19. Australian & New Zealand Journal of Psychiatry 55: 750–762. DOI 10.1177/0004867420961472. [Google Scholar] [CrossRef]
Quincozes-Santos A, da Rosa RL, Bobermin LD, Tureta EF, Santi L et al. (2021). Association between molecular markers of COVID-19 and Alzheimer’s disease. Journal of Medical Virology, DOI 10.1002/jmv.27391. [Google Scholar] [CrossRef]
Quincozes-Santos A, Rosa RL, Tureta EF, Bobermin LD, Berger M, Guimarães JA, Santi L, Beys-da-Silva WO (2021a). COVID-19 impacts the expression of molecular markers associated with neuropsychiatric disorders. Brain, Behavior, & Immunity-Health 11: 100196. DOI 10.1016/j.bbih.2020.100196. [Google Scholar] [CrossRef]
Quincozes-Santos A, Santos CL, de Souza Almeida RR, da Silva A, Thomaz NK et al. (2021b). Gliotoxicity and glioprotection: The dual role of glial cells. Molecular Neurobiology 58: 6577–6592. DOI 10.1007/s12035-021-02574-9. [Google Scholar] [CrossRef]
Rasmussen JM, Graham AM, Entringer S, Gilmore JH, Styner M, Fair DA, Wadhwa PD, Buss C (2019). Maternal interleukin-6 concentration during pregnancy is associated with variation in frontolimbic white matter and cognitive development in early life. NeuroImage 185: 825–835. DOI 10.1016/j.neuroimage.2018.04.020. [Google Scholar] [CrossRef]
Reyes-Lagos JJ, Abarca-Castro EA, Echeverría JC, Mendieta-Zerón H, Vargas-Caraveo A, Pacheco-López G (2021). A translational perspective of maternal immune activation by SARS-CoV-2 on the potential prenatal origin of neurodevelopmental disorders: The role of the cholinergic anti-inflammatory pathway. Frontiers in Psychology 12: 614451. DOI 10.3389/fpsyg.2021.614451. [Google Scholar] [CrossRef]
Rudolph MD, Graham AM, Feczko E, Miranda-Dominguez O, Rasmussen JM, Nardos R, Entringer S, Wadhwa PD, Buss C, Fair DA (2018). Maternal IL-6 during pregnancy can be estimated from newborn brain connectivity and predicts future working memory in offspring. Nature Neuroscience 21: 765–772. DOI 10.1038/s41593-018-0128-y. [Google Scholar] [CrossRef]
Santi L, Riesgo RS, Quincozes-Santos A, Schuler-Faccini L, Tureta EF et al. (2021). Zika virus infection associated with autism spectrum disorder: A case report. Neuroimmunomodulation 28: 229–232. DOI 10.1159/000516560. [Google Scholar] [CrossRef]
Saunders JM, Moreno JL, Ibi D, Sikaroodi M, Kang DJ et al. (2020). Gut microbiota manipulation during the prepubertal period shapes behavioral abnormalities in a mouse neurodevelopmental disorder model. Scientific Reports 10: 4697. DOI 10.1038/s41598-020-61635-6. [Google Scholar] [CrossRef]
Schoenmakers S, Snijder P, Verdijk RM, Kuiken T, Kamphuis SSM et al. (2021). Severe acute respiratory syndrome Coronavirus 2 placental infection and inflammation leading to fetal distress and neonatal multi-organ failure in an asymptomatic woman. Journal of the Pediatric Infectious Diseases Society 10: 556–561. DOI 10.1093/jpids/piaa153. [Google Scholar] [CrossRef]
Scuderi C, Verkhratsky A (2020). The role of neuroglia in autism spectrum disorders. Progress in Molecular Biology and Translational Science 173: 301–330. DOI 10.1016/bs.pmbts.2020.04.011. [Google Scholar] [CrossRef]
Smith SEP, Li J, Garbett K, Mirnics K, Patterson PH (2007). Maternal immune activation alters fetal brain development through interleukin-6. Journal of Neuroscience 27: 10695–10702. DOI 10.1523/JNEUROSCI.2178-07.2007. [Google Scholar] [CrossRef]
Solomon T (2021). Neurological infection with SARS-CoV-2-the story so far. Nature Reviews Neurology 17: 65–66. DOI 10.1038/s41582-020-00453-w. [Google Scholar] [CrossRef]
Song E, Zhang C, Israelow B, Lu-Culligan A, Prado AV et al. (2021). Neuroinvasion of SARS-CoV-2 in human and mouse brain. Journal of Experimental Medicine 218: e20202135. DOI 10.1084/jem.20202135. [Google Scholar] [CrossRef]
Tay TL, Savage JC, Hui CW, Bisht K, Tremblay MÈ (2017). Microglia across the lifespan: From origin to function in brain development, plasticity and cognition: Microglia across the lifespan. The Journal of Physiology 595: 1929–1945. DOI 10.1113/JP272134. [Google Scholar] [CrossRef]
Tremblay ME, Madore C, Bordeleau M, Tian L, Verkhratsky A (2020). Neuropathobiology of COVID-19: The role for glia. Frontiers in Cellular Neuroscience 14: 592214. DOI 10.3389/fncel.2020.592214. [Google Scholar] [CrossRef]
Vianna P, do Gomes JA, Boquett JA, Fraga LR, Schuch JB, Vianna FSL, Schuler-Faccini L (2018). Zika virus as a possible risk factor for autism spectrum disorder: Neuroimmunological aspects. Neuroimmunomodulation 25: 320–327. DOI 10.1159/000495660. [Google Scholar] [CrossRef]
Vivanti AJ, Vauloup-Fellous C, Prevot S, Zupan V, Suffee C, Do Cao J, Benachi A, de Luca D (2020). Transplacental transmission of SARS-CoV-2 infection. Nature Communications 11: 3572. DOI 10.1038/s41467-020-17436-6. [Google Scholar] [CrossRef]
Wu WL, Hsiao EY, Yan Z, Mazmanian SK, Patterson PH (2017). The placental interleukin-6 signaling controls fetal brain development and behavior. Brain, Behavior, and Immunity 62: 11–23. DOI 10.1016/j.bbi.2016.11.007. [Google Scholar] [CrossRef]
Wu YT, Liu J, Xu JJ, Chen YF, Yang W et al. (2020). Neonatal outcome in 29 pregnant women with COVID-19: A retrospective study in Wuhan, China. PLoS Medicine 17: e1003195. DOI 10.1371/journal.pmed.1003195. [Google Scholar] [CrossRef]
Xiao T, Xia S, Zeng L, Lin G, Wei Q et al. (2020). A multicentre observational study on neonates exposed to SARS-CoV-2 in China: The Neo-SARS-CoV-2 study protocol. BMJ Open 10: e038004. DOI 10.1136/bmjopen-2020-038004. [Google Scholar] [CrossRef]
Zhu H, Wang L, Fang C, Peng S, Zhang L, Chang G, Xia S, Zhou W (2020). Clinical analysis of 10 neonates born to mothers with 2019-nCoV pneumonia. Translational Pediatrics 9: 51–60. DOI 10.21037/tp.2020.02.06. [Google Scholar] [CrossRef]
Ziats MN, Edmonson C, Rennert OM (2015). The autistic brain in the context of normal neurodevelopment. Frontiers in Neuroanatomy 9: 115. DOI 10.3389/fnana.2015.00115. [Google Scholar] [CrossRef]
Zimmer A, Youngblood A, Adnane A, Miller BJ, Goldsmith DR (2021). Prenatal exposure to viral infection and neuropsychiatric disorders in offspring: A review of the literature and recommendations for the COVID-19 pandemic. Brain, Behavior, and Immunity 91: 756–770. DOI 10.1016/j.bbi.2020.10.024. [Google Scholar] [CrossRef]
Zuchero JB, Barres BA (2015). Glia in mammalian development and disease. Development 142: 3805–3809. DOI 10.1242/dev.129304. [Google Scholar] [CrossRef]
Cite This Article
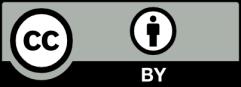
This work is licensed under a Creative Commons Attribution 4.0 International License , which permits unrestricted use, distribution, and reproduction in any medium, provided the original work is properly cited.